Fig. 37.1
Operative setup with the master–slave surgical robotic platform. The surgeon and control panel (master unit) are separate from patient and robotic arms (slave unit). This is the basic configuration of a surgical robot that enables telesurgery
Robotics and Remote Surgery: Expanding Boundaries
The idea to apply robotic technologies to surgery dates back to the 1970s when a military project of the National Aeronautics and Space Administration (NASA) aimed to provide surgical care to astronauts with remotely controlled robots and to replace the surgeon’s physical presence in situations of mass casualties in hostile environments such as war or natural catastrophes. The first generation of surgical robots that entered the OR was designed to perform image-guided precision tasks but was limited by basic computer interfaces. Of note is the PUMA 200 industrial robot used since 1985 for CT-guided brain biopsy [5] or the ultrasound-guided PROBOT, introduced in 1988 and used to perform prostate resections [6]. The first commercially available medical robot was manufactured in 1992 and was known as the ROBODOC (Integrated Surgical Systems, Sacramento, CA), which was approved to guide the surgeon during hip prosthetic replacements. The evolution of surgical robots has led to a current generation of real-time telemanipulators where robotic effectors reproduce the surgeon’s hand motion in a “master–slave” configuration. In these units, the “master” control console, from which the surgeon operates, is physically separated from the “slave” unit, composed of the robotic arms performing surgery on the patient. Two FDA-approved surgical robots originated from the DARPA (Defense Advanced Research Project Administration)-funded projects: the da Vinci® Surgical System (Intuitive Surgical, Inc., Sunnyvale, CA) and the Zeus® system (Computer Motion, Goleta, CA). Intuitive Surgery absorbed Computer Motion in 2003, and the only robotic platform available on the market today is the da Vinci® System.
Robotic surgery has been used in a vast array of surgical procedures, and although clinical benefits are not yet clear, the ability to perform remote surgery is certainly unique to the robotic interface.
The data transmission speed is the primary difficulty with teleoperations, especially over large distances or in the presence of insufficient retransmission infrastructures. Early systems, in fact, required the surgeon to be in the same room as the patient. However, with the use of high-speed telecommunications, both telementoring and telemanipulation were attempted from remote locations [7, 8].
Telementoring is the possibility for expert surgeons to mentor local surgeons through telepresence [9]. Telementoring programs that offer rural hospitals the possibility to gain full benefit of specialist advice are being established worldwide. One early report from 1996 demonstrated the ability of a surgeon located in the same city to successfully mentor another surgeon as well as to manipulate an endoscopic camera [10]. Cubano et al. [7] reported the efficacy of telementoring laparoscopic hernia repairs to surgeons operating on the Maryland-USS Abraham Lincoln Aircraft Carrier. Further reports have shown that specialist surgical skills can be disseminated effectively through telementoring [11].
Network latency affects the surgical performance with a longer task completion time of a factor of 1.45 and 2.04 in the presence of delays of data transmission of 250 ms and 500 ms, respectively, when compared to no time delay [12]. However, operators may still perform surgical training with a low error rate even at delays of approximately 1,000 ms [13]. Initially, latency in data transmission limited telemanipulation to a distance of a few 100 km [14].
In September 2001, the first transatlantic surgical procedure (Operation Lindbergh) covering the distance between New York (United States) and Strasbourg (France) was performed by our team. This is considered to be the milestone of global telesurgery [15]. In the Lindbergh operation, a combination of high-speed fiber-optic connection was used with an average delay of 155 ms with advanced asynchronous transfer mode (ATM) along with the Zeus® telemanipulator.
Now that one expert surgeon can operate from across continents, what is the next step?
The ultimate application for robotic telesurgery is probably the one that was initially conceived by the NASA: to provide surgical care to astronauts during long-lasting, extreme distance space explorative missions in which self-sufficiency of the space crew to face surgical emergencies is mandatory.
The challenges that must be overcome to make this possible are still manifold: the ability to perform surgery in reduced gravity conditions, portable and light equipment, and, most importantly, the possibility of cosmic distance data transmission.
The feasibility of Zero-Gravity surgery has been demonstrated with a cyst removal on a human subject onboard the European Space Agency (ESA) Airbus A-300 Zero-G Aircraft. Weightlessness phases were achieved performing parabolic curves [16]. Similarly, experimental laparoscopic surgery on a pig model is feasible in weightlessness [17], with only minor disturbances due to lack of gravity.
In addition, intensive research in miniaturization of surgical telemanipulators is under way, and a number of prototypes have been built with the aim to extend the possibilities for telesurgery, offering a lighter and more practical platform. The Raven (University of Washington, BioRobotics Lab) is a portable 22 kg mass robotic tool with two articulated arms. It has been conceived for both open and MIES [18], and it integrates long-distance remote control links. Another prototype under evaluation is the M7. It is also a light and portable device, developed by Stanford Research International, equipped with two arms with 7 Degrees of Freedom (DOF), and which integrates haptic feedback [19]. The software of the M7 is suitable for teleoperations, and in September 2007, it was successfully tested in the NASA first Zero-Gravity robotic experience during parabolic flights [20].
