, amino acids and glucose, with an iso-osmotic amount of water. In the ascending limb of the loop of Henle, Cl– is pumped out into the interstitial fluid, generating the medullary hypertonicity on which the ability to excrete concentrated urine depends. This removal of Na+ and Cl– in the ascending limb results in the delivery to the distal convoluted tubule of hypotonic fluid containing only 10% of the filtered Na+ and 20% of the filtered water. The further reabsorption of Na+ in the distal convoluted tubule is under the control of aldosterone, and generates an electrochemical gradient which promotes the secretion of K+ and H+.
The collecting ducts receive the fluid from the distal convoluted tubules and pass through the hypertonic renal medulla. In the absence of vasopressin, the cells lining the ducts are impermeable to water, resulting in the excretion of dilute urine. Vasopressin stimulates the incorporation of aquaporins into the cell membranes. Water can then be passively reabsorbed under the influence of the osmotic gradient between the duct lumen and the interstitial fluid, and concentrated urine is excreted.
The endocrine functions of the kidney include the ability to synthesise hormones (e.g. renin, erythropoietin, calcitriol), to respond to them (e.g. aldosterone, parathyroid hormone (PTH)) and to inactivate or excrete them (e.g. insulin, glucagon). All of these functions may be affected by renal disease, with local or systemic consequences.
Many diseases affect renal function. In some, several functions are affected; in others, there is selective impairment of glomerular function or of one or more tubular functions. In this chapter, we discuss the use of chemical tests to investigate glomerular and tubular function. In general, chemical tests are mainly of value in detecting the presence of renal disease by its effects on renal function, and in assessing its progress. They are of less value in determining the causes of disease.
Tests of glomerular function
The GFR depends on the net pressure across the glomerular membrane, the physical nature of the membrane and its surface area, which in turn reflects the number of functioning glomeruli. All three factors may be modified by disease, but, in the absence of large changes in filtration pressure or in the structure of the glomerular membrane, the GFR provides a useful index of the numbers of functioning glomeruli. It gives an estimate of the degree of renal impairment by disease.
Accurate measurement of the GFR by clearance tests requires determination of the concentrations, in plasma and urine, of a substance that is filtered at the glomerulus, but which is neither reabsorbed nor secreted by the tubules; its concentration in plasma needs to remain constant throughout the period of urine collection. It is convenient if the substance is present endogenously, and important for it to be readily measured. Its clearance is given by
where U is the concentration in urine, V is the volume of urine produced per minute and P is the concentration in plasma. When performing this calculation manually, care should be taken to ensure consistency of units, especially for the plasma and urine concentrations.
Inulin (a complex plant carbohydrate) meets these criteria, apart from the fact that it is not an endogenous compound, but needs to be administered by IV infusion. This makes it completely impractical for routine clinical use, but it remains the original standard against which other measures of GFR are assessed.
Estimated GFR (eGFR) is now widely reported on laboratory report forms. This is a calculated estimate based on creatinine and a number of other variables. It gets around some of the problems associated with creatinine, described below, by incorporating age and sex in the calculation, but suffers from significant imprecision, and is not always applicable. It is further described on p. 56.
Measurement of creatinine clearance
Creatine is synthesised in the liver, kidneys and pancreas, and is transported to its sites of usage, principally muscle and brain. About 1–2% of the total muscle creatine pool is converted daily to creatinine through the spontaneous, non-enzymatic loss of water. Creatinine is an end-product of nitrogen metabolism, and as such undergoes no further metabolism, but is excreted in the urine. Creatinine production reflects the body’s total muscle mass.
Creatinine meets some of the criteria mentioned above. Creatinine in the plasma is filtered freely at the glomerulus, but its concentration may not remain constant over the period of urine collection. A small amount of this filtered creatinine undergoes tubular reabsorption. A larger amount, up to 10% of urinary creatinine, is actively secreted into the urine by the tubules. Its measurement in plasma is subject to analytical overestimation. In practice, the effects of tubular secretion and analytical overestimation tend to cancel each other out at normal levels of GFR, and creatinine clearance is a fair approximation to the GFR. As the GFR falls, however, creatinine clearance progressively overestimates the true GFR.
