Safe Handling of Biological Agents in the Laboratory
Alan J. Beswick
Brian Crook
Catherine Makison Booth
Working with biological agents in the laboratory environment may involve the handling of microorganisms, cell cultures, or human endoparasites, including those that have been genetically modified, which may cause infection, allergy, toxicity, or other negative impact to human and/or animal health or the environment. Not all biological agents are hazardous by nature, but those that are harmful are often referred to as biohazards. When working in laboratories, the protection of test materials and sample integrity are important considerations, but these are secondary to the safety of personnel who may regularly be required to work with potentially infectious material. Risk assessment, management, and control are therefore important to laboratory biosafety to protect laboratory personnel and the wider community against inadvertent exposures and release of hazardous biological agents. This chapter has been prepared from authoritative information sources, including those from North America and Europe, in order to provide material that is internationally relevant for laboratory workers.
Four risk or hazard groups are often used to categorize biological agents based on several key factors associated with their inherent characteristics. These are
ability to cause disease
severity of disease
likelihood of spreading to the wider community
availability of prophylaxis or treatment
These groups are then often associated with an equivalent biosafety level (BSL; also known as containment level). Each BSL (ranging from levels 1 to 4) has a specific set of facility and operational requirements that must be complied with before work with the associated biological agents can be started. Most routine microbiological analytical work is undertaken in laboratories operated at BSL-2. Although biological agents handled at BSL-2 can cause disease, these typically present a low-to-moderate risk to employees and are unlikely to spread to the wider community because effective treatment or prophylaxis is available.1,2 Examples of these include common bacteria such as Staphylococcus aureus, Mycobacterium fortuitum, and Acinetobacter baumannii as well as viruses such as respiratory syncytial virus and norovirus. Fungal isolates such as Aspergillus fumigatus and Microsporum species also fall into this category. Many other examples exist and are listed by most national regulatory bodies as well as by international agencies.3,4,5,6,7
Work with biological agents may also be undertaken in facilities such as pharmaceutical production or with more hazardous pathogens in higher containment (BSL-3 or BSL-4) laboratories. Procedures and room design in these areas should reflect the nature of the activities undertaken. In some countries, regulations will specify the controls required, usually supported by codes of practice and guidance. These will typically allow for a broad range of clinical, diagnostic, and research work with biological agents.
In addition to identifying the hazards associated with the biological agents, other factors are important to ensure that work can take place within any laboratory setting safely and efficiently. These include effective health and safety management, including local risk assessment to identify what measures are needed to eliminate or control risk, implementation of these control measures, the competency of the staff to undertake the work, robust operational and safety procedures, and the fitness for purpose of the laboratory space itself (work surfaces, equipment, freedom of movement, appropriate waste disposal procedures, etc).

A hazard is something that has the potential to cause harm and a risk is the likelihood that somebody could be harmed by the hazard, together with an indication of how serious the harm could be. For example, in a microbiology laboratory, workers may be at risk of adverse health consequences from exposure to microorganisms. The risk to workers will increase
if the likelihood of exposure increases, which will depend on the activities being performed. The risk will also increase if the severity of the health consequences increases, which will be dependent on the type and number of microorganisms being handled. It is important to note that there may also be a risk of harm to people who do not work in the laboratory.
if the likelihood of exposure increases, which will depend on the activities being performed. The risk will also increase if the severity of the health consequences increases, which will be dependent on the type and number of microorganisms being handled. It is important to note that there may also be a risk of harm to people who do not work in the laboratory.
The aim of a risk assessment is to decide whether appropriate control measures are in place to eliminate or effectively control risks, or to determine whether more are required. The first step in assessing risk from biological agents in the laboratory is to identify and characterize the hazard(s) (ie, sources of harm), the nature of that harm, who might be affected, and how they may be harmed (ie, what they are doing and what could happen to cause harm).
