2 Safety and ergonomics in the laboratory
The goal of this chapter is to lay out a risk management plan that is applicable worldwide. While general in scope to encompass a variety of regulations, it is specific regarding the hazards unique to histology. Most of the information is from Dapson and Dapson (2005). Other references which should be in every laboratory include Montgomery (1995), the Prudent Practices Series (National Research Council 1989, 1995), aids for preparing chemical hygiene plans (Stricoff & Walters 1990), as well as guidelines from the Clinical and Laboratory Standards Institute concerning laboratory safety (2004), biohazards (2005) and waste management (2002). Indispensable publications from the Centers for Disease Control (USA) include guidelines for safety (1988), HIV and tuberculosis 1990, 1994).
Risk management
Occupational exposure limits
Most chemicals are hazardous to some degree; the question really is how hazardous are they? In other words, what would a safe level of exposure be? From many years of actual industrial experience, various agencies have developed standards for exposure to widely used chemicals. Generically, these are called occupational exposure limits, but each agency refers to its own values by unique names. OSHA’s Permissible Exposure Limits (PELs) are based upon scientifically based recommendations from the National Institute of Occupational Safety and Health, or NIOSH (2003), but are also influenced by special interest groups and Congressional actions. OSHA limits therefore typically are more lenient. Another source of exposure limits, called Threshold Limit Values (TLVs®) is ACGIH®, the American Conference of Governmental Industrial Hygienists (2004). These limits are more widely used around the world for occupational standards.
Kinds of exposure limits based upon the duration of exposure
TWA (or TWAEV). The time-weighted average (time-weighted average exposure value) is the employee’s average exposure over 8 hours. Shorter exposures may exceed this value as long as the average exposure does not. There may be some short exposure that is too high for safety; that is covered below. When additional exposure is likely through the skin, that may be noted after the TWA. This is especially true for chemicals like phenol and methanol that pass quickly through skin.
STEL (or STEV). The short-term exposure limit (or value) is the highest permissible time-weighted average exposure for any 15-minute period during the work shift. It should be measured during the worst 15-minute period. The STEL is always higher than the TWA.
CL (or CEV). The Ceiling Limit (Ceiling Exposure Value) is the maximum permissible instantaneous exposure during any part of the work shift. Few chemicals are given both a STEL and a CL; the CL is usually reserved for highly dangerous substances.
For chemicals lacking either a STEL or CL, prudent values may be determined by multiplying the TWA by 3 for the STEL or by 5 for the CL, as is suggested by the Ontario (Canada) Ministry of Labor (1991). When more than one harmful substance is present, complex formulas must be used to determine combined occupational exposure limits. These formulas are prescribed by various governments and vary from country to country.
IDLH. This airborne concentration is immediately dangerous to life and health. Chemicals with low IDLH should be considered very dangerous when spilled or when significant volumes are being dispensed. A single inhalation at or above this limit could have serious, if not lethal consequences.
Types of hazard
Biohazards can be infectious agents themselves or items (solutions, specimens or objects) contaminated with them. Anything that can cause disease in humans, regardless of its source, is considered biohazardous, even if the disease primarily occurs in animals. In many countries, biohazardous materials are specially labeled and disposal is generally strictly controlled.
Irritants are chemicals that cause reversible inflammatory effects at the site of contact with living tissue. Most often, eyes, skin and respiratory passages are affected. Nearly all chemicals can be irritating given sufficient exposure to tissue, so general hygiene practices dictate that direct contact be avoided as much as possible.
Corrosive chemicals present both physical and health hazards. When exposed to living tissue, destruction or irreversible alteration occurs. In contact with certain inanimate surfaces (generally metal), corrosives destroy the material. A chemical may be corrosive to tissue but not to steel, or vice versa; few are corrosive to both.
Sensitizers cause allergic reactions in a substantial proportion of exposed subjects. Nearly any chemical may cause an allergic reaction in hypersensitive individuals, so the key here is the prevalence of the reaction in the exposed population. True sensitizers are serious hazards, because sensitization lasts for life and only gets worse with subsequent exposure. It may occur at work because of the high exposure level, but chances are the chemicals will also be found outside the workplace in lower concentrations that aggravate the allergy. Formaldehyde is a prime example. Its vapors come off permanent press clothing, draperies, upholstery, wall coverings, plywood and many other building materials.