Estimation of creatinine clearance
A number of formulae exist for predicting creatinine clearance (or GFR) from plasma [creatinine] and other readily available information, such as age, sex and weight. The best known of these is that of Cockcroft and Gault (1976):
(creatinine clearance in ml/min, age in years, weight in kg, [creatinine] in μimol/L).
This equation has been shown to be as reliable an estimate of creatinine clearance as its actual measurement, since it avoids the inaccuracies inherent in timed urine collections. However, since it estimates creatinine clearance (not GFR), it suffers from the same overestimation of GFR as creatinine clearance when renal function declines. This calculation should not be used when serum [creatinine] is changing rapidly, when the diet is unusual (strict vegetarian diets, or creatine supplements), in extremes of muscle mass (malnutrition, muscle wasting, amputations) or in obesity.
The dosage of a number of potentially toxic chemotherapeutic agents is stratified by creatinine clearance calculated using the Cockcroft-Gault or a similar equation, so these calculations remain in use in pharmacy practice.
Plasma creatinine
If endogenous production of creatinine remains constant, the amount of it excreted in the urine each day becomes constant and the plasma [creatinine] will then be inversely proportional to creatinine clearance. The reference range of serum [creatinine] in adults is 55–120 μimol/L. However, individual subjects maintain their [creatinine] within much tighter limits than this. The consequence of this and the form of the relationship between [creatinine] and creatinine clearance is that a raised plasma [creatinine] is a good indicator of impaired renal function, but a normal [creatinine] does not necessarily indicate normal renal function (Figure 4.1). If a patient’s ‘personal’ reference range is low within the overall population reference range, [creatinine] may not be elevated until the GFR has fallen by as much as 50%. However, a progressive rise in serial creatinine measurements, even within the reference range, indicates declining renal function.
Figure 4.1 Relationship between plasma [creatinine] and creatinine clearance.
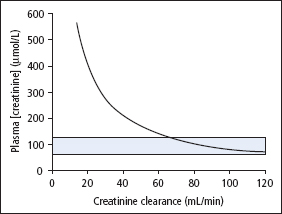
Creatinine clearance or plasma [creatinine]?
Measurement of plasma [creatinine] is more precise than creatinine clearance, as there are two extra sources of imprecision in clearance measurements, that is, timed measurement of urine volume and urine [creatinine]. Accuracy of urine collections is very dependent on patients’ co-operation and the care with which the procedure has been explained or supervised. The combination of these errors causes an imprecision (1 SD) in the creatinine clearance of about 10% under ideal conditions with ‘good’ collectors; this increases to 20–30% under less ideal conditions. This means that large changes in creatinine clearance may not reflect any real change in renal function.
It will be apparent that creatinine clearance measurements are cumbersome and potentially unreliable. They have essentially been superseded by calculation of the eGFR (see p. 56).
Low plasma [creatinine]
A low [creatinine] is found in subjects with a small total muscle mass (Table 4.1). A low plasma [creatinine] may therefore be found in children, and values are, on average, normally lower in women than in men. Abnormally low values may be found in wasting diseases and starvation, and in patients treated with corticosteroids, due to their protein catabolic effect. Creatinine synthesis is increased in pregnancy, but this is more than offset by the combined effects of the retention of fluid and the physiological rise in GFR that occur in pregnancy, so plasma [creatinine] is usually low.
High plasma [creatinine]
Plasma [creatinine] tends to be higher in subjects with a large muscle mass (Table 4.1). Other nonrenal causes of increased plasma [creatinine] include the following:
- A high meat intake can cause a temporary increase.
- Transient, small increases may occur after vigorous exercise.