Therefore, it is necessary to identify
What biological agents will be used
The activities or procedures to be undertaken, including whether sharps are used, and the potential for causing an exposure, for example, to generate aerosols or splash
The potential health consequences to both laboratory personnel and others who could be exposed
An estimation of risk can then be made. This will include consideration of both the severity of the hazard and the likelihood of harm, taking into account the control measures in place (or those planned for new activities). Having estimated the risk, the next stage of the risk assessment is to determine whether appropriate control measures are in place so that work can proceed or whether additional or different, more effective control measures are needed. Risk evaluation criteria will need to conform to national and local requirements.
Characterizing Biohazards
When making a biological risk assessment, the hazards relate directly to the biological agents to be handled. The risk associated with a biological agent will be dependent on several key factors including
the likelihood of infection if exposed
severity of disease (morbidity or mortality)
infectious dose
route(s) of transmission (natural versus laboratory; see in the following text)
communicability/R0 (basic reproduction rate—used to measure the transmission potential of a disease)
epidemiology (exotic or endemic)
effective prophylaxis and treatments (availability, practical, appropriate)
susceptibility of local population (naive)
Other factors that should be considered can include
environmental robustness (persistence outside of host/culture conditions) of the biological agent
natural host range (ie, coming across agents in laboratory not normally encountered), such as rabies being exotic in United Kingdom but endemic in mainland Europe and multi-drug-resistant tuberculosis (MDR TB) considered a greater risk pathology in laboratories at an inner city compared to a rural location
zoonosis
history of laboratory-acquired infection
susceptibility of staff working in the laboratory (immunocompromised)
symptoms (possibility of asymptomatic disease)
shedding (likelihood and type)
official control (is the agent on a national notifiable disease database, subject to human, plant, animal, or other regulations)
the concentration and volume of the biological agent to be handled
Routes of Transmission and Exposure
Biological agents usually have a certain route of entry into the body, although in some cases, infection can occur via multiple routes. Bacillus anthracis (the causative agent of anthrax), for example, is infectious via inhalation, percutaneous entry, or ingestion. It is important to note that natural routes of transmission and pathogenicity can differ from those associated with laboratory-acquired infection. This could be due to the use of high concentrations and high volumes of fluids, the generation of splashes and aerosols, use of sharps, and working with infected animals. In addition, the disease may also present itself differently if there has been an unconventional route of transmission. The nature of microorganisms being handled in the laboratory may vary, but one of the determining factors for an increased likelihood of microbiological exposure is when they are present at high titers as a result of their intensive propagation.
Using the inadvertent spillage of a bacterial culture as an example, the numbers of microorganisms involved will depend on the natural growth rate and age of the culture under laboratory growth conditions. A bacterial culture in late exponential growth typically has between 106 and 109 colony-forming units per millilitre (or milliliter) (CFUs)/mL,8 whereas cell concentration techniques may increase this to between 1010 and 1011 CFUs/mL. Viral particles can be similarly propagated and concentrated, for example, by filtration and centrifugation, to achieve high viral levels, usually measured as plaque-forming units per millilitre (or milliliter) (PFUs/mL) or ID50. Although less commonly used, fungal cultures for pathogen testing (eg, Coccidioides immitis9) produce stock suspensions of between 107 and 108 spores/mL. Therefore, there is the potential for significant exposure to microorganisms even when small volumes of cultures are being handled.
The main routes of infection in the laboratory are discussed in the following sections.
Percutaneous
Within the laboratory, routes of percutaneous transmission can include
sharps injury such as needles, broken glass, scalpel blades
splashing of the mucous membranes of the eye, nose, or mouth
contamination of unprotected skin lesions, for example, eczema
animal bites or scratches
presence of a vector, for example, biting or piercing insects
Blood-borne viruses (BBVs) are transmitted in contaminated blood or other body fluids following entry into the body of a susceptible person. The rate of viral transmission is dependent on the level of exposure to the virus, the type of virus, and the immune status of the exposed person. The BBVs are generally not thought to be transmitted via the respiratory route, although this possibility cannot be dismissed entirely if, under laboratory conditions such as in high-titer in vitro cultures, BBVs are present in concentrations far exceeding that found in normal body fluids. In the laboratory setting, BBVs are mainly transmitted by direct exposure to infected blood or other body fluids contaminated with infected blood, most likely via sharps injury.
