INITIAL TREATMENT OF SHOCK
Cardiovascular failure, or shock, can be caused by (1) depletion of the vascular volume; (2) compression of the heart or great veins; (3) intrinsic failure of the heart itself or failure arising from excessive hindrance to ventricular ejection; (4) loss of autonomic control of the vasculature; (5) severe untreated systemic inflammation; and (6) severe but partially compensated systemic inflammation. If the shock is decompensated, the mean blood pressure or the cardiac output (more precisely, the product of the pressure and output) will be inadequate for peripheral perfusion. In compensated shock, the perfusion will be adequate but only at the expense of excessive demands on the heart. Depending on the type and severity of cardiovascular failure and on response to treatment, shock can go on to compromise other organ systems. This chapter discusses the cardiovascular and pulmonary disorders associated with shock.
Hypovolemic shock (shock caused by inadequate circulating blood volume) is most often caused by bleeding but may also be a consequence of protracted vomiting or diarrhea, sequestration of fluid in the gut lumen (eg, bowel obstruction), or loss of plasma into injured or burned tissues. Regardless of the etiology, the compensatory responses, mediated primarily by the adrenergic nervous system, are the same: (1) constriction of the venules and small veins in the skin, fat, skeletal muscle, and viscera with displacement of blood from the peripheral capacitance vessels to the heart; (2) constriction of arterioles in the skin, skeletal muscle, gut, pancreas, spleen, and liver (but not the brain or heart); (3) improved cardiac performance through an increase in heart rate and contractility; and (4) increased sodium and water reabsorption through renin-angiotensin-aldosterone as well as vasopressin release. The result is improved cardiac filling, increased cardiac output (both directly by the increase in contractility and indirectly through increased end-diastolic volumes), and increased blood flow to organs with no or limited tolerance for ischemia (brain and heart).
The symptoms and signs of hypovolemic shock are many and can be caused either by the inadequate blood volume or by the compensatory responses. Some signs manifest themselves early, in mild forms of shock. Some present late and only in severe forms of shock. The goal is to pick up on the early signs. Doing so can save a life.
As early signs of shock, the physician might find it difficult to gain intravenous access. The skin might be cold (a nonspecific sign, but an early sign). But, by far and away, the most important, that is, most sensitive, of all the early signs of hypovolemic shock is diminished blood flow to the skin and subcutaneous tissues. It is a sign that needs to be elicited with care. It can be missed. It is best detected in the skin on the plantar surface of the foot, an area without pigmentation, with the color determined solely by the blood contained in the tissues. One begins by compressing the skin over the plantar surface of a toe with intense pressure, just short of being painful, followed by milking of the blood contained in the compressed area proximally, to the skin over the metatarsal phalangeal joint. The compression is then suddenly released. The previously compressed area will be profoundly pale, but will fill quickly, within a few seconds, if the patient is not in shock (and does not have peripheral vascular disease). In a hypovolemic patient, the refill takes longer. The test is usually done with the foot at the level of the heart, but if the patient is reasonably stable and if the lower extremities are not injured, it is more sensitive if it can be done with the foot raised above the level of the heart, to a height of perhaps 30 cm. Although the toe is usually used, one can also use the skin over the heel. The heel affords a larger surface for observation. One has to use different criteria, however. If the toe is used, color should begin to return within 4 seconds; if the heel is used, color should return in 2 seconds.
Postural hypotension—a fall in the systolic blood pressure of more than 10 mm Hg that persists for more than 1 minute when the patient sits up—can be a sign of early or mild shock. It is very useful in patients who are suspected of being hypovolemic from either dehydration or occult internal blood loss (eg, in a patient who might have gastrointestinal bleeding). It cannot be used, however, in very ill patients and in injured patients, who might not tolerate changes in position.
Low filling pressures in the right atrium are always present in hypovolemic shock, even in cases of mild shock, assuming there is no accompanying cardiac compression. Filling pressures high enough to distend the neck veins when the patient’s head, neck, and torso are elevated 30 degrees, in the absence of cardiac compression, rule out hypovolemic shock. Failure to see the veins suggests, but is not diagnostic of, hypovolemia.
Oliguria is a consistent finding in early shock, in the absence of a hyperosmolar-induced diuresis. A bladder catheter should be inserted in any patient suspected of being hypovolemic. Urine output is considered to be potentially inadequate if it is less than 0.5 mL/kg/h in an adult, less than 1 mL/kg/h in a child, or less than 2 mL/kg/h in an infant. The sign, if present, is both sensitive and specific. One must wait, however, for 30 minutes or so, before one knows that the sign is present.
The hematocrit will fall within minutes in the bleeding patient, or in the patient who has bled, even when the blood loss is mild, if the patient has been given asanguineous fluids. In the absence of fluid administration, however, the fall can take hours—restitution of the intravascular volume from interstitial albumin and water and electrolytes, takes time. One can make an estimate of the blood loss, if the patient has been given asanguineous fluids, by the magnitude of the fall in the hematocrit. A hematocrit fall of 3%-4% indicates that the blood volume was depleted by about 10%; a fall of 6%-8% indicates a depletion of about 20% (or 1 L in an average adult). These calculations assume that the patient has been given enough fluid to correct the hypovolemia. They also assume that the patient was not dehydrated, prior to the hemorrhage, and that the patient did not have large loses of plasma into the extravascular spaces, as in a patient with large burns, prior to a possible hemorrhage. The hematocrit in these patients can be normal in the face of even substantial bleeding.
