Fig. 14.1
SNP array-based single cell copy number analysis plots using three different WGA methodologies. The Sigma Aldrich GenomePlex WGA4 provided the most accurate copy number assignments when compared to Rubicon Genomics’ PicoPlex (also repackaged as SurePlex by Illumina) or Yikon Genomics’ MALBAC WGA kit. Both false-negative and false-positive imbalances were detected by the latter two WGA methods
SNP Array-Based CCS
There are many array-based methods available to interrogate WGA DNA for CCS, including array CGH with BAC arrays or oligonucleotide arrays and various SNP arrays from a number of manufacturers. As array CGH is discussed at length elsewhere in this book, here we will focus on SNP array technologies.
There are two major ways in which SNP arrays have been utilized to interrogate aneuploidy in the human embryo. One involves the use of genotyping data and inheritance patterns to indirectly predict chromosome copy number [19, 20]. Mendelian inheritance rules of bialleleic SNPs and linkage within haplotype blocks are important to the success of these methods. For example, when each parent possesses homozygosity for opposite alleles (i.e., mother is AA and father is BB), all normal embryos will inherit an AB genotype. When the observed embryonic genotype differs from AB, it is an indicator that aneuploidy exists. For example, if the embryo was found to have a BB genotype, it would indicate that only the paternal chromosome was inherited and that a monosomy of maternal origin was present. Additional criteria such as crossover frequency and position and probabilities of inheriting blocks of SNPs from each parent based upon published frequencies from the HapMap project also impact the predictions of aneuploidy in some instances. At least two groups have developed this type of approach which appears to be better suited for MDA-based WGA instead of PCR based, particularly since MDA provides better genotyping accuracy.
Another way that SNP array data can be used to evaluate aneuploidy is through direct analysis of signal intensities. That is, at a given position (SNP loci), if the signal intensities are higher in an embryo than in a known normal sample it would indicate a trisomy state, or if the signal were lower, a monosomy state. A number of groups have utilized this strategy, most of which involve the use of PCR-based WGA, which gives better copy number accuracy than MDA. Accuracy of evaluating single cell aneuploidy in at least one case was established using cell lines already known to possess specific abnormalities. This study demonstrated a 99 % level of accuracy and represented an important preclinical validation step [18].
One advantage of using SNP arrays (compared to array CGH) is the availability of information from genotypes. For example, the ability to track which embryo implants after multiple-embryo transfer using SNP-based DNA fingerprinting has proven useful in a number of clinical trials, including studies on the clinical impact of embryo biopsy [13] and oocyte vitrification [15]. Other applications include determining the origin of aneuploidy [21], cell division origin of polar bodies [22], the ability to detect uniparental disomy [23], and confirmation of success of somatic cell nuclear transfer in stem cell research [24, 25].
SNP arrays have also been extensively evaluated for clinical utility. For example, outcomes following trophectoderm biopsy and blastocyst vitrification included a live birth rate of 71 % among patients where mean maternal age was 37.8 years [2]. A prospective, blinded, non-selection study demonstrated a 96 % predictive value of an aneuploidy diagnosis for a negative clinical outcome and that trophectoderm-based CCS had significantly better positive predictive value than did blastomere biopsy-based CCS [14]. Finally, an ongoing randomized controlled trial has demonstrated significantly improved success rates with the incorporation of SNP array CCS and blastocyst vitrification compared to fresh transfer of unscreened blastocysts [26].
SNP arrays have also been used to evaluate translocations in embryo from carrier patients [27–31]. This involves interrogating subchromosomal imbalances on each side of the breakpoints from cases with reciprocal translocations. Studies have demonstrated resolution to segments as small as 2.3 Mb. However, the ability to detect de novo segmental imbalances remains a matter of debate as many findings may represent artifacts of the methodology.