Because most microbiology laboratory work involves handling cultures in aqueous suspension, splashing onto the mucous membranes is a real and feasible route of exposure and potential transmission through laboratory incidents, resulting from loss of primary containment. Aerosols may be created by, for example, spills or dropped and broken flasks.10 The loss of primary containment in these situations is likely to be in the form of material derived from a splash (see in the following text) and subsequently transferred on to other surfaces by the hands of workers.11,12,13
Damage to the skin barrier such as cuts, abrasions, or lesions caused by ongoing dermatological conditions can leave a person generally more susceptible to percutaneous transmission of infection. Consequently, it is important that laboratory workers are aware of any skin conditions, including broken or punctured skin and allergies, prior to handling biological agents or potentially contaminated materials, and take any additional precautions necessary to avoid exposure.
Contact
Direct contact with microbiologically contaminated objects or surfaces (fomites) can readily result in
ingestion of microorganisms, typically after pick up and hand-to-mouth transfer
their contact with mucus membranes
contact with the skin (commonly through broken skin but can occur via contact with intact skin)
The transfer of microorganisms, or other hazardous substances, via surfaces and objects is a well-known exposure route and has been particularly well studied in the health care settings. In poorly controlled work environments, there is a risk of cross-contamination from hazardous materials on surfaces and inadvertent transfer, for example, via gloved or unprotected hands.12 Human factors are also a significant factor. Even in a laboratory setting where the risks of hand to face contact are stressed, those contacts may still be made. In a study of 93 laboratory workers at BSL-2, 67 (72%) touched their face at least once, with contact to the nose being most common (44.9% of contacts), followed by contact with the forehead (36.9%), cheek/chin (12.5%), mouth (4.0%), and eye (1.7%).14 Another potential contact exposure scenario is while cleaning up a spill, warranting additional protection. The prevention of worker exposure via contact is therefore dependent on the effective use of primary containment, good laboratory technique and, where required, the decontamination of affected surfaces and equipment. Any intervention in the laboratory setting is also likely to include a disinfection step. The diligence of the operator and appropriateness of any products used for cleaning and disinfection are therefore critical to effective worker protection from surface contamination.
Airborne
The airborne route of exposure is commonly implicated in occupational exposures to all types of hazards including chemicals, nonbiological particulates, and bioaerosols. It is also an exposure that can be readily controlled in the laboratory setting using good laboratory techniques and effective engineering solutions, especially ventilation controls. Failure to do this can result in inhalation of infectious aerosols, conjunctival exposure, inhalation of infectious aerosols followed by swallowing/ingestion (eg, with norovirus), and droplet exposure.
Pottage et al11 examined the potential for routine microbiological techniques, such as serial dilution and pipetting, to be transmission routes by the generation of bioaerosols or splashing. Test activities, undertaken by staff with a range of experience and training, showed that aerosol and splash contamination was produced by all users, even those highly trained and experienced but that a correctly operating biological safety cabinet (BSC) will contain these aerosols. More experienced operators did not necessarily generate fewer aerosols, but those trained to work at BSL-3 did, suggesting that good microbiological technique was important. When preparing serial dilutions, those not trained at BSL-3 generated significantly more aerosols on average by over seven times. The study also showed that splash contamination on gloves and surfaces could potentially be spread within and outside the laboratory, emphasizing the importance
of effective personal protective equipment (PPE) and hygiene controls.
of effective personal protective equipment (PPE) and hygiene controls.
Working with animals, both large and small, may involve handling pathogenic microorganisms, and the animal’s physical activity may be the source of infectious bioaerosols. The containment of contaminated materials, such as fecal or urine-contaminated bedding, may be challenging under such circumstances. One study used a mouse parvovirus (MPV) model to assess the potential transfer from rodent housing containment to surrounding surfaces.15 The chosen virus represented the possible cross-contamination routes for other harmful viruses. The study found little evidence of significant deposition of the virus onto transfer surfaces, such as gloves and work surfaces outside of contained rodent storage cages. Even the positive pressure in ventilated cages did not result in animal room floor contamination, a suspected bioaerosol route of transfer, despite exhaust air escaping from the cage-top lip prior to high-efficiency particulate air (HEPA) filtration. However, soiled animal bedding and its handling by laboratory personnel was implicated in bioaerosol generation.