Hypovolemic shock is easier to recognize when it becomes more severe. In moderate cases (a deficit of 20%-30% of the blood volume), the patient can be thirsty. Hypotension can be present, even in the supine position. A metabolic acidemia, usually with a compensatory rapid respiratory rate, can develop after initial resuscitation. (The acidemia is usually not present before resuscitation. The products of anaerobic metabolism in the ischemic tissues are only flushed into the circulating blood volume once some degree of reperfusion has been achieved.)
In profound hypovolemic shock (a deficit of more than 30% of blood volume), the blood pressure will always be low, even in the supine position. Cerebral and cardiac perfusion can become inadequate. Signs of the former include changes in mental status, restlessness, agitation, confusion, lethargy, or the appearance of inebriation; signs of the latter include an irregular heartbeat or electrocardiographic evidence of myocardial ischemia, such as ST–T segment depression and, over time, the appearance of Q waves. A metabolic acidemia will always be present, after initial resuscitation.
There are many pitfalls in making the diagnosis of hypovolemic shock, and every clinician will miss the diagnosis on occasion. In some patients, especially children and young adults, strong compensatory mechanisms can maintain the blood pressure at normal levels in the setting of mild to moderate shock. In other patients, one might not know if the observed pressure is abnormally low—a young patient might normally have systolic pressures in the low 100s; the same pressure in a chronically hypertensive patient might precede catastrophe. Pain-producing injuries in the absence of blood loss should produce hypertension; a normal pressure in a patient with a pain-producing injury suggests hypovolemia. Administration of a sedative or narcotic in the face of hypovolemia can produce hypotension but usually has no effect on the pressure in a normovolemic subject—one should not ascribe hypotension to sedatives or narcotics until the possibility of hypovolemia has been ruled out.
The heart rate is notoriously unreliable as a sign of hypovolemic shock. Although it increases in response to graded hemorrhage in anesthetized animals, the correlation between hypovolemia and heart rate in unanesthetized human beings is poor. Unanesthetized hypovolemic human beings often have normal heart rates. Severe hypovolemia can even produce bradycardia, as the cardiovascular system makes a last attempt to allow filling of the ventricles during diastole. A normal heart rate provides no assurance that the patient is not in shock. (A rapid heart rate, however, has to be taken seriously. It might be the only indication of shock. The pitfall is in the assumption that a normal heart rate rules out shock.)
There are at least three other pitfalls that can lead to missing the diagnosis of shock: (1) the cutaneous vasoconstriction of hypovolemic shock can be ablated by the vasodilation induced by alcohol or other pharmacologic agents, both therapeutic and recreational; the patient might have well-perfused skin even when hypovolemic; (2) the oliguria of shock can be overcome by an osmotic diuresis induced by high blood alcohol or glucose levels; and (3) shock can cause alterations in mental status that resemble intoxication with drug use or inebriation. If so, the patient might be in a preterminal state, in which the shock is so severe that cerebral blood flow becomes inadequate. The diagnosis of drug or alcohol use, as the cause of the mental abnormalities, should only be made after shock has been ruled out.
Resuscitation of patients in hypovolemic shock, either hemorrhagic or nonhemorrhagic, begins with ensuring adequacy of the airway. In many patients in shock, especially severe shock, this means preemptive intubation and mechanical ventilation. The physician cannot let uncertainty about the airway interfere with evaluation and resuscitation of other problems in the often confusing picture of shock. A patient who later turns out to need no support for the airway or ventilation can easily be extubated; failure to intubate a patient who later loses control of an airway or who can no longer ventilate means, at the least, an emergency intubation under difficult conditions and, at the worse, anoxic brain damage or death. This approach is different from the approach for medical patients with decompensated chronic obstructive lung disease, in whom intubation can mean prolonged mechanical ventilation and perhaps even failure to ever extubate. Those patients have such severe underlying pulmonary disease that even under baseline conditions they have trouble breathing. The baseline condition in surgical patients is usually perfectly adequate pulmonary function. Once the possible surgical problems are ruled out or dealt with, there is usually no problem with extubation.
If the patient requires mechanical ventilation, and almost all patients intubated for shock do, volume control ventilation, or some variant thereof, is preferable, in the initial phases of resuscitation (in the latter phases, after the dust has settled, we usually use pressure modulated ventilation—see the section in this chapter on Treatment of Acute Pulmonary Failure). The tidal volume is set at 7 mL/kg ideal body weight (IBW); the inspiratory time, at 1 second; the respiratory rate, at 15 breaths/min; and the end expiratory pressure, at 0 cm H2O, with all of these settings designed to minimize the mean airway pressure. To maintain adequate arterial oxygen saturation, the inspired oxygen concentration is set at 1.00. (The oxygen concentrations can be decreased later, after blood gases come back and the patient is more stable.)
The quick-to-intubate-and-ventilate approach described above pertains to all patients in shock with one exception—patients who present immediately after injury with life-threatening torso hemorrhage. The bleeding can be into the abdomen or into the chest or into the pericardium. The diagnosis is usually made on the basis of the clinical setting or on sonographic imaging. Some of these patients have to be intubated in the emergency room. If possible, however, one should delay intubation until the patient is in the operating room (which is the immediate destination for all of these patients, once the problem of exsanguinating cavitary hemorrhage is recognized).
A patient in profound shock from bleeding into the torso can suddenly deteriorate, to the point of losing his vital signs, with intubation and initiation of positive pressure ventilation. Positive pressure ventilation has effects on the cardiovascular system. All of them are bad, and all are worsened in the face of hypovolemia. The positive pressure compresses the superior and inferior venae cavae, increasing the time needed to fill the right atrium and right ventricle during diastole. (Normal negative pressure ventilation, spontaneous ventilation, decreases the time needed.) Positive pressure squeezes the thin-walled chambers of the heart (the atria and the right ventricle), making it harder for the chambers to fill during diastole. (Negative pressure ventilation makes it easier for the chambers to fill.) Positive pressure squeezes the pulmonary artery, pulmonary microvasculature, and pulmonary veins, making it hard for the right ventricle to transmit its generated energy into the pulmonary artery. (Negative pressure makes it easier to transmit the energy.) Positive pressure ventilation also compresses the pulmonary veins, mandating longer times for the left atrium to fill during diastole. (Negative pressure allows for quicker filling.)