qPCR-Based CCS
While the use of SNP arrays was shown to improve outcomes compared to traditional morphology-based embryo selection, the per-embryo cost of WGA and SNP array chips is significant. Due to the processing time required for SNP array, trophectoderm biopsy and fresh embryo transfer within the same cycle would not be feasible within the window of time that the endometrium was receptive. These factors provided the impetus to develop more rapid methods of CCS. qPCR is faster and less expensive than all array-based methods of CCS which represents the driving force behind its development [32–34]. One of the ways in which costs are reduced is through avoiding the need for WGA. Instead, the embryonic DNA is amplified by multiplex PCR of specific targets in the genome, four per chromosome. Each target is then individually quantified in a subsequent qPCR reaction on a 384-well plate. Relative quantities of each target are determined through comparison to a known normal sample. Because the method only requires two PCR steps, it is extremely easy to perform in the laboratory, requires very little specialized equipment, and can be completed within 4 h of embryo biopsy. In addition, because the initial amplification uses conventional PCR, additional primers can be added which work under the same conditions and allow for specific targets such as single gene disorder loci to be interrogated in parallel [35].
Preclinical experiments have demonstrated 99 % accuracy of predicting 24-chromosome aneuploidy using qPCR [34]. In addition to demonstrating equivalence to G-banding in cell lines and SNP array testing in embryos, two randomized controlled trials showed significant improvements in the success of IVF when incorporating qPCR-based CCS [10, 11]. The first of these studies demonstrated a 17.2 % increase in delivery rates with the use of qPCR CCS compared to controls. The second study demonstrated equivalent success rates and no twins when a single qPCR euploid blastocyst was transferred compared to a control group that received two unscreened embryo transfers, having a twin rate of 53 %. Together, these studies appear to establish that elective single qPCR euploid blastocyst transfer is an effective methodology and follow-up studies indicate improved neonatal outcomes [36].
Next-Generation Sequencing-Based CCS
Similar to the driving force behind qPCR CCS development, next-generation sequencing (NGS) provides an even greater opportunity to reduce testing costs. The main premise of NGS-based CCS is the ability to produce massively parallel sequencing, which can then be computationally aligned to the published human genome sequence. The number of sequence reads which align to each specific chromosome can be counted and then normalized to a known normal sample so that each chromosome can be quantified. The ability to add molecular barcodes to each embryo allows for many embryos to be sequenced in the same reaction. The molecular barcode is essentially a specific unique sequence of bases that can be artificially synthesized and added to the ends of the PCR products of each embryo. The labeled PCR products can then be pooled together for sequencing and then computationally segregated back according to which embryo it came from.
Some groups have begun to develop NGS for CCS using whole genome amplification (WGA) which may allow for whole genome sequencing (WGS) depending on the number of bases sequenced for each sample [37, 38]. However, counting chromosomes requires far less sequence per sample to accomplish than does WGS. Sequencing at low coverage and depth also helps avoid the potential for incidental findings, while maintaining an appropriate cost per sample [39].
Another approach to NGS-based CCS involves targeted multiplex PCR in place of WGA [38]. Similar to qPCR, a subset of the genome with distribution of targets on each chromosome can be amplified by PCR. This further enhances the cost reduction benefit of NGS since PCR is much less expensive than any commercially available WGA methodology. Another advantage is the ability to add primers for specific single gene disorder targets for simultaneous PGD and CCS [35].
Mosaicism
Mosaicism represents an important challenge to the success of CCS. Currently there is no CCS method that provides an opportunity to diagnose mosaicism in a preimplantation embryo. This is due to the unavoidable sampling error associated with CCS, since a representative biopsy is used to make a diagnosis. For example, in the event that a mosaic embryo is biopsied and the biopsied portion of the embryo is aneuploid, that embryo will be diagnosed as aneuploid, when in fact there may be euploid cells in the remaining embryo. In addition, if the biopsied portion is euploid, the embryo will be diagnosed as euploid despite the fact there may be aneuploid cells in the remaining embryo. In both cases, an accurate diagnosis would have been mosaic.
