Fig. 9.1
Symmetric and asymmetric divisions (modified from http://stemcells.nih.gov/info/scireport/chapter1.asp)
9.3.3 Ability to Give Rise to Specialized Cell
The ability of stem cells to give rise to specialized cells is a crucial one. In this process of differentiation, unspecialized stem cells produce specialized cells. It is believed that genes regulate the internal signals that trigger this process. These genes carry the specific code or instructions for all the parts and functions of a cell. External signals are those outside of the cell, which include chemicals released from other cells, physical connections with nearby cells, and various other molecules in the surrounding area. Currently, scientists are searching for similarities and differences between the signals from one stem cell to another. Ultimately, answers will allow researchers to find ways to control stem cell differentiation. With control, scientists can more easily grow cells and tissues for specific functions.
9.3.4 Plasticity of Stem Cells
Plasticity is an important property of stem cells which allows these cells to be committed to generating a fixed range of progeny. When they have been relocated, to make other specialized sets of cells appropriate to their new niche, they can switch. The evidence for stem cell plasticity in rodents and man was explained by Poulsom et al. (2002).
Adult stem cells are surprisingly flexible in their differentiation repertoires due to the existence of different pathways. Adult stem cells typically generate the cell types of the tissue in which they reside. A blood-forming adult stem cell in the bone marrow, for example, normally gives rise to the many types of blood cells such as red blood cells, white blood cells, and platelets. Until recently, it had been thought that a blood-forming cell in the bone marrow (hematopoietic stem cell) could not give rise to the cells of a very different tissue, such as nerve cells in the brain. However, a number of experiments over the last several years have raised the possibility that stem cells from one tissue may give rise to cell types of a completely different tissue. This phenomenon is known as plasticity.
9.4 Classification
Stem cells can be classified according to their plasticity. Different types of stem cells vary in their degree of plasticity or developmental versatility. The categories into which they fall include: the totipotent stem cell, pluripotent stem cell, multipotent stem cell, and oligopotent and nullipotent stem cells (Fig. 9.2).
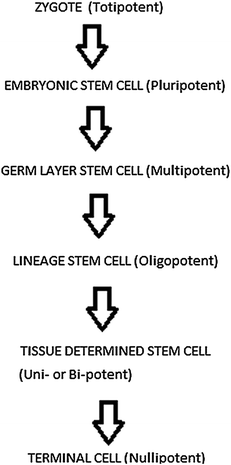
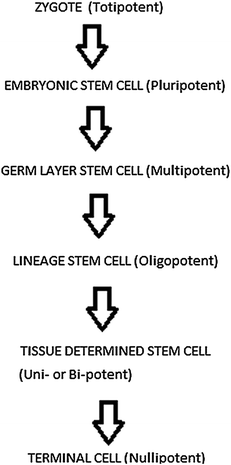
Fig. 9.2
Hierarchy of stem cells during differentiation showing that differential potential decreases and specialization increases at each stage of development
9.4.1 Totipotent Stem Cells
“Toti” comes from the Latin word meaning “whole” or “Total”, so totipotent stem cells have total potential to develop into any cell type or organ/tissues including extra embryonic tissues. Totipotent stem cells develop during sexual reproduction when male and female gametes fuse during fertilization to form a zygote. The zygote is totipotent because its cells can become any type of cell and they have limitless replicative abilities. After fertilization, zygote divides into identical totipotent cells, which can later develop into any of the three germ layers of a human, i.e., endoderm, mesoderm, or ectoderm. This stage is called morula stage (16-cell stage); the totipotent cells of morula start shifting toward pluripotent stem cells called blastocyst.
9.4.2 Pluripotent Stem Cells
Pluripotent stem cells are those cells which are able to form all the different types of cells in the body. Specialization in pluripotent stem cells is minimal, and therefore they can develop into almost any type of cell. Pluripotent cells show the formation of tumor-like mass called teratoma. Teratoma formation is a hallmark of true pluripotency. It results from the aggressive proliferation and differentiation of transplanted stem cells.