If the patient loses his vital signs in the operating room, with intubation, the surgeon and anesthesiologist will be ideally situated to deal definitively with the underlying problems of hemorrhage and airway management. In contrast, if the patient is intubated in the emergency department and loses his vital signs, the team will be at a major disadvantage in dealing with the underlying problems. An example is in the patient with a gunshot wound to the abdomen who losses vital signs shortly after intubation in the emergency department. Opening the chest will be done hastily, under suboptimal conditions and it is unlikely to be of help. The maneuver does not deal with the underlying problem-bleeding into the abdomen. The patient needs a celiotomy, which cannot be done in the emergency department.
Direct pressure should be the first maneuver in controlling external bleeding from any part of the body. Commercially available tourniquets or improvised pneumatic tourniquets can be used for bleeding from extremities. They are easy to apply and are usually safe for up to 2 hours, with the caveat that bleeding should be controlled quickly so as to minimize tourniquet time. Several commercially available, FDA-approved hemostatic agents are available for temporary control of external bleeding. They have been proven to be effective in prehospital and combat environments and can be effective in the hospital setting as well. Blood in the pleural cavities should be drained with a chest tube. Expansion of the lung will decrease further bleeding from the pulmonary parenchyma through apposition of the visceral and parietal pleurae and will reestablish ventilation through the compressed lung. The tube will also allow monitoring for ongoing bleeding, providing key information that can prompt early operative intervention. Bleeding from pelvic fractures may require temporary binder placement. More definitive control can come later, with angiographic embolization or surgical control. Major long bone fractures should be immobilized. Although it might not be necessary to say, patients with intra-abdominal or intractable intrathoracic bleeding should be prepared for the operating room.
Blind clamping, without direct visualization, in the emergency room, should not be attempted, in the initial control of external bleeding. It can result in target vessel injury or, worse, adjacent venous laceration with increased bleeding.
Vascular access is best obtained with percutaneously placed, large-bore (ideally 14-gauge or larger) venous catheters. The catheters can be placed in superficial veins in the upper extremities, in central veins at the thoracic outlet, or in the femoral veins; catheters can also be placed in the saphenous veins, by cutdown, or directly into interosseous sites (sternum, tibia, or humerus) using specially designed needle introducer systems (FAST-1, EZ IO), the major advantage of the latter devices being the minimal training required to achieve successful access. Choice of access depends on severity of the shock, pattern of injury, provider experience, and consequences of a complication with the access, should one occur. If veins in the lower extremities are to be used, they must be decannulated within 24 hours to minimize the risk of thrombosis and infection. Gaining central venous access can be dangerous as patients in hypovolemic shock will frequently have collapsed central veins. Urgency of placement predisposes to errors in technique. A pneumothorax or an unintentional arterial puncture in the unstable patient might prove fatal.
Initial fluid resuscitation begins with a warmed crystalloid solution. Either normal saline or lactated Ringer solution can be used. Use of lactate in the resuscitative fluid is reasonable if the shock seems to be severe and if the arterial pH is likely to be less than 7.20. The lactate will buffer the hydrogen ions that are released into the central circulation with the initiation of resuscitation. The resultant lactic acid is then oxidized in the liver to carbon dioxide and water, which are excreted by the lungs and kidneys.
If the arterial pH is not likely to be excessively low, normal saline can be used as the initial resuscitative fluid. A modest hyperchloremic acidemia in the immediate postresuscitative state might favorably change the conformation of albumin molecules, decreasing movement of plasma into the interstitium. The acidemia might also increase myocardial contractility.
Blood flow to the liver is not a factor in deciding on lactated Ringer versus normal saline. Even minimal flow, as in severe shock, will be enough to deliver the buffered hydrogen ion to the liver parenchyma. Lactated Ringer should not be used, however, if the patient has preexisting liver disease. Adequate oxidation requires functioning liver cells.
The rate at which the initial crystalloid resuscitation should be given depends on two factors: (1) the severity of the shock and the presence of uncontrolled bleeding. When bleeding has been controlled, resuscitation to normovolemia is the goal. (2) Two L is given as fast as possible, followed by a third liter infused over 10 minutes if necessary. This amount of fluid will resuscitate most patients in whom hemorrhage has been arrested.
If the patient is not resuscitated with this amount of fluid, one should renew the search for bleeding, which might be into the chest, abdomen, or retroperitoneum. In addition, one should begin to use blood products, while cutting back on asanguineous fluids.
Transfusion therapy can be life saving, but it is not without risk. Transfusion reactions, transmission of blood-borne pathogens, acute lung injury (ALI), and immunomodulation can all arise from the administration of blood products. In the acutely bleeding patient, allogeneic packed red cells should be used when the estimated blood loss exceeds 1.5 L (30% of the blood volume), and possibly earlier if there is the potential for ongoing bleeding or if the patient is at risk for having coronary artery disease (Table 12–1).