Since mosaicism cannot be accurately diagnosed, it is important to consider what the prevalence might be. However, prior studies have been limited either by a lack of comprehensive analysis of all chromosomes (i.e., FISH), sample selection bias (i.e., abnormal or discarded embryos), or the use of methodologies of CCS having unproven diagnostic consistency. For example, SNP array-based analysis of blastocysts indicated a mosaicism rate of 24 %, but this was derived from the analysis of 50 embryos that had been given an aneuploid diagnosis by cleavage stage FISH [40]. Therefore, these data may not reflect the rate of mosaicism present in the larger population of embryos from patients seeking CCS due to selection bias. Another set of data that might be used to infer the prevalence of mosaicism comes from the evaluation of the origin of aneuploidy. That is, aneuploidy derived from postzygotic mitotic errors would by definition result in a mosaic embryo. For example, data from sequential analysis of polar bodies and the embryo indicated a non-maternal meiotic origin of aneuploidy rate of 33 % [21]. However, since the contribution of paternal meiotic aneuploidy could not be determined, a more precise estimate of the frequency of mitotic errors was not possible.
Another important consideration when performing CCS at the blastocyst stage is sensitivity to detection of aneuploidy within a mosaic trophectoderm biopsy. Previous data suggest that the sensitivity of array CGH is approximately 50 % [40], while SNP arrays are 40 % [41]. That is, when 40–50 % of the cells within a biopsy have the same aneuploidy, these methods may be able detect the aneuploidy successfully. Some authors have proposed that when an embryo has greater than 50 % euploid cells, then a euploid diagnosis is accurate, and that when an embryo is more than 50 % aneuploid, an aneuploid diagnosis is accurate [42]. However, this declaration on ploidy is based on arbitrary thresholds unsupported by any objective, scientific evidence.
With all of these factors in mind, it becomes important to consider how often mosaicism might lead to misdiagnosis. As discussed previously, one method to evaluate the predictive value of CCS is through a non-selection study where embryos are biopsied, but the CCS data are not used to select the embryos. This study allows one to evaluate whether CCS would have correctly predicted the actual clinical outcome. In the only such study, SNP arrays demonstrated a 96 % negative predictive value, indicating that 4 % of embryos diagnosed as aneuploid still possessed euploid cells sufficient to develop into a chromosomally normal child [14]. In this study, there were no false negatives (i.e., diagnosis of euploidy with subsequent implantation of an aneuploid gestation). However, the sample size to evaluate false negatives may not have been sufficient since most aneuploidies are incompatible with implantation or development into a clinically recognized pregnancy. Instead, a retrospective analysis of a large number of euploid embryo transfers can be performed. Indeed, such a study has indicated a clinical error rate of 0.21 % in 4,794 euploid embryo transfers, with all follow-up analyses of products of conception indicating the presence of mosaicism (unpublished observations).
Discussion
Considerable progress has been made over the last several years to establish evidence that supports the use of CCS to select embryos and improve the success of IVF. Additional factors such as the costs associated with the procedure and the applicable patient populations represent the current focus of ongoing research and development. Solutions to the problems associated with mosaicism may present themselves in the form of new noninvasive methodologies such as time-lapse morphokinetics. In addition, future work to develop additional biomarkers of reproductive competence of the human embryo stands to benefit significantly from the incorporation of aneuploidy screening in study designs. Specifically, it will be important to control for aneuploidy when evaluating the predictive value of new biomarkers since it is clearly one of the most important restraints on reproductive potential. Finally, as we continue to benefit from the advances made in assessing and understanding the human genome sequence and move towards the era of personalized medicine, future studies to identify the genetics of aneuploidy risk and prevention represent an exciting opportunity to continue to improve the treatment of infertility.