- Some analytical methods are not specific for creatinine. For example, plasma [creatinine] will be overestimated by some methods in the presence of high concentrations of acetoacetate or cephalosporin antibiotics.
- Some drugs (e.g. salicylates, cimetidine) compete with creatinine for its tubular transport mechanism, thereby reducing tubular secretion of creatinine and elevating plasma [creatinine].
If non-renal causes can be excluded, an increased plasma [creatinine] indicates a fall in GFR, which can be due to pre-renal, renal or post-renal causes, as follows:
Table 4.1 Causes of an abnormal plasma [creatinine]
Reduced plasma [creatinine] | |
Physiological | Pregnancy |
Pathological | Reduced muscle bulk (e.g. starvation wasting diseases, steroid therapy) |
Increased plasma [creatinine] | |
No pathological significance | High meat intake, strenuous exercise |
Drug effects (e.g. salicylates) | |
Analytical interference (e.g. due to cephalosporin antibiotics) | |
Pathological | Renal causes, i.e. any cause (acute or chronic) of a reduced GFR |
- Impaired renal perfusion (e.g. reduced blood pressure, fluid depletion, renal artery stenosis).
- Loss of functioning nephrons (e.g. acute and chronic glomerulonephritis).
- I ncreased pressure on the tubular side of the nephron (e.g. urinary tract obstruction due to prostatic enlargement).
Plasma urea
Urea is formed in the liver from ammonia released by deamination of amino acids. Over 75% of non-protein nitrogen is excreted as urea, mainly by the kidneys; small amounts are lost through the skin and the GI tract. Urea measurements are widely available, and have come to be accepted as giving a measure of renal function. However, as a test of renal function, plasma [urea] is inferior to plasma [creatinine], since 50% or more of urea filtered at the glomerulus is passively reabsorbed through the tubules, and this fraction increases if urine flow rate decreases, such as in dehydration. It is also more affected by diet than [creatinine].
Low plasma [urea]
Less urea is synthesised in the liver if there is reduced availability of amino acids for deamination, as in the case of starvation or malabsorption (Table 4.2). However, in extreme starvation, plasma [urea] may rise, as increased muscle protein breakdown then provides the major source of fuel. In patients with severe liver disease (usually chronic), urea synthesis may be impaired leading to a fall in plasma [urea].
Table 4.2 Causes of an abnormal plasma [urea]
Reduced plasma [urea] | Low protein diet, severe liver disease, water retention |
Increased plasma [urea] | |
Pre-renal causes | High protein diet, GI haemorrhage (‘meal‘ of blood) |
Any cause of increased protein catabolism (e.g. trauma, surgery, extreme starvation) | |
Any cause of impaired renal perfusion (e.g. ECF losses, cardiac failure, hypoproteinaemia) | |
Renal causes | Any cause (acute or chronic) of a reduced GFR |
Post-renal causes | Any cause of obstruction to urine outflow (e.g. benign prostatic hypertrophy, malignant stricture or obstruction, stone) |
Plasma [urea] may fall as a result of water retention associated with inappropriate vasopressin secretion or dilution of plasma with IV fluids.
High plasma [urea]
It is convenient to subdivide the causes of high plasma [urea] into pre-renal, renal and post-renal (Table 4.2).
- Pre-renal uraemia may develop whenever there is impaired renal perfusion, and is essentially the result of a physiological response to hypovolaemia or a drop in blood pressure. This causes renal vasoconstriction and a redistribution of blood such that there is a decrease in GFR, but preservation of tubular function. Stimulation of vasopressin secretion and of the renin–angiotensin–aldosterone system causes the excretion of small volumes of concentrated urine with a low Na content. This reduced urine flow in turn causes increased passive tubular reabsorption of urea. Thus shock, due to burns, haemorrhage or loss of water and electrolytes (e.g. severe diarrhoea), may lead to increased plasma [urea]. Renal blood flow also falls in congestive cardiac failure, and may be further reduced if such patients are treated with potent diuretics. If pre-renal uraemia is not treated adequately and promptly by restoring renal perfusion, it can progress to intrinsic renal failure.