Coronary Artery Disease (CAD) | Environment | Likelihood of Bleeding | Desired Hematocrit (%) |
---|---|---|---|
No | ICU | Unlikely | 21 |
No | ICU | Possible | 21 |
No | ED | Unlikely | 24 |
No | ED | Possible | 27 |
Yes | ICU | Unlikely | 271 |
Yes | ICU | Possible | 302 |
Yes | ED | Unlikely | 332 |
Yes | ED | Possible | 362 |
In urgent settings, type-O blood should be given to restore circulating blood volume. Rh-positive blood can be given to men and women who are beyond childbearing age; Rh-negative blood has to be used in women of childbearing age. The patient’s blood type can be determined within 10 minutes, in most hospitals, and type-specific, uncrossed matched red cells should be used when they become available (except in the case of massive casualties, when type O, Rh-negative universal donor cells should be used, to minimize the chance of giving the wrong type of blood to a misidentified patient). Type specific cells should also be used in the setting of massive transfusion, when cross-matching becomes impossible because of the heterogeneous origin of the circulating cells following rapid blood volume replacement.
If resuscitation is ongoing and if it appears as though the total blood loss is likely to exceed one blood volume in 12 hours, plasma and platelets should be given in amounts sufficient to approximate a 1:1:1 ratio. This should be determined as early as possible to avoid dilutional coagulopathy. “Massive transfusion protocols” can maximize efficient use of blood products through the utilization of predetermined component ratios, bedside refrigeration, and in some cases, protocol-driven laboratory monitoring. Crystalloid infusion can be restricted in the case of massive transfusions—adequate amounts of crystalloids will be infused in conjunction with the blood products.
Giving excessive amounts of fluids in an effort to restore the blood pressure to normal or supranormal levels will create edema. Edema in the gut can create an abdominal compartment syndrome, with compression of the inferior vena cava, displacement of the diaphragm into the chest, and compression of the heart and lungs; edema in the liver can lead to compression of biliary ductules and inability to excrete bilirubin into the gut; edema in the lungs can hinder ventilation and oxygenation; and edema in wounded tissues can impede healing and the ability to fight off infection. Unnecessarily high blood pressures can also potentially exacerbate bleeding. On the other hand, inadequate resuscitation can leave the patient exposed to the many adverse late effects of prolonged shock, such as multiorgan failure.
The primary goal in fluid resuscitation for all forms of shock is the same: restoration of adequate end organ perfusion, the product of the mean pressure perfusing the organ and the blood flow to the organ. Resuscitating to a brachial systolic pressure of 80 mm Hg or a radial pulse in the bleeding patient is reasonable until hemorrhage has been controlled. (The treating physician will not know the cardiac output or organ blood flow in the early phases of resuscitation, but he or she can use clinical criteria, such as skin perfusion, urine output, and mental status, in managing the patient, along with the blood pressure.) After the bleeding is controlled, a systolic pressure of 90 mm Hg might be reasonable. In the very old, the blood pressure goal should be higher to ensure a cushion of perfusion for the brain, heart, and other viscera that might be supplied by arteries obstructed by atherosclerosis.
One must keep in mind during the resuscitation, however, that using the blood pressure to define severity of shock or to assess the adequacy of resuscitation can be hazardous. Most patients in hypovolemic shock will be able to constrict the arterioles in their skin, muscle, and visceral organs in response to the hypovolemia (the exception being inebriated patients). They can often maintain a normal pressure even in the face of continued shock. One must consider many variables in assessing adequacy of resuscitation, including peripheral perfusion, urine output, mental status, acid-base balance, and resolution of any signs of myocardial ischemia.
After severe trauma or major sepsis, many patients will demonstrate signs of intravascular coagulation, with prolonged clotting times, low platelet counts, decreased fibrinogen levels, and production of fibrin degradation products or fibrin monomers. Correction requires administration of plasma and platelets, especially to patients who continue to bleed and to those with severe head injuries, in whom intracranial bleeding could be devastating.
Traditional replacement of coagulation factors has been done with thawed frozen plasma. The advantages of frozen plasma are logistical; the disadvantage is that it has to be thawed prior to use, which may take from 30 to 60 minutes. Increasingly, trauma centers are using either prethawed plasma or “liquid” plasma that has never been frozen. The advantage of the former is that the product does not have to be thawed; the major disadvantage is the potential for waste. “Liquid” plasma has the potential advantage of increased factor activity with the disadvantage of waste. Finally, there has been interest in developing freeze dried plasma, particularly by the military. Although the Europeans have extensive experience with the product (it is approved for use in Europe), there is currently no FDA approved formulation in the United States.
Over the last decade, pharmacologic therapy for hemorrhage-related coagulopathy has become a standard adjunct in the setting of hemorrhagic shock. In the recent past, recombinant activated factor VII has been used routinely for severely injured patients with ongoing traumatic coagulopathy. Administration of doses between 80 and 100 mcg/kg is generally recommended for coagulopathy in trauma patients. Smaller doses may be useful for warfarin reversal in the setting of intracranial hemorrhage. Due to the mechanism of action, platelet and fibrinogen levels need to be adequate at the time of administration.
Tranexamic acid (TXA) is an antifibrinolytic agent that has recently been adopted for routine use by the military as a pharmacologic adjunct for hemorrhage control (REF). It is being used with increasing frequency in civilian settings. The impetus for use in trauma originated from a large prospective international trial (CRASH-2) as well as a large retrospective cohort of combat casualties. Although there is no universally recommended monitoring when using the product, it seems reasonable to think that it should be particularly useful in the presence of excessive fibrinolysis, which can be assessed with the use of the thromboelastogram (TEG), a test that is being used more and more often in diagnosing fibrinolysis in the bleeding patient.
Using pharmacologic agents to improve hemostasis can lead to procoagulant side effects. These have been well documented with activated factor VII but are less well known with antifibrinolytic agents such as TXA. If the patient is not bleeding and is not at risk for a devastating consequence of rebleeding, one should not give procoagulant factors—they will only fuel the fire of systemic inflammation and coagulation. As with any intervention, the benefit to risk ratio should be individualized.