Infectious Dose and Environmental Robustness
The ability of a pathogen to cause infection requires its contact with susceptible host receptors (a combination of routes of transmission and exposure, as mentioned earlier) in a sufficient quantity, that is, at an infectious dose. Data on infectious dose for the wide range of human pathogens are limited. However, some notable examples illustrate the importance of taking this into consideration. Escherichia coli is an ubiquitous bacterium. Most E coli strains can cause minor infection via the oral route (hand-to-mouth transfer) but require a high dose to do so; therefore, it is safe to work with them in a general-purpose microbiology laboratory (BSL-2) using routine laboratory precautions. Disabled strains are commonly used tools in laboratory work, especially in genetic and molecular biology modifications and because of this attenuation, they are of minimal hazard (equivalent of BSL-1). However, verocytotoxigenic strains of E coli, such as O157, are zoonotic agents that can cause severe infection in humans at a very low infectious dose, estimated at just tens or hundreds of cells.16,17 This E coli serotype therefore requires laboratory handling using enhanced precautions (equivalent to BSL-3) that minimize exposure.
Where data on infectious dose are available, it is important to factor this into the biohazard assessment in developing a risk assessment. In addition to evidence from research papers, public and occupational health Web sites also provide a valuable source of data on infectious dose of human pathogens (eg, European Centre for Disease Prevention and Control,18 World Organisation for Animal Health,19 Public Health Agency of Canada,20 Health and Safety Executive,21 Public Health England,22 Centers for Disease Control and Prevention,23 World Health Organization24). However, such data must be used with caution because it is typically based on infectious dose derived from natural routes of transmission. In the laboratory, much higher titers of pathogens may be handled in culture and laboratory activities and, as described in the following text, this may create different potential routes of transmission. For example, although some vector-borne pathogens are most likely to be transmitted in the laboratory when handling infected mosquitoes or ticks, percutaneous injury from contaminated sharps may mimic the vector-borne route. These laboratory-specific routes of transmission must be taken into consideration.
Environmental robustness, that is, persistence of a pathogen outside its host, or outside of ideal culture conditions, is a further factor in assessing biohazard. At the one extreme, some enveloped viruses are more sensitive to ambient humidity and pH and quickly lose viability on surfaces or in aerosols,25 whereas at the other extreme, spore-forming bacteria have been shown to survive in the wider environment for decades.26

Laboratory work covers a broad range of procedures. This section describes how these procedures translate into laboratory activities and how these activities correlate with the risk of exposure to biological agents. The inherent hazard is the natural level of hazard associated with the agent being handled. The work carried out using the agent, eg, amount, titre used or procedures undertaken, will in turn influence any handling risk associated with the hazard.
Procedures that are less likely to result in exposure to biological agents include those that use low volumes and at low titer, such as molecular testing and use of diagnostics kits. For example, polymerase chain reaction (PCR) assays are unlikely to lead to significant exposure because of the preliminary steps in the process to lyse cells and extract nucleic acid, thus eliminating viable pathogens. Procedures that confer an increased likelihood of exposure include those that introduce energy into handling microbial cultures, for example, aspirating and ejecting liquids with pipettes, vortex mixing, or centrifuging. It should be noted that the potential for exposure via vortex mixing or centrifugation can and should be reduced with the use of sealed vessels and rotors. Procedures that present the greatest likelihood of exposure are those associated with handling high volumes with high titers of infectious agents in less contained conditions, including infected human or animal tissues. Figure 59.1 illustrates the relationship between examples of different types of laboratory procedure and the related likelihood of exposure to the hazard (the infectious agent) associated with the material/activity.
Estimating the Risks
To estimate the risks associated with the handling of microorganisms, the following need to be considered: consequence of release, consequence of exposure, likelihood of exposure, what could go wrong, and how likely it would be to go wrong.
