Control
Extended sperm search of ejaculates (min)
30–60
61–120
121–180
>180
Cycles
3,559
55
27
5
2
[ ] × 103/ml
58,892
3,986
15.2
0.006
0.003
Sperm seen (range)
1–729
1–87
1–53
1–25
1–3
Motile sperm (range)
1–68
0–17
0–5
0–2
0–1
MII injected
31,156
719
350
62
18
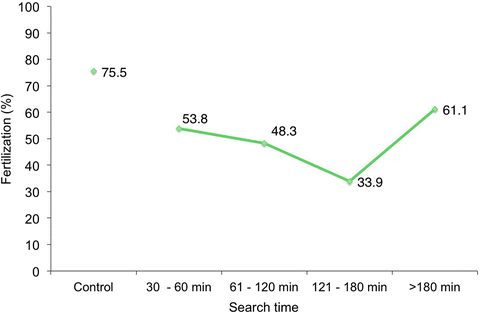
Fig. 6.1
Fertilization rate of ejaculated specimens as a function of time spent searching for an injectable spermatozoon
Table 6.2
Embryo characteristics according to length of sperm search
Control | Extended sperm search of ejaculates (min) | ||||
---|---|---|---|---|---|
30–60 | 61–120 | 121–180 | >180 | ||
Cycles | 3,559 | 55 | 27 | 5 | 2 |
Replacements | 3,162 (88.8) | 51 (92.7) | 23 (85.2) | 4 (80.0) | 2 (100) |
Embryos transferred | 7,269 | 51 | 40 | 8 | 4 |
Mean embryos transferred | 2.3 | 2.9 | 1.7 | 2.0 | 2.0 |
D3 mean blastomeres | 7.5 ± 1 | 7.3 ± 1 | 7.8 ± 1 | 8.3 ± 1 | 9.0 ± 0 |
Fragmentation rate | 6.6 ± 3 | 7.9 ± 3 | 6.4 ± 3 | 5.2 ± 2 | 3.7 ± 3 |
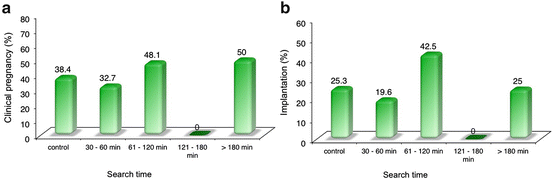
Fig. 6.2
Clinical pregnancy (a) and implantation (b) rate, according to extended sperm search time
In case of azoospermia or complete asthenospermia at the time of egg retrieval, if the patient is willing to consider the surgical risks and obvious discomfort related to surgery, then testicular sampling can be considered for retrieving spermatozoa.
Due to the limited number of nonideal spermatozoa identified within ejaculates, we wondered about the genomic integrity and competence of these cells. DNA damage can either be single-stranded nicks or double-stranded breaks. DNA strand breakage can occur either by free radical attack generated by the metabolic process of the spermatozoa or contaminating cells. It can also be that the spermatozoon’s chromatin was not properly compacted, thus making it more susceptible to oxidative stress and enzymatic cleavage by endonucleases [59].
From this, we wondered where does the actual DNA damage occur? Was chromatin adversely affected during spermiogenesis in the seminiferous tubules, or could it be some epididymal malfunction that allowed these chromatin-damaged spermatozoa to spill into the ejaculate? Alternatively, was sperm nuclear damage caused by reactive oxygen species in the male genital tract post-spermiogenesis?
The nature of chromatin compaction in the sperm nucleus has been thoroughly described [4, 60]. In brief, sperm DNA is compacted into doughnut-shaped protamine-rich toroids that contain the DNA in a semicrystalline state [8, 10]. Interspersed between the toroids are histone-rich linker regions of DNA, required for rapid access to facilitate transcription once the spermatozoon is inside the oocyte. Sperm DNA fragmentation can be assessed by SCSA (the gold standard), sperm dispersion assay (SCD), TUNEL, and Comet.
To quickly assess if the sperm DNA fragmentation occurred at the level of sperm production on the germinal epithelium or during its station in the epididymis, we thought to assess the men’s abstinence period. Careful review of records where males had abnormal DFI revealed that the longer the abstinence period, the higher the sperm DFI (p < 0.05). Moreover, when dynamic parameters were assessed, we observed an inverse relationship between motility and chromatin fragmentation: as motility declined, DNA fragmentation progressively increased—a finding confirmed on all assays [8].