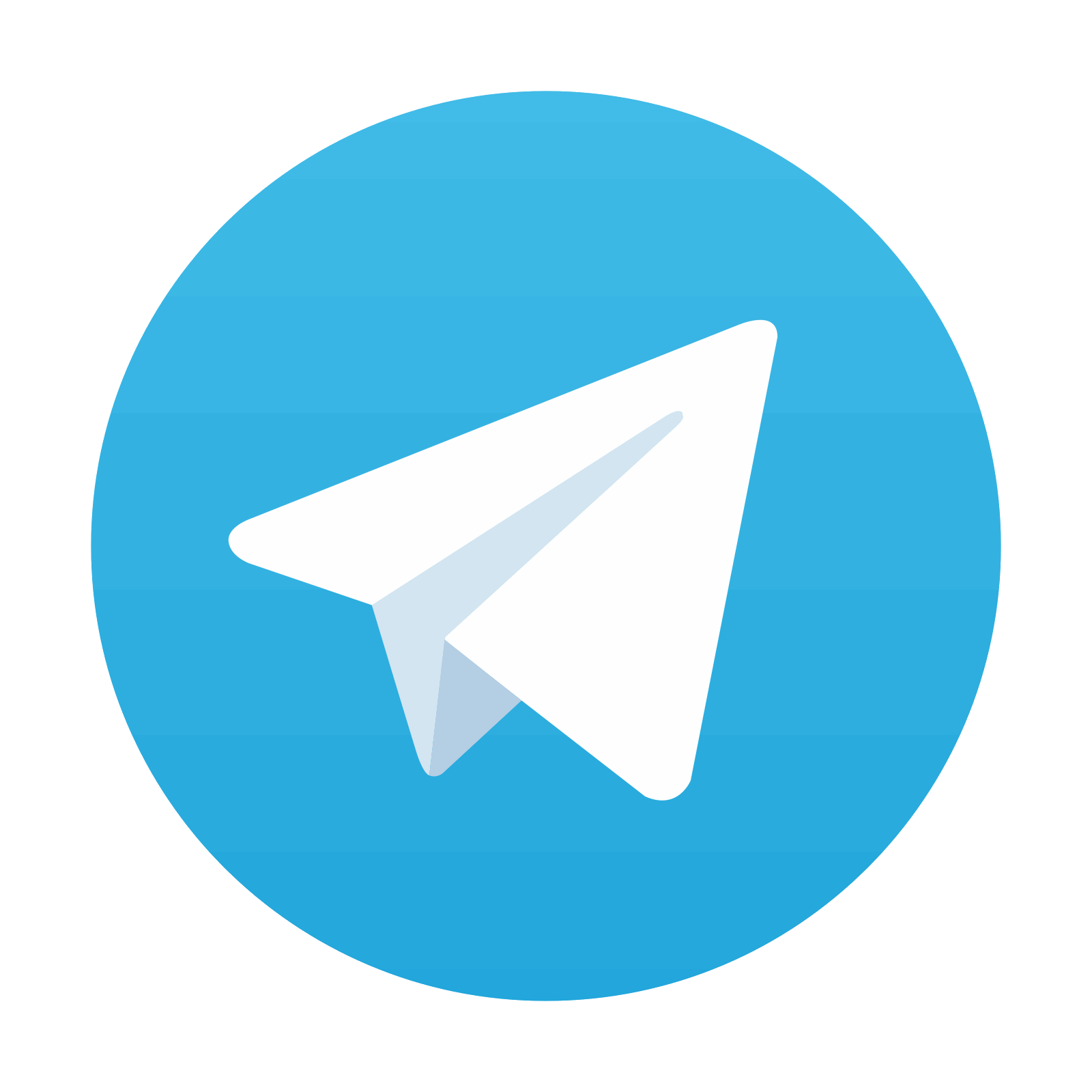
Stay updated, free articles. Join our Telegram channel

Full access? Get Clinical Tree
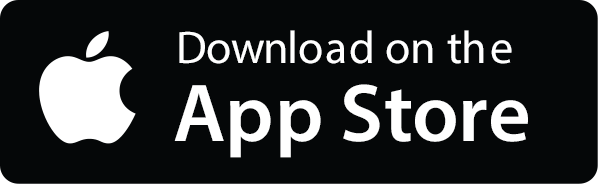
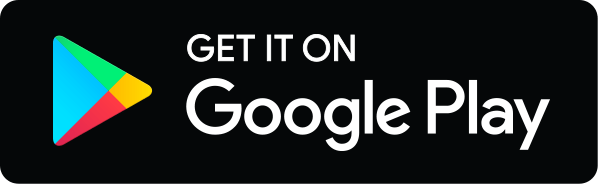