Figure 6.2 Chart® record of antagonism of acetylcholine by d-tubocurarine in the presence of 1 μM serine in leech isolated dorsal muscle. The bath volume was 25 mL, and the abscissa is time (min.).
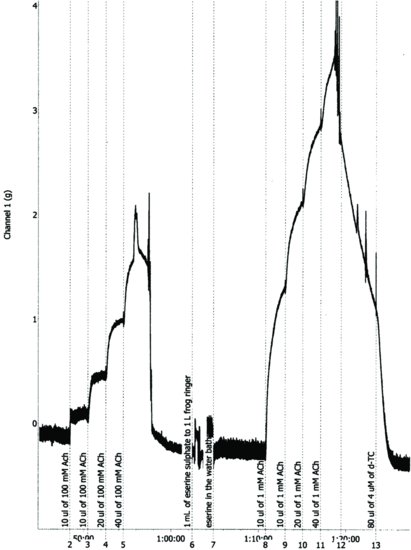
Results
Figure 6.3 Concentration–response curves for acetylcholine (a), and nicotine (b), in the absence and presence of three concentrations of d-tubocurarine (d-TC) in the leech isolated dorsal muscle.
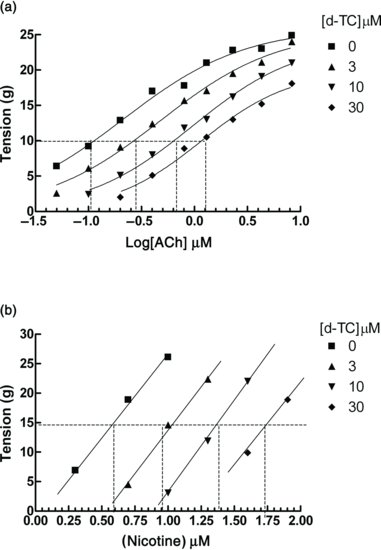
Figure 6.4 Schild plot for the antagonism of responses to acetylcholine and nicotine by d-tubocurarine (d-TC) in the leech isolated dorsal muscle. Note that very similar pA2 values (pA2 = 5.75, slope = −1.2 against acetylcholine, and pA2 = 5.64, slope = −1.01 against nicotine) are found for the antagonist using both agonists, indicating that acetylcholine and nicotine are both acting at the same receptor.
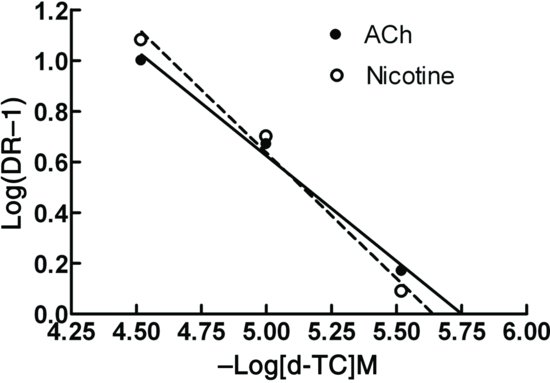
Questions
6.3 FOCALLY INNERVATED SKELETAL MUSCLE PREPARATIONS
It is challenging to recommend a focally innervated muscle preparation that is suitable to be carried out by students in a class practical. Not only do these preparations require a high animal usage (one animal provides no more than two preparations), but also they are technically demanding in that they require good dissection skills and the attachment of a nerve to an electrode. The preparation that is probably most suitable and robust for students is the frog gastrocnemius muscle and sciatic nerve preparation. The nerve is easy to dissect, and the muscle is large and shows a large force development. However, as mentioned in Section 6.2, the access to frogs is restricted since there has been a large decline in frog populations worldwide. Furthermore, it is desirable to minimize the use of vertebrates for student education. Nevertheless, it is important to understand the pharmacology of focally innervated muscles since it is therapeutically important. This is one area where the use of computer aided learning programmes is especially useful. It may be useful to list the types of preparations that have been used in research, and describe the protocol for one in detail. As examples of in vitro preparations, the frog gastrocnemius–sciatic nerve, the rat phrenic nerve hemidiaphragm and the innervated biventer cervicis preparation of the chick were particularly widely used. Many years ago, in vivo experiments in anaesthetized animals were used to gain much of our understanding about the action of intravenously administered drugs on electrically stimulated muscle preparations. The results of such experiments provided the pharmacological basis for many of the drugs routinely used therapeutically today. Examples of such uses are in surgical operations done under anaesthesia, orthopaedic treatments such as knee and hip replacements, and to control convulsions due to electroconvulsive therapy or infection with Clostridium tetani (tetanus).