We then looked at a special group of patients where we concurrently assessed the DFI at different levels: at the ejaculate, the epididymis, and within the seminiferous tubule. In 12 men with recurrent ICSI failure (mean = 3.5 cycles/patient), their ejaculated spermatozoa yielded a fragmentation rate of 54.8 ± 29 % (up to 96 %). Here, we saw that the incidence of DNA fragmentation was remarkably higher in the ejaculate, somewhat lower in the epididymal sperm, and more drastically reduced when sperm were retrieved via testis biopsy (p = 0.007) (see Fig. 6.3). These were non-azoospermic men with high DFI in their ejaculated sperm (ranging from 19 to 96 %); after thorough counseling, they opted to undergo testicular biopsy.
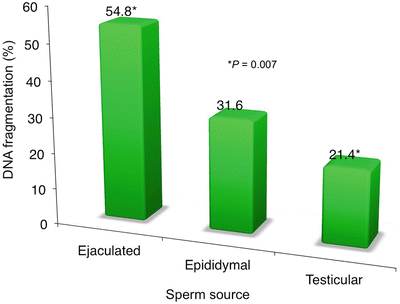
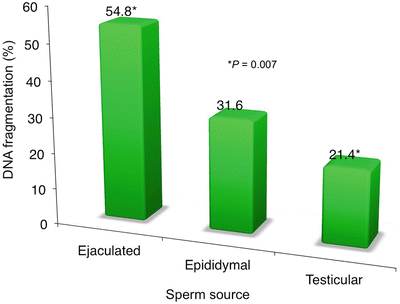
Fig. 6.3
Sperm DNA fragmentation classified by sperm source
On the basis of this observation and on some published reports [61–63], we were able to compare ejaculated (n = 28) and TESE sperm (n = 13) cycles. While fertilization was higher with ejaculated (60.0 %) than testicular (46.9 %; p < 0.01), the embryo cleavage rate was comparable. Following the replacement of an average of 2.8 embryos, the clinical pregnancy was 10.7 % (3/28) for the ejaculate sperm group and 30.8 % (4/13) for the TESE [61].
In a subset of individuals (n = 8), whose semen characteristics were given in Table 6.3, a paired analysis was carried out with the ejaculated cycle closest in time to the TESE cycle. The fertilization rate was comparable between the ejaculated sperm cycles and the surgically retrieved sperm cycles at 55.9 % and 50 %, respectively. The embryo cleavage was lower in the ejaculate at 63.6 % resulting in a pregnancy rate of 12.5 %, while in the TESE cohort all embryos cleaved resulting in a 25.0 % clinical pregnancy rate. This study argues in favor of utilizing a testicular sperm sample when a higher DFI is measured in the ejaculated specimen.
Table 6.3
Semen characteristic of men who had DNA fragmentation assessment on both ejaculated and surgically retrieved specimens
Ejaculated | Surgically retrieved | |
---|---|---|
Men | 8 | |
Male age | 41.1 ± 5 | |
Concentration | 12.8 ± 19 | 0.41 ± 0.50 |
Motility (%) | 29.0 ± 30 | 4.3 ± 2 |
It appears that sperm DNA fragmentation in these infertile men is most likely caused by a post-spermatogenic insult, as this is confirmed by the direct relationship between the increased DFI and the lengthening of the abstinence period. Although patients should be counseled about attendant surgical risks, potential anesthesia complications, and the possibility that even with TESE sperm may not be recovered and pregnancy may not occur, the initial results are encouraging. This is a clear indication that damage to the sperm nucleus occurs post-spermiogenesis and is obviated by utilization of sperm obtained more proximally. From these data, we can conclude that in couples with recurrent pregnancy failures with ejaculated specimen evidencing high sperm DNA fragmentation, the option to undergo testicular biopsy should be offered after appropriate counseling.
Future Approaches
A number of new technologies are undergoing refinement to allow better sperm selection to be used for standard in vitro insemination and ICSI. Epigenetic techniques (i.e., characterization of sperm DNA methylation patterns in developmental genes) have been shown to influence embryonic growth competence [64]. Interesting findings are emerging from proteomics that also show promise in assisting sperm selection [65–67]. Likewise, mRNA assessment in mature human sperm cells [68, 69] may be used as a transcriptomic screening technique particularly for idiopathic forms of male infertility [70, 71]. The application of in vitro metabolomics to identify genetically compromised cells [72] (or used to profile blood samples) could also serve as “fertility indicator” [73].