Maintenance of normothermia is critical in the setting of hemorrhagic shock. Patients who have significant blood loss may lose the ability to increase metabolic heat production. In addition, initial fluid replacement is often done using room temperature or cold solutions such as crystalloid administered in the field by paramedics or unwarmed blood products in the hospital. The added stress of environmental or surgical exposure can make it difficult to maintain normothermia. The impact cannot be overstated. The adverse effects of hypothermia on coagulopathy can be profound.
For these reasons, hypothermia prevention and treatment should be an immediate and ongoing management priority during resuscitation from hemorrhagic shock. At the very least, one should keep the environment warm, infuse fluids through warmers, and keep the patient covered, as much as possible. These efforts should commence as soon as possible after the injury or after the onset of illness.
There is no role for using colloid solutions in the setting of hypovolemic shock except when size and weight of the crystalloid solutions limit their availability, as in treating mass casualties or under wartime conditions. Under these circumstances, solutions containing hetastarch or hypertonic saline and dextran can be used. Otherwise, colloids provide no benefit over crystalloid solutions, and they add to cost. Vasopressors should not be used in resuscitating neurologically intact hypovolemic patients, except in desperate situations for short periods while the vascular volume is reexpanded. The idea that vasopressors divert flow from nonessential organs to essential organs is ill-conceived. Although some organs can withstand ischemia for longer periods of time than others, there are very few parts of the body that are not essential. Patients given vasopressors in shock are at risk for ischemic gangrene of the limbs, gut necrosis, liver failure, and acute tubular necrosis. Vasopressors, however, are often indicated in patients in neurogenic shock, because those patients may have lost critical physiological compensatory responses (see section on Neurogenic Shock).
Elevation of the lower extremities above the level of the heart (Trendelenburg position) in a normovolemic subject shifts blood to the heart and increases ventricular end-diastolic volumes. In the hypovolemic patient, however, adrenergically mediated venoconstriction has likely already achieved this shift. Thus the position is of little value in treating hypovolemic shock, and its awkwardness can make evaluation and treatment of other problems more complicated. The position, however, is useful for the treatment of neurogenic shock.
In trauma patients, the pneumatic antishock garment can be useful for temporary compression of bleeding sites that cannot be controlled by other means, for temporary stabilization of pelvic fractures, and as a temporary expedient to increase the blood pressure during transport in patients in neurogenic shock. It has no other uses. It limits the physical examination. It precludes use of the veins in the lower part of the body as sites for venous access. It can hinder filling of the ventricles by compressing the inferior vena cava and the renal and hepatic veins. It can hinder left ventricular ejection by compressing the arterioles in the lower body. It can push the diaphragm into the chest and interfere with ventilation. It is of no use in displacing blood from the periphery to the heart in neurologically intact patients—discharge of the adrenergic system will already have achieved that goal.
Cardiac compressive shock can arise from any condition that compresses the heart or great veins, including pericardial tamponade, tension pneumothorax, massive hemothorax, rupture of the diaphragm with encroachment of abdominal viscera into the chest, and distention of the abdomen with compression of the intra-abdominal great veins and elevation of the diaphragm into the chest. All of these conditions are worsened if the patient has to be mechanically ventilated.
The signs of compressive shock are similar to those of hypovolemic shock—postural hypotension, poor cutaneous perfusion, oliguria, hypotension in the supine position, mental status changes, electrocardiographic signs of myocardial ischemia, metabolic acidemia, and hyperventilation—combined with distended neck veins. The only other type of shock that can produce the combination of poor perfusion associated with distended neck veins is cardiogenic shock, which rarely poses a problem in differential diagnosis. Cardiogenic shock usually develops against a background of evident disease that predisposes to primary myocardial dysfunction. Cardiac compression usually follows trauma or occurs in a setting where mechanical compromise of the heart or great veins can arise from imposition of external pressure (as in a possible pericardial tamponade).
The so-called paradoxic pulse is occasionally helpful in diagnosis. A spontaneous breath in a normovolemic subject without cardiac compression produces little effect on systemic blood pressure. If the heart is compressed, the systolic pressure can fall by more than 10 mm Hg. (In contrast, a fall in the blood pressure with positive pressure ventilation is common and nonspecific, especially in hypovolemic patients; the concept of a paradoxic pulse applies only to patients who are breathing on their own.)
The diagnosis of cardiac compression is facilitated if the patient can be monitored in an intensive care unit (ICU) with a pulmonary artery catheter, when small stroke volumes in the face of high filling pressures can be documented by direct measurement. In addition, the catheter can be used to compare pressures in the left and right atria. Under normal circumstances, the pressure in the left atrium is about 5 mm Hg higher than in the right. In tamponade, the pressures are the same.
Infusion of fluid can transiently overcome some of the ill effects of cardiac compression, but the cause of shock in these patients is mechanical, and definitive treatment must correct the mechanical abnormality. Treatment of compressive shock caused by large-volume, high-pressure mechanical ventilation is discussed later in this chapter in the section on mechanical ventilation.
Cardiogenic shock can arise from several causes, including arrhythmias, ischemia-induced myocardial failure, valvular or septal defects, systemic or pulmonary hypertension, myocarditis, and myocardiopathies. Of all the forms of shock, it can be the most resistant to treatment. If the heart cannot pump, there may be nothing that can be done. On the other hand, in less severe cases, it is possible to improve the efficiency of the pumping capability that remains.