Compared with multiply-innervated muscles, this type of muscle responds very differently to agonists acting at the nicotinic cholinoceptor and depolarizing and non-depolarizing blockers. In order to observe the effects of drugs on these preparations it is necessary to electrically stimulate the nerve supplying the muscle. Because of the difference in the way tension development is controlled, it is necessary to stimulate using a square-wave pulse generator (Section 3.7) in order to study the pharmacology of focally innervated skeletal muscle. Unlike multiply-innervated muscles, there is only one junction of a motor nerve ending on each muscle cell (neuromuscular junction). Here there is a specialized region where there is a very tight junction between the nerve ending and muscle membrane where there are deep invaginations in the outer muscle cell membrane. The nicotinic cholinoceptors are located in this region. When an action potential arrives at the neuromuscular junction there is release of acetylcholine; it activates post-synaptic nicotinic receptors which result in a small depolarization of the muscle cell, termed miniature end-plate potentials (MEPPs). These are too small to be propagated throughout the fibril. However, if action potentials arrive at the nerve terminal with sufficient frequency, there is an additive effect of the MEPPs and the local end-plate potential depolarization is sufficient to reach the threshold depolarization at about −65 mV. At this voltage, an action potential is generated which is then propagated to the muscle extremities. The resultant wave of depolarization travels to the T-tubule system, where Ca2+ is released from sarcoplasmic reticulum storage sites resulting in actin–myosin interaction and the transitory development of tension, termed a twitch. A muscle twitch is defined as a single contraction of a muscle tissue resulting from a single stimulus or a single compound neural action potential. The contraction of a single fibre will give an all-or-nothing transitory twitch, which rapidly returns to the baseline. The amplitude of the twitch increases as the frequency of pulses or pulses increases. This is due to the recruitment or triggering of an increasing number of muscle fibres. At higher frequencies twitches will merge and fail to return to baseline between twitches, and twitches begin to merge. Eventually a frequency is reached where merged twitches reach a maximum. This is termed as tetanus. After a tetanus is established and allowed to return to baseline for 1 min, when stimulation is started at a lower frequency, a supra-normal size of twitch is obtained, termed post-tetanic potentiation. This is thought to be due to a large increase in intracellular [Ca2+] during tetanus, which does not reach baseline during a short rest period. Post-tetanic potentiation rapidly fades over a 10 min period.
To set up a focally innervated muscle preparation, it is first necessary to establish the stimulation parameters. When a sub-maximal voltage and frequency have been established, the actions of drugs acting at the neuromuscular junction can be explored. A large number of drugs act pre-junctionally on the cholinergic nerve endings. This includes drugs interfering with the synthesis, storage and release of acetylcholine. In addition, the pre-junctional nerve is the target of surprisingly large array of venoms and toxins (Bowman and Rand, 1980). The most important of the drugs which act post-junctionally, at sites on the nicotinic cholinoceptor, are depolarizing and non-depolarizing antagonists. Non-depolarizing antagonists compete reversibly for the acetylcholine binding site on the nicotinic receptor. As in multiply-innervated muscle preparations, the block is removed by the application of acetylcholine inhibitors, which cause an increase in the synaptic concentration of acetylcholine. The effect of depolarizing antagonists differs from those on multiply-innervated muscles (Section 6.1). Whereas succinylcholine (suxamethonium) causes the development of a slow contracture in multiply-innervated muscles, in focally innervated muscles there is a short-lasting increase in electrically generated twitches, known as ‘fasciculation’, which is followed by a block. This block is not reversed by acetylcholinesterase inhibitors, or by any other known drug. This is one disadvantage to the clinical use of depolarizing blockers.