GM1 (Monosialotetrahexosylganglioside)
At present, we are testing a method to measure the intrinsic ability of the male gamete to exert its fertilization task as a way to facilitate single embryo transfer. This approach is based on the physiologic fact that ejaculated spermatozoa are not immediately able to fertilize an egg. Rather, they must undergo a process of functional maturation known as “capacitation.” Currently, there are no sensitive and simple markers for capacitation that can be used in a clinical setting. A close approximation of such a test might be based on protein tyrosine phosphorylation events during capacitation, as this technique has been described in sperm from other species [71, 74–77]. However, the necessary polyacrylamide gel electrophoresis and immunoblotting processes may require ~48 h to complete, making it poorly suited for clinical purposes [75, 78].
Redistribution of GM1 ganglioside in sperm induced to undergo capacitation can be used as a method for both diagnostic and predictive purposes, to assess sperm reproductive fitness. The redistribution of GM1 during capacitation in distinct patterns has been seen in all mammalian species examined, including the bull, boar, stallion, and human [76, 79]. This assay can assess functional activity of spermatozoa before embarking on ART, to determine which insemination method is appropriate (conventional IVF vs. ICSI) [80, 81].
3D Video Imaging
As with other dynamic time-sensitive morphometric techniques to identify the ideal embryo that will most likely develop to blastocyst [82], we have adapted genetic assessment and molecular markers to screen the proportion of competent spermatozoa. Noninvasive techniques under development (i.e., 3D video imaging technology) aim to link selected bio-morphometric sperm parameters with the 360° shape of each sperm cell and its inner chromatin structure. The future holds continued application of such methods, corroborated by TEM, SEM, cytogenetic chromosomal mapping, and eventually proteomics [83].
Conclusions
Against the background of aspiring to move toward single embryo transfer (where preimplantation diagnosis mandates ICSI to avoid polyspermy), we unfortunately have no real knowledge on the long-term effect of utilizing suboptimal gametes of men with severe male factor on ICSI offspring. The contribution of the paternal genome to the development of the conceptus is definitively creating more awareness and receiving more scrutiny. As novel sperm selection methods continue to emerge, most will offer only aggregate data and do not allow selection of individual sperm cells. High microscopy magnification or disaccharide polymer markers are currently to evaluate single sperm cells, but these methods await verification. Increasing emphasis on single embryo transfer has necessarily led to a corresponding heightened interest in sperm, thus stimulating the quest for new tools to more accurately diagnose and select individual spermatozoa prior to direct injection. This will allow us to counsel and treat couples with greater confidence and efficacy and at the same time assuage the passing onto the progeny any nonideal paternal genetic condition when treating IVF couples—one embryo at a time. What is certain is that the identification of the highest-quality sperm to be used in ART will continue to have a direct and welcome effect as a catalyst, promoting further research in this area.
Acknowledgments
We thank the clinicians, embryologists, andrologists, and scientists of The Ronald O. Perelman and Claudia Cohen Center for Reproductive Medicine and Urology Department, Weill Cornell Medical College. We are grateful to Theodore Paniza and Laura Park for their assistance in the laboratory work and to Dr. Brian Levine for generating a 3D model of the spermatozoon.
Conflict of Interest The authors disclose no conflict.
References
1.
Hull MG, Glazener CM, Kelly NJ, Conway DI, Foster PA, Hinton RA, et al. Population study of causes, treatment, and outcome of infertility. Br Med J (Clin Res Ed). 1985;291:1693–7.CrossRef
2.
3.
4.
Carrell DT. Paternal influences on human reproductive success. Cambridge: Cambridge University Press; 2013. p. 1–195.CrossRef
5.
World Health Organization. WHO laboratory manual for the examination and processing of human semen. 5th ed. Geneva: World Health Organization; 2010.
6.