Consequence of Release
The consequence of release (loss of containment in the laboratory) may immediately impact the operator directly involved, coworkers in neighboring laboratories, or others in the immediate vicinity. If loss of containment spreads beyond the laboratory environment, it may have implications for the nearby community or the wider environment (including farmed, domestic, or wild animal populations). As an example, foot-and-mouth disease (FMD) virus causes minimal harm to humans but seriously affects some farm animals. A nationwide outbreak of FMD in 2001 was estimated to cost the UK economy £8 billion, and another outbreak in 2007, although limited to eight farms, was estimated to cost the government department responding to it an estimated £47 million and the British livestock industry £100 million.27 The overall impact, in terms of health and economics, will be dependent on the key factors associated with the biological agent, such as infectious dose and method of dissemination. It is important to note that the likelihood of acquiring infection in the event of exposure may differ between laboratory personnel and the community, for example, laboratory staff might have been vaccinated lowering their risk of infection.
Considerations in determining the consequences if the biological agent was released can include
impact/consequence
environmental survival
natural distribution (of the agent in the country)
novelty (new or emerging biological agent, eg, pandemic influenza)
country preparedness (public health context)
epidemiology (endemic, exotic)
In cases where the consequences of the release can be significant, the characteristics of the biological agent (as previously noted) that may also be important to consider can include the overall impact to populations (eg, FMD on livestock), survival for long periods in the environment (eg, B anthracis), being a new or emerging pathogen, exotic epidemiology (nonendemic), and minimal country preparedness.
Consequence of Exposure
Several countries collect information on the nature of laboratory-acquired incidents (LAIs) and any reported operator infection as well as the wider impact. In addition, the American Biological Safety Association (ABSA) has developed a database of reported LAIs.28 The main exposure risks associated with working in this environment are therefore well identified, with some of these reports extending back many years. Some key examples are presented in the following text and provide an international insight into LAIs that help to put in perspective the biological agents implicated in these exposures and how they occurred. Most are based on human error, rather than failure of engineering controls.
The laboratory environment is no different from any other workplace in that there is the potential for incidents to occur, including due to human error. Where it does differ from many workplaces is the higher potential for those incidents to lead to exposure to infectious agents. The consequence of such exposures could be the potential for an LAI, or an actual infection, where the outcome could be fatal depending on the nature of the agent. From early in the development of laboratory-based work, incidents leading to serious infections and fatalities have underpinned the development and improvement of biosafety procedures. However, although improvements may have diminished such problems, they are not eradicated. Table 59.1 provides details of recent historical case reports of LAIs, the agents responsible, and underlying causes, providing useful evidence on the underlying causes of these exposures.
In addition to individual case reports and some surveys as described earlier, wider ranging summaries and surveys have previously been published but, with information dating back to the 1970s42 or the late 1990s,43 it is
questionable how relevant they are to current laboratory practices. However, Willemarck et al41 sourced 57 more recent surveys and reports and selected 47 for further review, with a total of 309 LAIs included. These are summarized in Table 59.2 and offer a useful focus on the known infectious microorganisms most associated with LAI.
questionable how relevant they are to current laboratory practices. However, Willemarck et al41 sourced 57 more recent surveys and reports and selected 47 for further review, with a total of 309 LAIs included. These are summarized in Table 59.2 and offer a useful focus on the known infectious microorganisms most associated with LAI.