Carcinogens: While many substances induce tumors in experimental animals exposed to unrealistically high dosages, officially recognized carcinogens must present a special risk to humans. Criteria for the carcinogenic designation differ slightly among agencies, but in the end, any carcinogenic chemical used in histology is universally recognized as such. Examples include chloroform, chromic acid, dioxane, formaldehyde, nickel chloride, and potassium dichromate. Additionally, a number of dyes are carcinogens: auramine O (CI 41000), basic fuchsin (pararosaniline hydrochloride, CI 42500), ponceau 2R (ponceau de xylidine, CI 16150) and any dye derived from benzidine (including Congo red, CI 22120; diaminobenzidine and Chlorazol black E, CI 30235).
Flammable materials have flash points below the specified temperature discussed above, and thus are of greater concern. Vapors should be controlled carefully to prevent buildup around electrical devices that spark. Special provisions for storage are usually mandated by national regulations, but local codes may impose even stricter measures. Storage rooms, cabinets and containers may have to be specially designed for flammable liquids; volumes stored therein may also be limited. Original manufacturers’ containers should be used whenever possible, and preferably should not exceed 1 gallon (4–5 liters).
Explosive chemicals are rare in histology, the primary example being picric acid. Certain silver solutions may become explosive upon aging; they should never be stored after use. In both cases, explosions may occur by shaking. Picric acid also forms dangerous salts with certain metals, which, unlike the parent compound, are potentially explosive even when wet. The best defense against explosive reagents is to avoid them altogether; this is certainly feasible today with picric acid.
Oxidizers initiate or promote combustion in other materials. Harmless by themselves, they may present a serious fire risk when in contact with suitable substances. Sodium iodate is a mild oxidizer that poses little risk under routine laboratory conditions. Mercuric oxide and chromic acid are oxidants that are more serious. Organic peroxides are particularly dangerous oxidizers sometimes used to polymerize plastic resins. Limit their volume on hand to extremely small quantities. Pyrophoric, unstable (reactive) and water-reactive substances are not generally found in histology. All involve fire or excessive heat.
Control of chemicals hazardous to health and the environment
Labeling
• chemical name and, if a mixture, names of all ingredients;
• manufacturer’s name and address if purchased commercially, or person making the reagent;
Many laboratories use small self-adhesive labels that say ‘Received: ——’. These are dated and affixed to each incoming container. Similarly, an expiration date should also be included for those chemicals that do not have an indefinite shelf life. Most inorganic compounds and many non-perishable organic chemicals are good for many years, but mixtures frequently deteriorate in a shorter time. Information on shelf life is hard to come by, and the best source is your own experience since each laboratory has different conditions and perhaps slightly varied formulations. Kiernan (1999) has included shelf-life data from his own extensive experience, which should serve as a good first approximation for your use.
Protective equipment
Gloves are the most controversial PPE, and misinformation abounds. It is important to understand how gloves work, so that informed decisions can be made about glove selection. Glove material is rarely completely impermeable; it delays penetration of harmful material for a time sufficient to provide adequate protection. Chemical resistance refers to how well material holds up in the presence of solvents, but says nothing about how readily substances move through the material. In most cases, liquids rarely penetrate intact glove material. The vapors are the problem, both because they penetrate more efficiently through gloves and skin, and because the worker usually cannot detect them. Reputable manufacturers of gloves evaluate their products in standardized tests, measuring the time it takes for detectable amounts of a particular chemical to appear on the far side of the material. This is called the breakthrough time, and it increases non-linearly with glove thickness. A glove twice as thick as another made from the same material will not have a breakthrough time that is double that of the thinner glove. Schwope et al. (1987) present the most comprehensive listing of data on this subject.
Ventilation
Ventilation is the foremost engineering control; ensuring proper airflow through a laboratory is the first critical step in improving working conditions. Every laboratory scientist should be aware of the following basic principles. For further details on hood design and placement, see Dapson and Dapson (2005) and Saunders (1993). Laboratories should have two separate systems of ventilation, one for general air circulation (often combined with heating and air conditioning and called HVAC), and the other for local removal of hazardous fumes. They must work in concert to be effective, and must not merely shift the noxious vapors to another part of the facility.
Air purification systems based upon ozone should not be used. They generate a chemical that is more hazardous than most of the fumes found in histology labs: ozone has a Ceiling Limit of 0.1 ppm according to ACGIH®. Further, ozone from these purifiers does not seem to be effective in destroying formaldehyde vapors (Esswein & Boeniger 1994).