6.3.1 The Frog Gastrocnemius Muscle–Sciatic Nerve Preparation
Experimental Conditions
Organ bath | 20 mL, or larger |
Ringer solution | Frog Ringer |
Aeration | Air |
Temperature | 20°C |
Transducer | Isometric |
Dose cycle | 10–15 min |
Stimulus parameters | Amplitude 0.2–10 V |
Pulse width 0.2 ms | |
Frequency | 0.1–15 Hz |
Procedure
Remove the skin from the whole length of the leg. Place the leg in a deep petri dish containing frog Ringer solution. Carefully tease apart the muscle and locate the thick, myelinated sciatic nerve. This is silvery white in appearance. Gently tease out the nerve but be careful not to use any sharp instruments to make sure it stays intact. It is useful to place a narrow glass rod under the rod. Remove the femur. Locate the large gastrocnemius muscle in the lower part of the leg. Note the attachments to the tibia below the knee and the Achilles tendon. Free the muscle from surrounding tissue and tibia, but leave the attachments at the knee and Achilles tendon. A cotton thread is placed around the attachment to the Achilles tendon. This is tied to a metal support. Another thread is placed around the remaining knee tissue and upper muscle attachment and kept free for later attachment to the transducer. The sciatic nerve is carefully fastened to the metal electrode. The whole nerve and muscle structure is placed in the organ bath containing frog Ringer solution, and the free thread from the upper muscle–knee attached to the transducer.
Electrical Stimulation
The maximum amplitude (voltage) and frequency are first established. Begin at a frequency of 0.1 Hz and a voltage of 0.2 V. Stimulate for 3 s intervals, and double the voltage for each interval until a maximum size of twitch is reached. Using this maximum frequency, repeat this procedure of stimulation for 3 s intervals, but this time double the frequency of stimulation. As the frequency increases the twitches will start to fail to return to baseline, and eventually completely merge and reach a maximum size. This is when tetanus has been reached. Rest the tissue for 1 min, and begin to stimulate again at a lower frequency. Large twitches will be seen and that soon fade to a normal height. A graph of the log frequency–response should show a sigmoid relationship.
The actions of blockers of the nicotinic cholinergic receptor are now tested. Select a sub-maximal frequency and stimulate for 3 s. Add 2.5 μM suxamethonium to the bath and wait for 5 min. Stimulate for 3 s intervals, then wash out. When the control twitch height is restored, add 1 μM d-TC and wait 5 min before stimulating for 3 s intervals again. Wash out and add 10 μM eserine, and wait 5 min. Add 2.5 μM suxamethonium, and repeat the electrical stimulation for 3 s intervals. There should be no effect on twitch height. Repeat this procedure with 1 μM d-TC in the presence of eserine. The twitches should be seen to be clearly blocked. After washing out, if 10 μM acetylcholine is added in the presence of serine, the twitches should also be seen to be clearly decreased due to depolarization block.
REFERENCES
Dale, H.H. and Feldberg, W. (1934) Chemical transmission of secretory impulses to the sweat glands of the cat. J. Physiol. 82: 121–127.
Flacke, W. and Yeoh, T.S. (1968a) The action of some cholinergic agonists and anticholinesterase agents on the dorsal muscle of the leech. Br. J. Pharmacol. Chemother. 33: 145–153.
Flacke, W. and Yeoh, T.S. (1968b) Differentiation of acetylcholine and succinylcholine receptors in leech muscle. Br. J. Pharmacol. Chemother. 33: 154–161.
Battacharya, B.K. and Feldberg, W. (1958) Comparison of the effects of eserine and neostigmine on the leech muscle preparation. Br. J. Pharmacol. Chemother. 13: 151–155.