Cohen J. Cross-overs, sperm redundancy and their close association. Heredity (Edinb). 1973;31:408–13.CrossRef
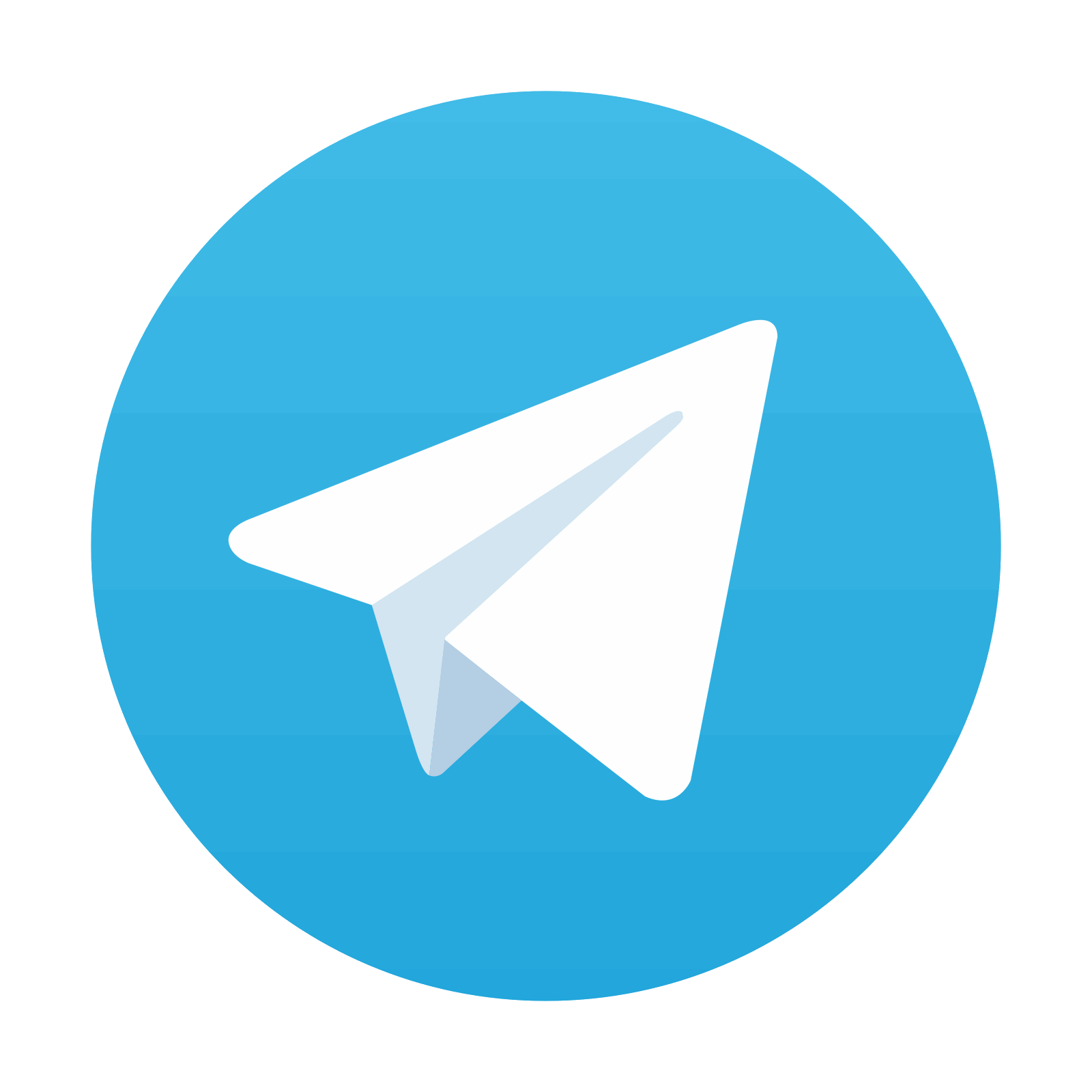
Stay updated, free articles. Join our Telegram channel

Full access? Get Clinical Tree
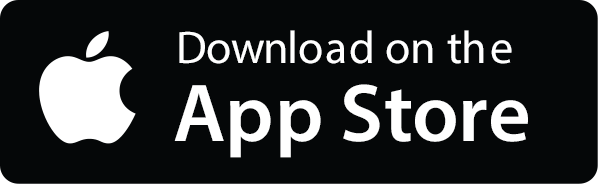
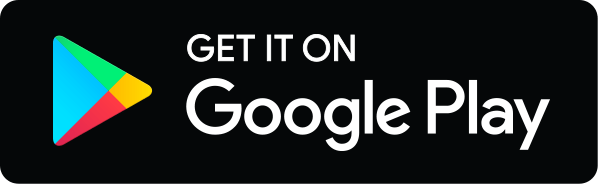