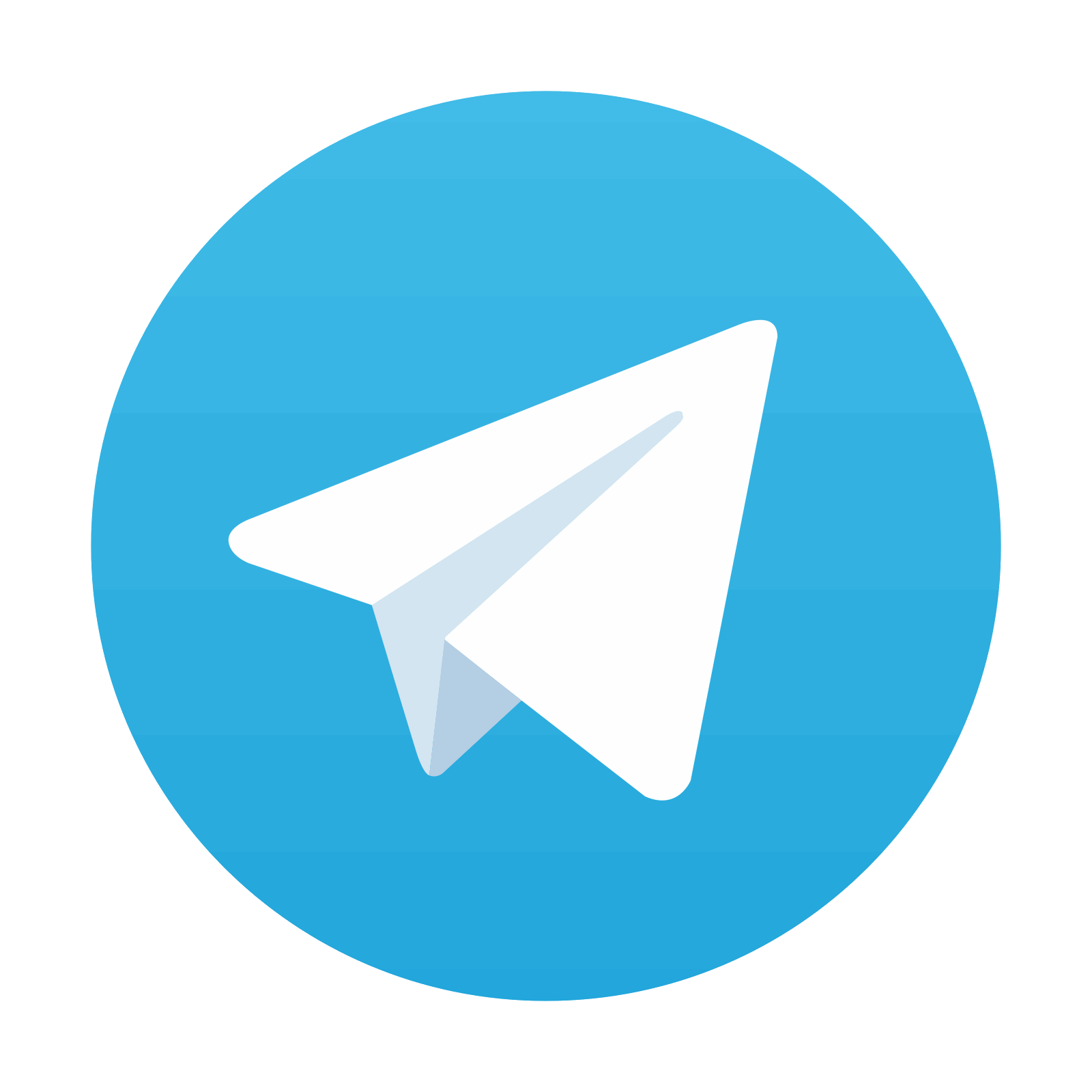
Stay updated, free articles. Join our Telegram channel

Full access? Get Clinical Tree
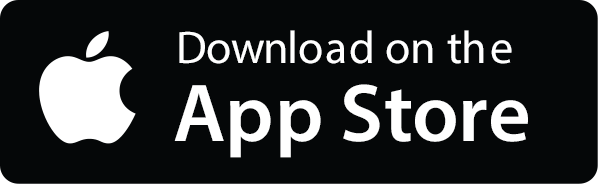
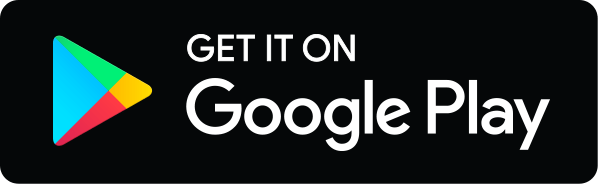