- Increased production of urea in the liver occurs on high protein diets, or as a result of increased protein catabolism (e.g. due to trauma, major surgery, extreme starvation). It may also occur after haemorrhage into the upper GI tract, which gives rise to a ‘protein meal’ of blood.
- Plasma [urea] increases relatively more than plasma [creatinine] in pre-renal uraemia. This is because tubular reabsorption of urea is increased significantly in these patients, whereas relatively little reabsorption of creatinine occurs.
- Renal uraemia may be due to acute or chronic renal failure, with reduction in glomerular filtration. Plasma [urea] increases until a new steady state is reached at which urea production equals the amount excreted in the urine, or continues to rise in the face of near-total renal failure. Although frequently measured as a test of renal function, it is always important to remember that plasma [urea] may be increased for reasons other than intrinsic renal disease (pre-renal and post-renal uraemia).
- Post-renal uraemia occurs due to outflow obstruction, which may occur at different levels (i.e. in the ureter, bladder or urethra), due to various causes (e.g. renal stones, prostatism, genitourinary cancer). Back-pressure on the renal tubules enhances back-diffusion of urea, so that plasma [urea] rises disproportionately more than plasma [creatinine].
Impaired renal perfusion and urinary tract obstruction, each in itself possible causes of uraemia, may in turn cause damage to the kidney and thus renal uraemia.
Estimated GFR and chronic kidney disease
Chronic kidney disease (CKD) affects up to 10% of the population and is often asymptomatic until renal function is severely reduced. Furthermore, mild CKD is a significant risk factor for cardiovascular disease. Detection of asymptomatic progressive CKD could allow patients to be actively treated to preserve renal function and reduce cardiovascular risk. However, it is apparent that creatinine clearance can be a reasonable measure of GFR, but is cumbersome and imprecise, whereas [creatinine] gives an indication of GFR and is readily performed, but can be misleading. In particular, [creatinine] is influenced by muscle mass (and hence by sex and age) as well as by GFR. A small elderly woman could lose a large proportion of her GFR and still have a [creatinine] within the population reference range and less than that of a young more muscular man, so a ‘normal’ [creatinine] can be misleading in such a patient.
eGFR is an estimate of GFR from a formula developed from a large study of patients with renal impairment (the Modification of Diet in Renal Disease (MDRD) study). A number of equations were derived in this study, but the four-variable MDRD equation is widely used in clinical laboratories. This uses [creatinine], age, sex and ethnic origin (for African-Caribbean people the eGFR should be multiplied by 1.212). This equation is not valid in people less than 18 years old, in acute renal failure, when [creatinine] is changing rapidly, pregnancy, muscle wasting diseases, malnutrition or amputees. The eGFR suffers from significant imprecision, and as GFR increases the precision and accuracy of eGFR decreases. Most laboratories therefore report eGFR >60 mL/min/1.73 m2 as such, rather than as an exact number.
eGFR is the basis for the classification of CKD (Table 4.3). It should be emphasised that an eGFR >60 mL/min/1.73 m2 should be regarded as normal in the absence of any other indication of kidney disease. This can be structural, such as polycystic kidney disease, or a urine abnormality, such as proteinuria or haematuria.
Tests of tubular function
Specific disorders affecting the renal tubules may affect the ability to concentrate urine or to excrete an appropriately acidic urine, or may cause impaired reabsorption of amino acids, glucose, phosphate, etc. In some conditions, these defects occur singly; in others, multiple defects are present. Renal tubular disorders may be congenital or acquired, the congenital disorders all being very rare. Chemical investigations are needed for specific identification of these abnormalities and may include amino acid chromatography, or investigation of calcium and phosphate metabolism (Chapter 5), or an oral glucose tolerance test (OGTT) (p. 94). The functions tested most often are renal concentrating power and the ability to produce an acid urine.