The diagnosis of cardiogenic shock usually depends on recognizing an underlying medical condition predisposing the heart to dysfunction in conjunction with an abnormal electrocardiogram. Given the relative oxygen demands of the left and right ventricles, shock caused by left ventricular failure is much more common in patients with ischemic heart disease and typically presents with chest pain, a third heart sound, rales, ST segment elevation on a 12-lead electrocardiogram, and, on chest film, an enlarged heart or pulmonary edema. Cardiogenic shock may be associated with distended neck veins if the right ventricle has failed, unless the patient is also hypovolemic, as in a bleeding patient with a recent myocardial infarction. In many cases, patients with recent shock or trauma may have mild to moderate forms of right ventricular dysfunction related primarily to the heart’s response to systemic inflammation or elevated ventilator pressures. Right-sided dysfunction can be associated with peripheral edema, an enlarged and tender liver, and, on chest film, an enlarged heart. The diagnosis is usually easy, but two common situations may pose a problem.
The first is a ruptured abdominal aortic aneurysm in a patient with coronary artery disease. The patient might have abdominal pain consistent with a myocardial infarction and electrocardiographic signs of ischemia—the ischemia being caused by hypovolemia and shock. The key is to observe the neck veins.
The second is to ascribe shock to myocardial contusion in a patient who has just suffered a blunt injury to the chest. Although blunt chest trauma can damage the heart, the damage is usually either fatal, with death at the scene of the injury, or, more often, of no clinical significance. A contusion that produces failure but not death is rare. Shock after blunt trauma in a patient who survives to reach the hospital is almost never caused by contusion—it is far more likely to be caused by hypovolemia or by a mechanical problem.
In the initial treatment of acute dysrhythmias, we use the approach described by Ursic and Harken (ref in the ACS textbook). A bradydysrhythymia in a hypotensive patient with a heart rate less than 50 beats/min deserves treatment, even if the ventricular contractions are well coordinated. One should begin with the intravenous administration of atropine, at a dose of 0.5 mg, repeated at 2-minute intervals for a maximum dose of 2 mg. If the rate remains slow and if the patient is still unstable, the heart should be paced by transvenous or external means.
A tachydysrhythmia can put a patient at risk for myocardial ischemia regardless of origin (sinus vs nonsinus) or etiology (hypovolemic or cardiogenic shock). Thus one has to decide if the rate is so rapid that it threatens the patient’s myocardium. As a first approximation, the goal for most patients should be a ventricular rate between 50 and 100. But the goal can be different for different patients. The maximal ventricular rate, the rate that can be sustained for perhaps 5 minutes, but not longer, declines with age (220—age in years). The maximal aerobic rate, a rate that can be maintained indefinitely, but with strain, is 60%-90% of this value depending on the physical condition of the patient and the presence or absence of ischemic heart disease. For example, a healthy 20-year-old man in good condition should be able to tolerate and sustain a heart rate of 160 (80% of 200) without difficulty (although one should search for the cause of the tachycardia). On the other hand, a 65-year-old man with known coronary stenosis may have a myocardial aerobic threshold of 93 beats/min:
Rates exceeding these limits should be treated.
A moribund patient with a tachydysrhythmia should undergo electrical cardioversion, an intervention that result in full cardiac function with normal blood supply to the brain in a matter of seconds. No other treatment has this potential. Electrical cardioversion is the treatment of choice for coarse ventricular fibrillation, ventricular tachycardia, and for supraventricular dysrhythmias with unsustainably rapid ventricular responses. It is not the treatment of choice for asystole or fine ventricular fibrillation, but no treatment for these dysrhythmias has a chance of achieving resuscitation with full neurological function. Nothing will be gained with cardioversion in the patient in asystole or fine fibrillation, but nothing will be lost.
One hundred joules (J) should be used initially, with rapid escalation to 360 J, as needed. The cardioversion takes precedence over securing the airway, and it takes precedence over obtaining vascular access; it even takes precedence over making a diagnosis of the arrhythmia. Once converted, the patient should be given a single 100-mg dose of lidocaine. Serum electrolytes should be measured and corrected, if needed. One to two grams of magnesium sulfate should be given intravenously over 15 minutes, regardless of initial plasma concentrations. More should be given later if the values are low.
Treatment of any non-moribund patient with a tachydysrhythmia begins with ensuring euvolemia and treating other possible extracardiac causes of a tachycardia (such as fever, stress, pain, and anxiety). Dangerously rapid tachydysrhythmias associated with abnormal ventricular conduction should be electrically cardioverted, followed with lidocaine, electrolyte correction, and administration of a single dose of magnesium. In the non-moribund patient with a dangerously rapid tachydysrhythmia and normal ventricular conduction, the initial goal is to slow the rate. Give up to 10 mg of verapamil intravenously over 10 minutes. This will slow the rate in the large majority of the patients. If the initial dose fails to slow the rate, an additional dose can be given 30 minutes after the initiation of the first dose. If the ventricular response is still too rapid, give digoxin. The digoxin is administered as a loading dose of 0.5 g intravenously, followed by 0.25 g every 6 hours for two additional doses (total of 1.0 g). More might be needed—the goal is to block the AV node enough so that the ventricular response is acceptable. Daily maintenance doses are usually needed, for long-term control, and the serum levels should be measured. Electrolytes should be corrected; magnesium should be supplemented.
For continued control of the rate, we favor digoxin. But one can also continue to use verapamil or add a beta-blocker, for long-term control. All three classes of drugs–calcium channel blockers, digoxin, and beta-blockers-slow AV nodal conduction, but, of the three, only digoxin increases myocardial contractility. Surgical patients need contractility to perfuse wounded or infected tissues.
If more than one drug has to be used, the patient will need to be in a monitored setting. Complete heart block is a potentially disastrous side effect with all of these drugs, especially when used in combination.
