Chapter 27 The search for naturally derived anticancer agents*
In the endeavour to discover effective drugs for the treatment of various cancerous diseases, the natural kingdoms, especially the plant kingdom, have been extensively researched. The research involved has been enormous and although the number of successful outcomes appears very modest, the effective drugs produced rank among the most common chemotherapeutic agents employed. Also, the wide diversity and complexity of the compounds isolated have afforded valuable material for the manufacture of semi-synthetic derivatives, often less toxic and clinically superior to the original isolate.
PLANTS IN CANCER TREATMENT
Very successful higher plant materials used in cancer chemotherapy are the alkaloids of Catharanthus roseus. Research on this plant, the Madagascan periwinkle, was stimulated by its mention in folklore,not as a cure for cancer, but in the treatment of diabetes. No hypoglycaemic activity was detected, but treated test animals became susceptible to bacterial infection, and this led the researchers to undertake extensive examination for possible immunosuppressive principles causing these effects. A number of bisindole alkaloids showing antileukaemic activity have subsequently been isolated and two of these, vincaleukoblastine (vinblastine) and leurocristine (vincristine), are now extracted commercially from Catharanthus roseus and used, either alone, or in combination with other forms of therapy for cancer treatment. Another important, more recent, addition to the list of anticancer drugs is paclitaxel (Taxol), a diterpene derivative isolated initially from the bark of the Pacific Yew, Taxus brevifolia. Although reportedly used by Native North Americans for various conditions, it does not appear to have had any traditional cancer usage and was obtained as part of the random collection programme of the US National Cancer Institute (NCI). Taxol and related taxanes are treated below.
METHODS OF INVESTIGATION
In the more recent systematic studies for useful plant constituents, every portion of the plant and every fraction of the extract is tested biologically before any constituent is isolated and characterized. Usually only those fractions showing biological activity are studied further. Thus, one may isolate almost any class of compound as an active constituent, and it may not be one traditionally associated with a particular plant family. Even procedures involving continuous monitoring of fractions for biological activity are not free from anomalies. It is quite well known that isolated constituents of a plant drug may not give the same clinical response as a crude preparation of that plant drug. Very often, the total therapeutic activity is greater than, or different from the therapeutic activities of the individuals. Synergism or antagonism (see Chapter 7) due to the complex nature of the extract are probably the causes of such observations. It is thus possible that a fraction from a plant extract, although showing significant biological activity, possesses no single constituent with this activity. Conversely, a fraction showing no activity may still contain an active constituent. A further complication is that crude fractions may contain additional substances with delayed toxicity, causing test animals to die at about the same time as control animals.
An effective screening procedure in which a large number of crude plant extracts are to be assayed for biological activity should fulfil several criteria. It should be sufficiently selective to limit the number of leads for follow-up evaluation yet it should be highly sensitive in order to detect low concentrations of active compounds, and it should be specific so that the assay is not affected by a wide variety of inactive compounds. The preliminary screens employed in the NCI studies changed during the lifetime of the programme to avoid detecting weakly active common plant products such as polyphenols, tannins, saponins and sterols not capable of being developed into useful drugs; such dereplication (Chapters 8, 9) is now commonly employed. The routine testing of extract fractions for antitumour activity is frequently done via an in vitro cytotoxity assay, although in vitro cytotoxicity is not always an effective or reliable means of predicting in vivo antitumour activity. However, since the in vitro cytotoxicity bioassay is rapid and inexpensive, and only small amounts of extract are necessary, it is the popular method for initial tests.
Plant tissue cultures might provide a reliable source. If total synthesis of the active chemical is feasible, this will always be the preferred option. However, the extremely complex structures of most bioactive natural products frequently preclude satisfactory commercial syntheses.
Over the years, separation techniques (TLC, HPLC, chiral chromatography, etc.) and chemical structure determination (MS, NMR, UV, X-ray crystallography) have reached high levels of sophistication. For the examination of large numbers of samples, high-throughput screening is now employed (Chapter 9).