Table 4.3 Classification of CKD
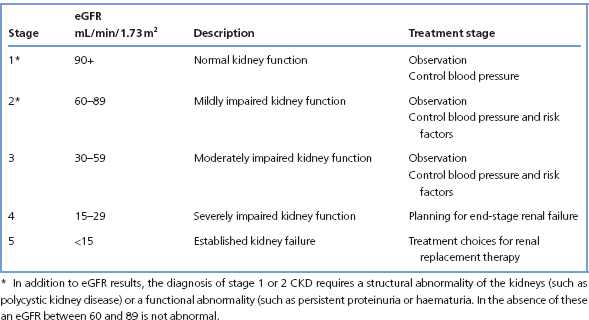
The healthy kidney has a considerable reserve capacity for reabsorbing water, and for excreting H+ and other ions, only exceeded under exceptional physiological loads. Moderate impairment of renal function may reduce this reserve, and this is revealed when loading tests are used to stress the kidney. Tubular function tests are only used when there is reason to suspect that a specific abnormality is present.
Urine osmolality and renal concentration tests
Urine osmolality varies widely in health, between 50 and 1250 mmol/kg, depending upon the body’s requirement to produce a maximally dilute or a maximally concentrated urine.
The failing kidney loses its capacity to concentrate urine at a relatively late stage. A patient with polyuria due to chronic renal failure is unable to produce either a dilute or a concentrated urine. Instead, urine osmolality is generally within 50 mmol/kg of the plasma osmolality (i.e. between about 240 and 350 mmol/kg). This has important implications. To excrete the obligatory daily solute load of about 600 mmol requires approximately 2 L of water at a maximum urine osmolality of 350 mmol/kg, compared with 500 mL of the most concentrated urine achieved by the normal kidney. Hence, patients with CKD require a daily water intake of at least 2 L to maintain their water balance. On the other hand, a large intake of water can lead to dangerous hyponatraemia, since water excretion is limited by the inability to produce a sufficiently dilute urine.
Urine osmolality is directly proportional to the osmotic work done by the kidney, and is a measure of concentrating power. Urine specific gravity, which can be estimated using urinalysis dipsticks, is usually directly proportional to osmolality, but gives spuriously high results if there is significant glycosuria or proteinuria.
Renal concentration tests are not normally required in patients with established chronic renal failure, and indeed may be dangerous. However, the tests may be indicated in patients with polyuria in whom common causes (e.g. diabetes mellitus) have first been excluded. In a number of conditions, the kidney loses its ability to maintain medullary hyperosmolality, and hence to excrete a concentrated urine, but these should have been excluded before renal concentration tests are performed. Causes of failure to concentrate urine are shown in Table 4.4.
In patients with polyuria, measurement of the osmolality of early morning urine specimens should be made before proceeding to formal concentration tests. If urinary osmolality greater than 800 mmol/kg is observed in any specimen, as should be the case in most patients who can concentrate urine normally, there is no need to perform further tests of concentrating ability.