If ventricular conduction is normal and the patient is in atrial fibrillation, and if it is thought that the fibrillation is going to be of long duration, and if the physician is sure that the patient needs an “atrial kick,” the patient can be converted to a sinus rhythm with amiodarone. This set of conditions is rarely the case, however, in the noncardiac surgical patient. The “atrial kick” provides close to no added energy production from the heart in all but the most extreme cases of myocardial dysfunction. The atria in any reasonably functional heart serve only as reservoirs of energy, as capacitors, like other capacitance elements in the circulation, like the venules and small veins. The atria allow the ventricles to fill evenly under conditions of varying heart rates, but that goal is achieved with or without active contraction of the chambers.
Amiodarone frequently results in conversion of atrial fibrillation to a sinus rhythm, but the disadvantages of the drug, in the noncardiac patient, almost always outweigh the potential benefit. Conversion can lead to embolization of clot from the atrial appendage to the brain. The drug depresses myocardial contractility (potentially impeding wound healing and the ability to fight off infection). It has a long half-life. Its effects can last for weeks.
Opioids can be especially effective in treating cardiac failure after myocardial infarction. They relieve pain, provide sedation, block adrenergic discharge to the arterioles, block discharge to the venules and small veins, redistribute the blood from the atria and ventricles to the venous capacitance vessels in the periphery, and decrease myocardial oxygen requirements.
Diuretics are the keystone of therapy in congestive heart failure with large ventricular end-diastolic volumes. By decreasing vascular volume, diuretics decrease atrial pressures and mobilize peripheral and pulmonary edema. Pulmonary vascular pressures and volumes decrease; effectiveness of right ventricular contraction increases. Coronary blood flow increases as coronary sinus pressure drops. Decreasing pressures in the ventricles during diastole, when the ventricular muscle receives its nutrient blood flow, alleviates compression of the coronary vasculature in the endocardium. Decreasing pressures in the right atrium decreases the stiffness of the coronary vasculature, which decreases the stiffness of the ventricles during diastole (the garden hose effect). The ventricular end-diastolic volumes potentially can increase without much of an associated increase in the end-diastolic pressures.
Almost all patients in cardiac failure with ischemia and a rapid heart rate will benefit from a beta-adrenergic blocking agent (eg, esmolol or metoprolol). Decreasing the rate and reducing ventricular stiffness during systole decreases myocardial oxygen requirements. Increasing time in diastole and decreasing ventricular stiffness during diastole augments ventricular filling and increases efficiency of ventricular contraction. All of these effects reduce myocardial oxygen consumption and potentially salvage marginal myocardium. In many patients, the reduced oxygen requirements can be achieved with only minimal loss of energy output from the ventricles. The only contraindication to the use of beta-blockers, beyond the rare development of bronchospasm with administration of the drugs, is hypotension. This latter problem is easily monitored.
Hypertension is unusual but not unheard of in patients with cardiogenic shock. The hypertension is usually associated with inefficient delivery of energy into the aortic root. Treatment should begin with opioids, if the patient is in pain, and then diuresis, if the ventricular end-diastolic volumes are large. Nitroprusside and nitroglycerin are the most useful short-term vasodilators in surgical patients in heart failure (besides opioids). Both drugs act quickly and are easy to monitor; both dilate the systemic arterioles; nitroglycerin also dilates the systemic venules and small veins. For long-term control of pressure, angiotensin-converting enzyme (ACE) inhibitors and calcium channel blockers should be used in place of the nitrates. If the patient has a tachycardia or, as may well be the case, if the patient is at risk for having coronary artery disease or myocardial ischemia, beta-blockade can be used.
Beneficial consequences of controlling the pressure include mobilization of edema, both pulmonary and systemic; enhanced perfusion of the myocardium; reduction of ventricular work and oxygen requirements and, consequently, relief of myocardial ischemia. On the other hand, excessive venous dilation can decrease cardiac filling enough so that stroke volumes and blood pressures fall; excessive arteriolar dilation can make the pressures fall further.
Inotropic agents, such as dobutamine or milrinone, can increase cardiac output in some, but not all, patients in cardiogenic shock. Inotropic agents almost always result in increased myocardial oxygen requirements, but this is not usually a problem. Patients receiving the agents should be monitored in an ICU. Development of chest pain or ischemic electrocardiogram changes suggest that oxygen demand is exceeding supply. If it is necessary to use inotropic agents for more than 1 hour, a pulmonary artery catheter should be inserted. Systemic arterial pressures, atrial filling pressures, and cardiac output should be determined at different infusion rates. If any question remains about the adequacy of volume resuscitation, cardiovascular parameters should be measured before and after a fluid bolus is given.
Digitalis compounds should not be used in acute cardiac failure except to control ventricular rates in patients with supraventricular tachydysrhythmias. Toxicity may develop, especially when pH and electrolyte changes are unpredictable. The inotropic actions of digitalis are no different from those of dopamine and milrinone.
Although uncommon in the surgical setting, patients with cardiac failure and a low heart rate (< 70 beats/min) may temporarily benefit from the administration of a chronotropic agent, such as dopamine. (Isoproterenol is almost never used nowadays.) When using dopamine, the heart rate should be increased only to levels that can be tolerated comfortably. A 60-year-old patient with normal coronary arteries gains little with a heart rate that exceeds 120 beats/min; the limit is about 90 beats/min in the presence of coronary artery disease. In most cases, however, the price to be paid for using a chronotropic agent exceeds the potential benefit. Chronotropic agents increase myocardial work and oxygen requirements and shorten the time during diastole for coronary blood flow and ventricular filling. They should be used only as a temporary expedient. If they are used for more than 30 minutes, a pulmonary arterial catheter should be inserted. The goal of therapy is a normal or slightly supranormal cardiac output that provides adequate end-organ perfusion and reverses shock. Trying to achieve more than that only increases the risk of myocardial ischemia.