For cytotoxicity testing, human cancer cell lines obtained from the NCI are frequently employed. Plant selection for screening can be assisted by initially choosing those from an ethnomedical database and submitting them to a search for biological and chemical information in the libraries held by NAPRALERT (see Chapter 2), industry or other research centres.
ESTABLISHED NATURAL PRODUCTS AS TUMOUR INHIBITORS
The tumour-inhibitory principles isolated in screening tests are usually new natural products, spanning a wide range of structural types. Examples of these, subdivided into phytochemical groups, are listed in Table 27.1, and structures of the more promising chemicals which were subsequently evaluated in clinical tests are shown in Fig. 27.1. However, a number of the compounds isolated were, in fact, previously known natural products. These were presumably compounds which had not been subjected to rigorous testing for biological, particularly antitumour, activity. Amongst these are usnic acid, ellagic acid, the anthraquinone aloe-emodin, the quinones juglone and lapachol, pyrrolizidine alkaloids retronecine and monocrotaline, the nitrophenanthrene aristolochic acid (see ‘Serpentary’), the bufadienolide hellebrigenin acetate, the Colchicum alkaloids colchicine, demecolcine and 3-demethylcolchicine (see ‘Colchicum’). Other known compounds that proved active but were rejected on terms of toxicity, low therapeutic index, etc. were the alkaloids of Senecio, indicine N-oxide from Heliotropium indicum and the cucurbitacins. Further examples established as tumour inhibitors at much later dates than the above were the alkaloids acronycine, ellipticine, emetine and nitidine. Acronycine, from Acronychia baueri, failed in phase 1 clinical trials. The pyridocarbazole alkaloids ellipticine and 9-methoxyellipticine from Ochrosia elliptica and other related plants, together with a number of synthetic analogues appeared useful but exhibited unacceptable side-effects. However, the quaternization of 9-hydroxyellipticine to give the water-soluble 9-hydroxy-2-N-methylellipticinium acetate (elliptinium acetate) (Fig. 27.2) has produced a highly active material, of value in some forms of breast cancer, and perhaps also in renal cell cancer. A variety of such quaternized derivatives is being tested, and some water-soluble N-glycosides also show high activity. Cephaelis ipecacuanha has been used for many years as an emetic and expectorant and the principal alkaloid, emetine, was shown to have antitumour properties. Clinical usefulness was marginal, however, and some toxic effects were noted. Nitidine is a benzophenanthridine alkaloid isolated from Zanthoxylum nitidum, and has more recently been obtained from screens of Fagara species (Rutaceae). Nitidine was selected for development based on its exceptional antileukaemic activity, but was dropped owing to erratic toxicity. The closely related alkaloid fagaronine is less toxic than nitidine. Similar benzophenanthridine derivatives are present in Chelidonium majus (Papaveraceae), a plant with substantial folklore history of use in the treatment of cancers. More recently, NK 109 (Fig. 27.2), a synthetic isomer of fagaridine found in Fagara xanthoxyloides, has been found to have greater antitumour activity than any of the natural benzophenanthridine structures, coupled with excellent stability, and was entered for clinical trials in Japan.