Bowman, W.C. and Rand, M.J. (1980) Textbook of Pharmacology, Section 17.30, 2nd edn. Oxford: Blackwell Scientific Publications.
< div class='tao-gold-member'>
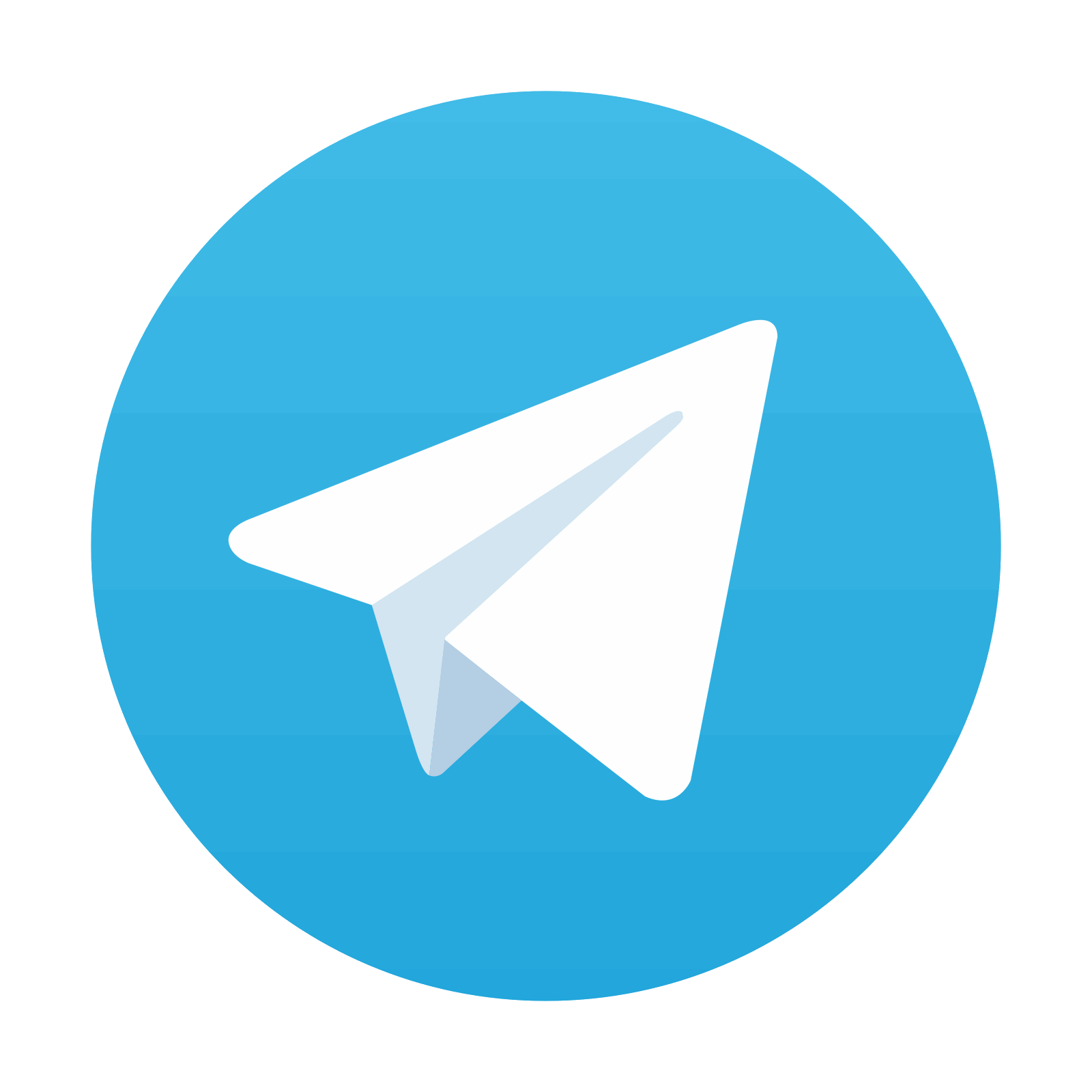