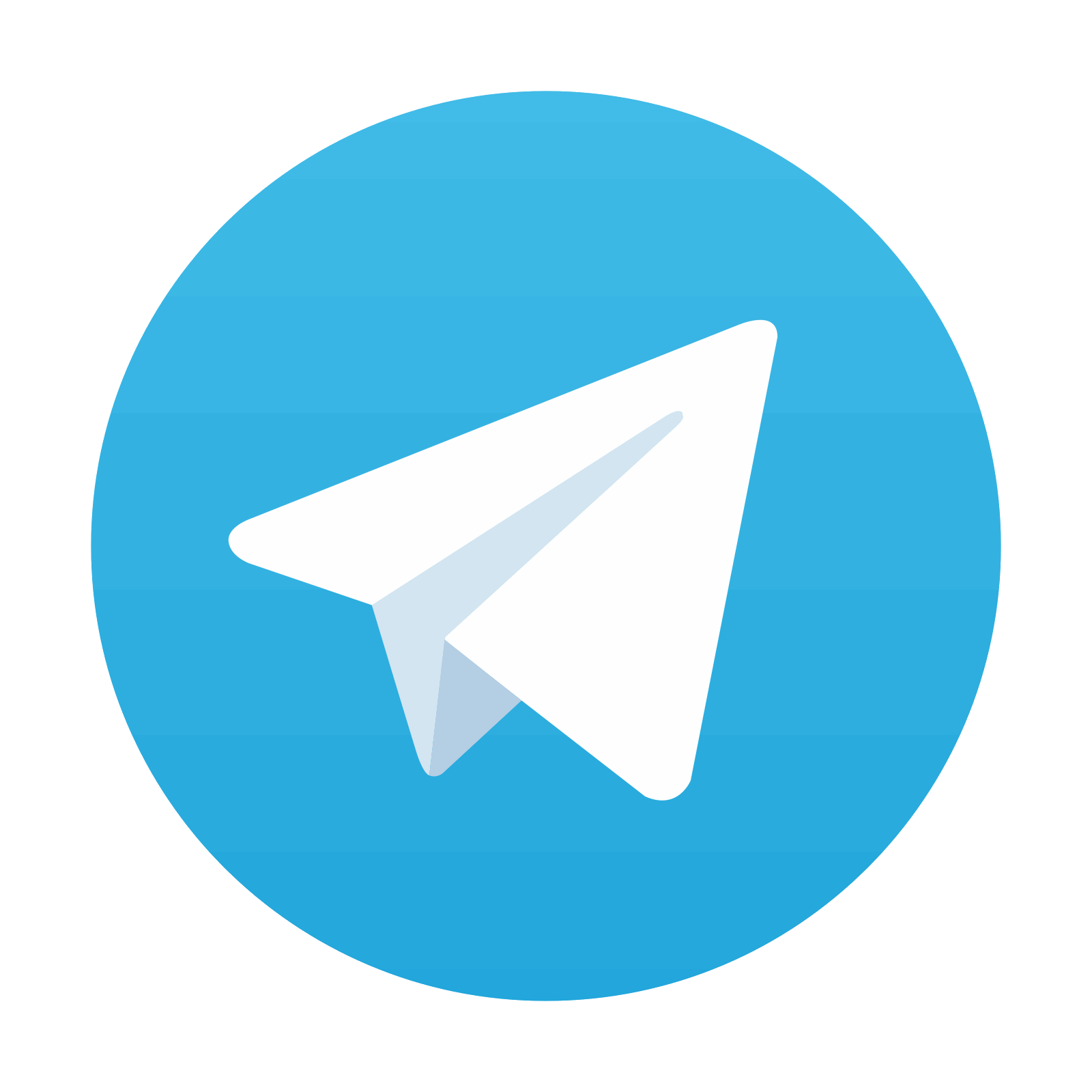
Stay updated, free articles. Join our Telegram channel

Full access? Get Clinical Tree
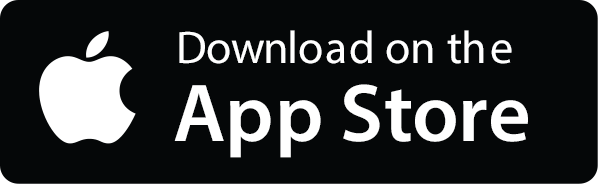
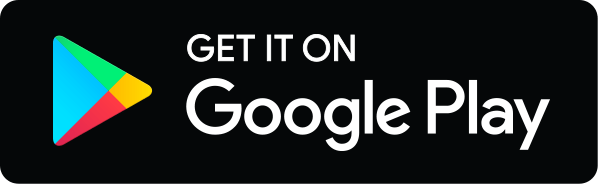