The best example of pluripotent stem cell is embryonic stem cell (ESC). ESCs seem to be more flexible compared to adult stem cells, because they have the potential to produce every cell type in the human body. ESCs are derived from inner cell mass, called blastocyst, of 5–6-days-old embryo. After the fertilization of an egg by a sperm, blastocyst which is a tiny and hollow sphere of 30–34 cells develops generally in 5 days that has potential to develop into a complete human being. In normal development, the blastocyst implants in the wall of the uterus to become embryo and then develops into a mature organism. Outer layer of cells initiates the formation of the placenta and the inner cell mass starts differentiating into the progressively more specialized cells of the body. During the early stages of development, ESCs present in specific part of embryo may develop into eye, blood, muscle, nerve, liver cells, etc. When ESCs which may become liver, are isolated and transferred to another section of the embryo, can be developed into eye or blood. This shows that differentiation of stem cell depends on their position in embryo.
ESCs can be induced to replicate in an undifferentiated state for very long periods of time and then produce specialized cells only after differentiation, which can be used for therapeutic and transplantation purposes. However, undifferentiated ESCs can form a teratoma and therefore, could not be used directly for tissue transplants. In an experiment, hESCs have been successfully differentiated into tissues derived from the three germ layers (Cai et al. 2007). This proves that hESCs can differentiate into a broad spectrum of cell types in culture.
The differentiation of hESCs is efficient and has advantages and disadvantages. The main advantages of hESCs are that these cells can develop into most of the cells/tissues of the body and are easy to cultivate in laboratory. These cells have great potential for developing future therapies to cure diseases. The major disadvantage of hESCs is that blastocyst must be destroyed when cells are removed. Moreover, egg donation is an important and serious issue which creates ethical problems.
Recently, iPSCs are developed which are genetically altered adult stem cells. These cells are induced or prompted in a laboratory to take the characteristics of ESCs. Although iPS cells behave like ESCs and express some of the same genes that are expressed normally in ESCs, they are not exact duplicates of ESCs.
9.4.3 Multipotent Stem Cells
Multipotent stem cells have the ability to differentiate into a limited number of specialized cell types. Multipotent stem cells typically develop into any cell of a particular group or type. For example, bone marrow stem cells can produce any type of blood cell. However, bone marrow cells do not produce heart cells. Mesenchymal stem cells (MSCs) are multipotent cells of bone marrow that have the ability to differentiate into several types of specialized cells related to them. These stem cells give rise to cells that form specialized connective tissues, as well as cells that support the formation of blood. Fetal stem cells, adult stem cells, and umbilical cord stem cells are examples of multipotent cells.
Fetal stem cell is the main site of hematopoiesis and is rich source of hematopoietic stem cells (HSCs) that leads to the generation of multiple cell types in blood. Tissues extracted from the fetal pancreas, when transplanted into diabetic mice, are reported to stimulate insulin production. The possible reason of this is due to a true stem cell, a more mature progenitor cell, or to the presence of fully mature insulin-producing pancreatic islet cells themselves.
Primordial germ cells are multipotent cells that have been isolated from the gonadal ridge, a structure that arises at an early stage of the fetus that will eventually develop into eggs or sperms in the adult. Germ cells can be cultured in vivo and have been shown to form multiple cell types of the three embryonic tissue layers.
Adult stem cells or somatic stem cells are undifferentiated cells, found throughout the body after embryonic development. These cells multiply by cell division to replenish dying cells and regenerate damaged tissues. Adult stem cells are present in the depth of organs along with millions of ordinary cells and restock some of the body’s cells. These stem cells are tissue specific in their location and are derived from adult tissues such as bone marrow, blood, eye, brain, skeletal muscle, dental pulp, liver, skin, and the lining of the gastrointestinal tract and pancreas. Usually, they have very limited power of division due to which these can develop into the cells of only one particular tissue, therefore considered as multipotent stem cells. In some tissues, these cells sustain turnover and repair throughout life. For example, stem cells of skin will give rise to new skin cells and those obtained from the brain can differentiate into blood cells and muscle tissue.
Adult stem cells are not only difficult to identify and purify, but also difficult to maintain in the undifferentiated state during growth in culture medium. Culturing of adult stems cells in a definitive medium is a high priority of stem cell research. The one important feature of stem cell is that these stem cells can be manipulated easily and effectively.
An adult stem cell is a completely committed stem cell. This concept is one of the exciting discoveries of last millennium. There is now evidence that some of blood stem cells apparently are committed adult stem cells that are able to become a stem cell in a different organ. Committed blood forming stem cell can produce different cells performing different functions. There are experiments performed for bone marrow transplantation in rats with damaged livers which help the liver to re-grow partially with bone marrow-derived stem cells. Bone marrow-derived stem cells in muscle connective tissue, satellite cells in adults, and stem cell marker expressing cells located in muscle connective tissue can be derived from bone marrow in adulthood (Dreyfus et al. 2004).