< div class='tao-gold-member'>
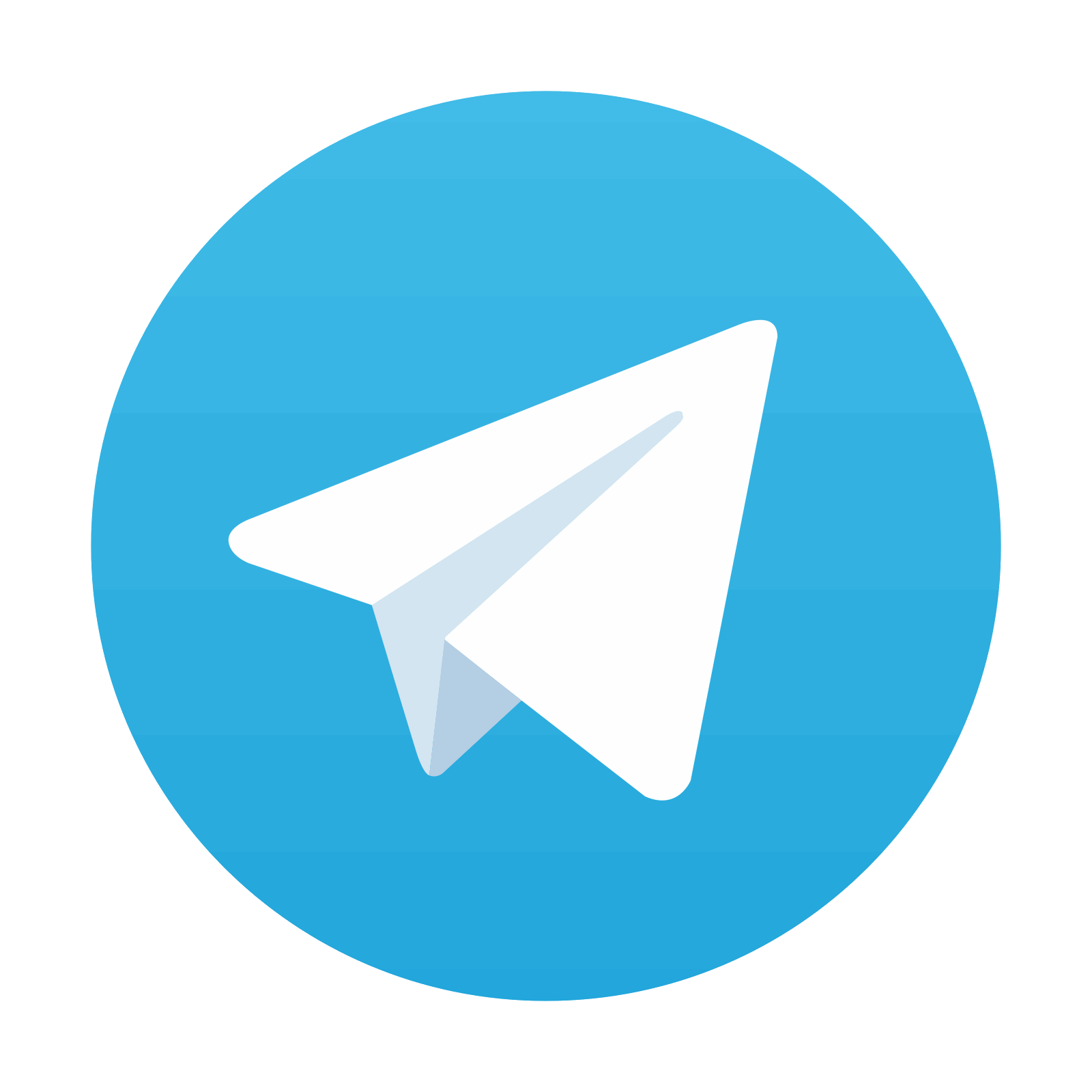
Stay updated, free articles. Join our Telegram channel

Full access? Get Clinical Tree
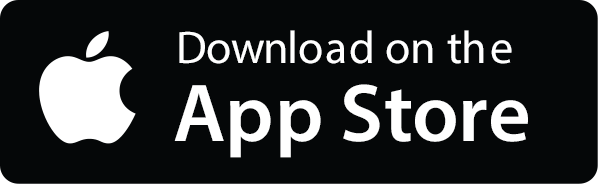
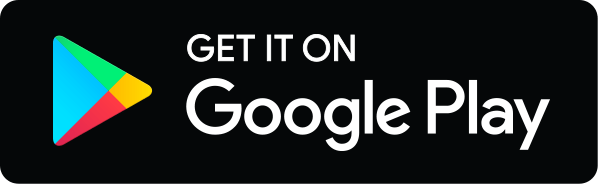