Internet-based communication speed and quality are sufficient to practice telesurgery on planet Earth, with reasonable delays of around 400 ms, but it is not suitable for space missions. Telesurgery in extremely remote locations requires more advanced telecommunications [21, 22]. The ability to provide real-time interaction between the spacecraft and the ground is obviously inversely related to distance. Taking advantage of satellite-based transmissions, with signals propagating at light speed (300,000 km/s), approximately 1-s delay would be experienced for an Earth-Moon distance, which is still reasonable for simple remotely controlled procedures. For very large distances, as an example, for an average orbiting distance between the Earth and Mars (72 million km), the delay would be around 6 min, which means that remotely controlling real-time procedures would not be possible, neither would be telementoring. The limitations for effective telementoring are probably below a 60-s delay. Beyond this range, a trained surgeon should be onboard and able to perform “solo.”
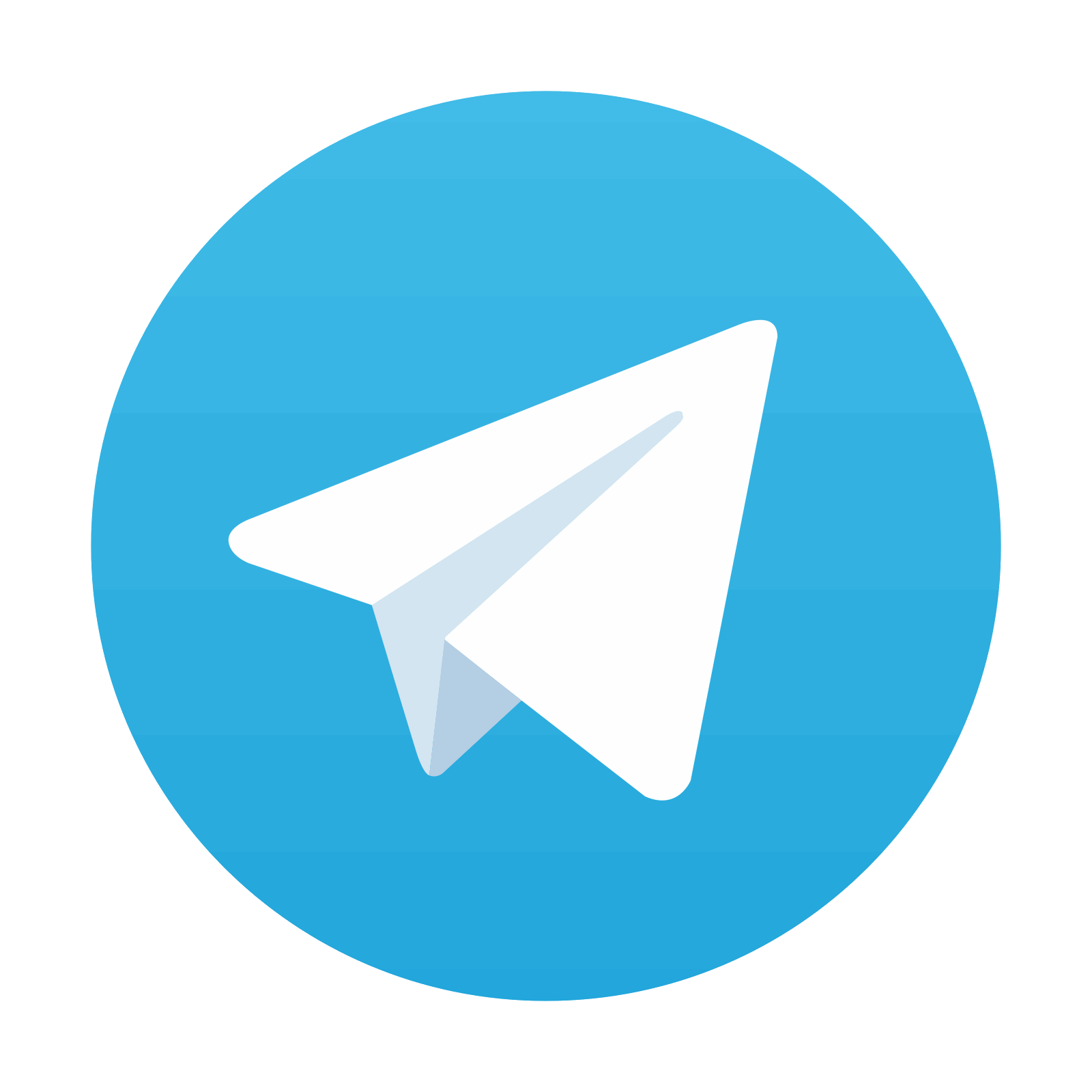
Stay updated, free articles. Join our Telegram channel

Full access? Get Clinical Tree
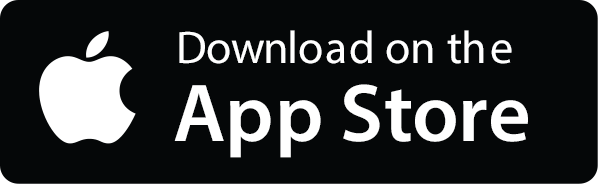
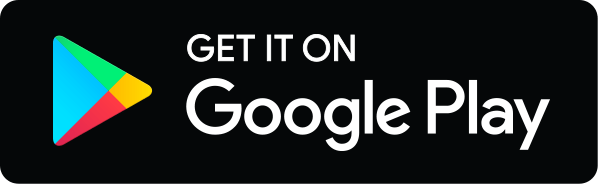