9.4.4 Oligopotent Stem Cells
These stem cells have the ability to differentiate into just a few types of cells. A lymphoid stem cell is an example of an oligopotent stem cell. This type of stem cell cannot develop into any type of blood cell as bone marrow stem cells can do. They only give rise to blood cells of the lymphatic system such as T and B cells.
9.4.5 Unipotent Stem Cells
These stem cells have unlimited reproductive capabilities, but can only differentiate into a single type of cell or tissue. Unipotent stem cells are derived from multipotent stem cells and formed in adult tissue. Skin cells are one of the most prolific examples of unipotent stem cells. These cells must readily undergo cell division to replace damaged cells.
9.5 Stem Cell Technology
A stem cell has the potential to develop into all kinds of body cells. This versatility of stem cells can be used as an important tool to solve many kinds of problems in biology and medicine and treatment of numerous diseases to save lives. With further research, scientists will hopefully be able to understand more about the intriguing properties of stem cells and can then find ways to isolate, cultivate, and manipulate stem cells for therapeutic value. This process is called stem cell technology (Fig. 9.3). Researches on stem cells provide advance knowledge about the development of organism from a single cell and replacement of damaged cells with healthy cells in adult organisms.
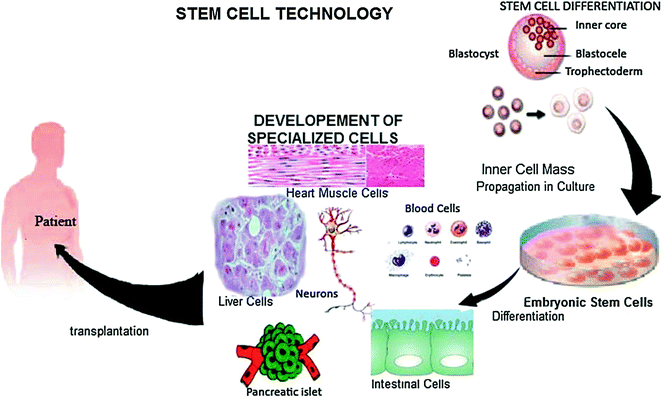
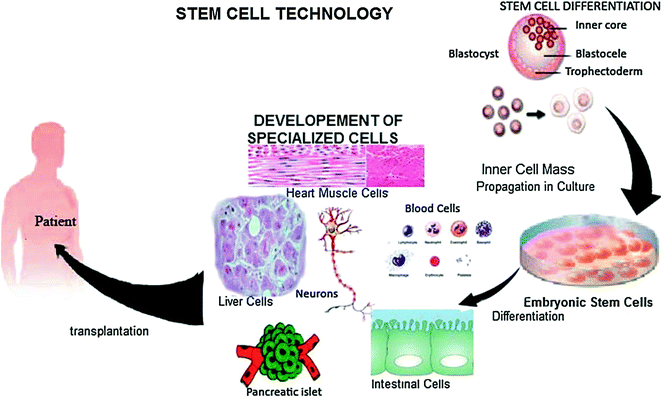
Fig. 9.3
Stem cell technology for replacing the damaged cells of human body (modified from http://www.stemcellsforhope.com)
9.5.1 Sources of Stem Cell
Stem cells can be isolated from different sources. These can be efficiently obtained from immature tissues possessing undifferentiated cells such as embryonic cells (Fig. 9.4). Different populations of stem cells exist in embryo and are arranged hierarchically. This arrangement makes the isolation of stem cells easy, different ESCs can thus be isolated from different stages of developing organism such as morula, blastocyst, placenta, amniotic fluid, organ primordia, and umbilical cord blood. The stem cells are also present in the adult organisms revealing the nondisappearing of adult cells after birth. However, these cells dilute among differentiated cells and also change their properties in response to the change of niche and new demands of the organism. Tissue-specific stem cells can be harvested from different tissues and at different time-points: from children tissues, adult tissues, tissues of elderly people, and even postmortem.