A vasoconstrictor is occasionally useful to increase coronary perfusion pressure in the setting of coronary stenoses. To be effective, the agent must increase aortic pressure enough so that the increased myocardial perfusion compensates for the increase in the myocardial oxygen requirements.
The major untoward effect of these agents is ischemic necrosis of noncardiac organs, such as the extremities or intestine. They will not increase perfusion to the brain in the setting of cardiogenic shock, assuming that the carotid arteries are open and that the patient has a functioning adrenergic nervous system. The endogenous adrenergic nervous system is ideally suited for ensuring adequate blood flow to the brain. Constrictors should be used only when absolutely necessary and for no more than 60 minutes unless a pulmonary arterial catheter is in place.
The transaortic balloon pump decreases the hindrance that the left ventricle faces when it ejects its blood into the aortic root and can be very effective in resuscitating selected patients with severe reversible left ventricular dysfunction (eg, after cardiopulmonary bypass or acute myocardial infarction). It should be used only if a pulmonary arterial catheter is in place.
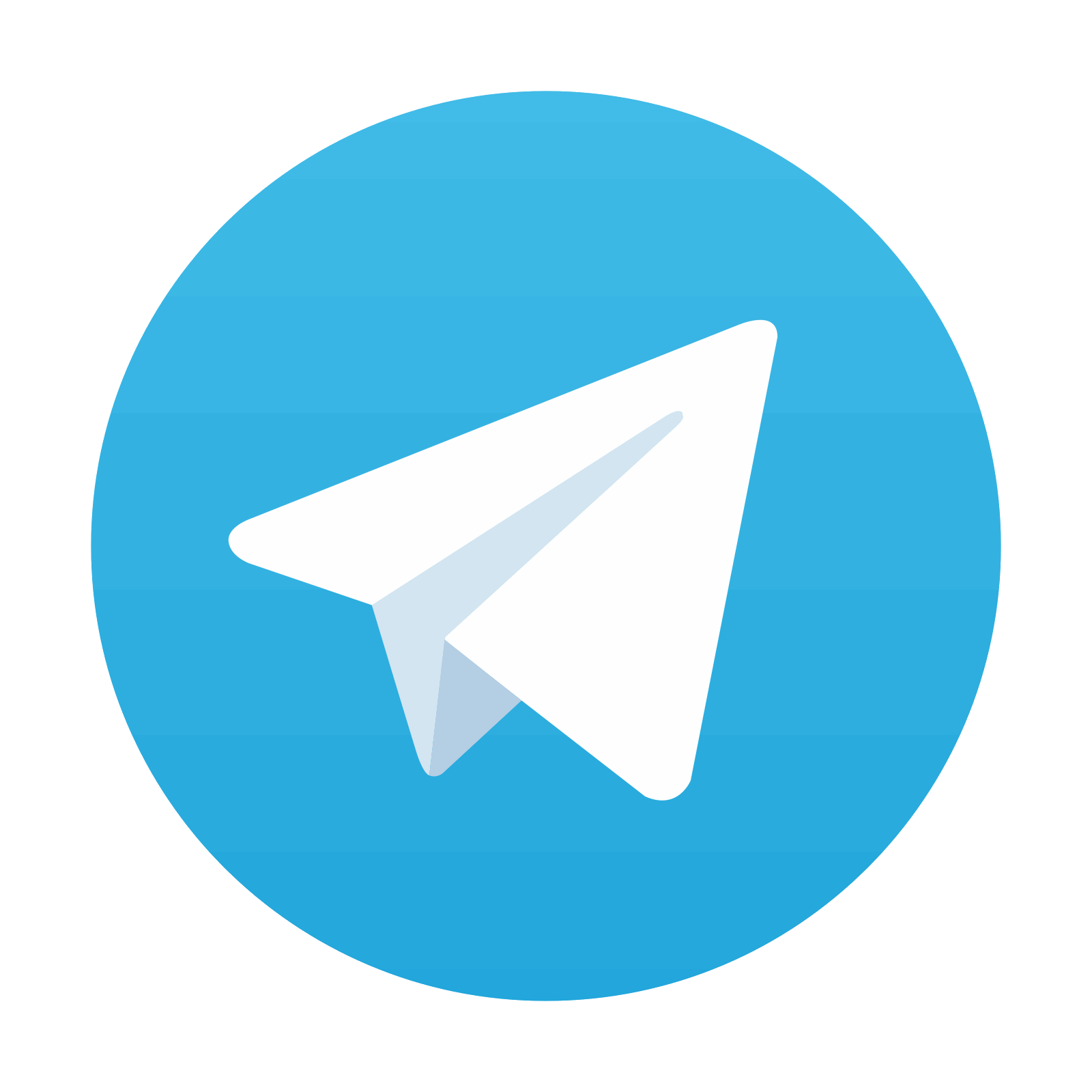
Stay updated, free articles. Join our Telegram channel

Full access? Get Clinical Tree
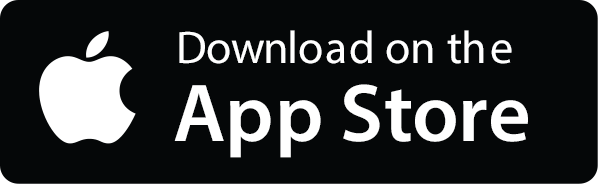
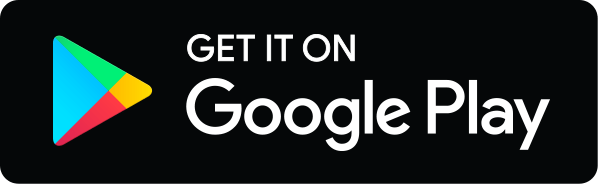