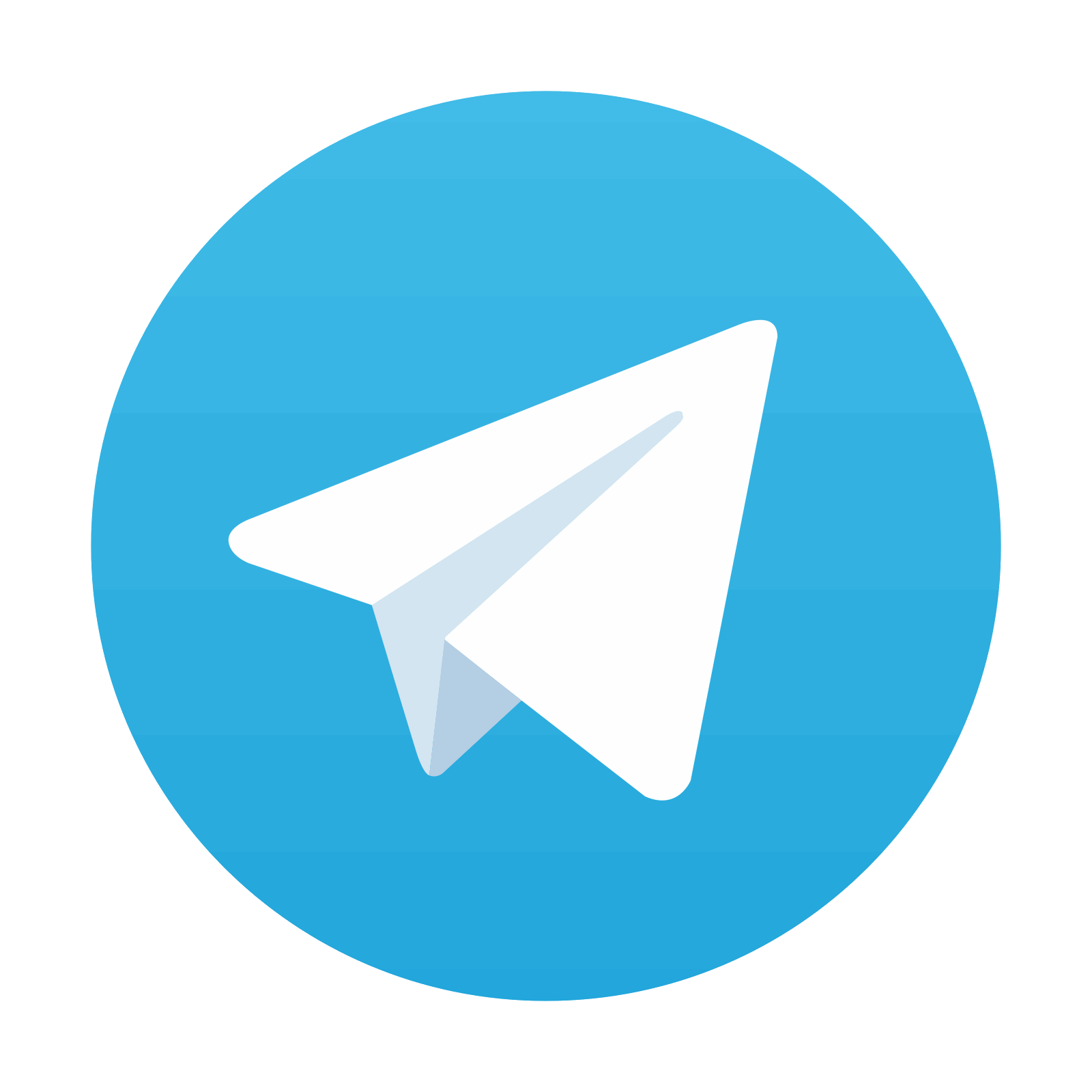
Stay updated, free articles. Join our Telegram channel

Full access? Get Clinical Tree
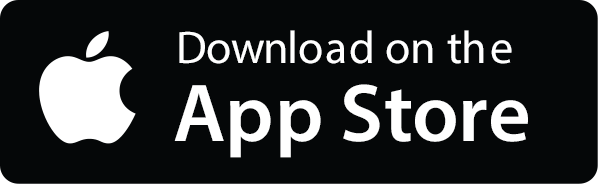
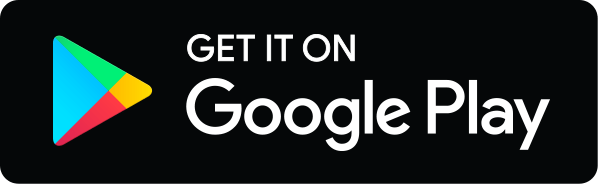