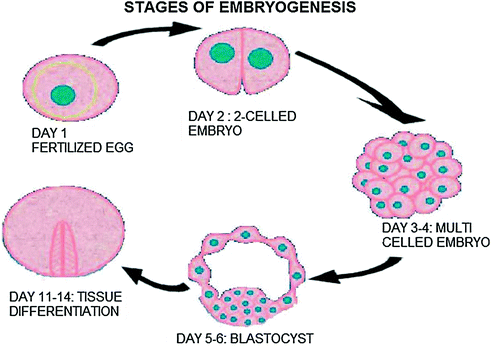
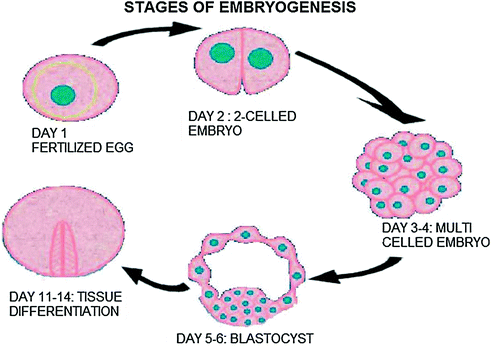
Fig. 9.4
Different stages of embryo development as a possible source of stem cells (modified from http://www.godandscience.org/slideshow/stem006.html)
9.5.1.1 Embryonic Stem Cell Sources
(a)
In vitro fertilization
The main potential source of blastocysts for stem cell research is IVF clinics. On administrating fertility drugs, stimulated ovaries start producing multiple mature eggs. These eggs are retrieved by surgical procedure and all donated eggs are fertilized by sperms in order to increase their chance of producing a viable blastocyst that can be used for implantation in the womb. However, all blastocysts are not implanted; remaining can be stored in freezers which can be a major source of ESCs for use in medical research.
IVF facilitates the formation of blastocysts by fusion of sperm (specific genetic traits) with eggs of donor. This will help us in the study of particular diseases. However, the creation of stem cells/blastocysts specifically for research using IVF is ethically problematic for some people, because it involves intentionally creating a blastocyst that will never develop into a human being.
(b)
Nuclear transfer
Another potential way to produce ESCs is nuclear transfer. Nuclear transfer can be done by inserting the nucleus of an already differentiated adult cell into a donated enucleated egg. Due to insertion of nucleus, enucleated egg becomes nucleated which is then stimulated to form a blastocyst. This blastocyst is a source of ESC which are copies or clones of adult cell due to the presence of nucleus of adult stem cell. The ESCs created by nuclear transfer are genetically matched to the person needing a transplant, therefore shows the less chance of rejection by immune system.
There is a misconception that nuclear transfer technique is similar to reproductive cloning. The use of nuclear transfer to develop disease-specific stem cells can be called research cloning, and the use of this technique for tissue transplants is called therapeutic cloning. These terms are distinguished from reproductive cloning in which the intent is to implant a cloned embryo in a female’s womb and allow it to develop fully into an individual. This was the technique by which “Dolly” the sheep was made and is now widely used for reproductive cloning in animals. In humans, however, reproductive cloning has been discouraged by most of researchers.
Unfortunately, nuclear transfer has not been successful in the production of hESCs. Scientists may use this technique to develop human stem cells in the future for studying the development and progression of specific diseases. This method may be useful in creating the stem cells that contain only DNA of a patient suffering from a specific disease.
(c)
Cord blood and aborted fetus
Recent researches have shown that ESCs can be obtained from cord blood which may increase chances of the acceptance of stem cell research. Besides cord blood, stem cells can be harvested from aborted fetus.
(d)
Amniotic fluid
In the womb, fetus is bathed with amniotic fluid which contains fetal cells including MSCs possessing ability to make a variety of tissues. Many pregnant women elect to have amniotic fluid drawn to test for chromosome defects, the procedure known as amniocentesis. This fluid is normally discarded after testing, but Dr Dario Fauza, a surgeon at Children’s Hospital, Boston and a member of the stem cell program, have been investigating the idea of isolating MSCs and using them to grow new tissues for babies who have birth defects detected while they are still in the womb, such as congenital diaphragmatic hernia. These tissues would match the baby genetically, hence face less chances of rejection by the immune system, and can be easily implanted either in utero or after the baby is born.
9.5.1.2 Adult Stem Cell Sources
1.
Manipulating differentiated cells
Specialized adult human cells can be reprogrammed genetically by introducing specific genes to create iPSCs (stem cell-like state). These iPSCs can be differentiated to any cell type.
2.
Somatic cells
Stem cells are thought to reside as quiescent (nondividing) cells in a specific area of each tissue called a “stem cell niche”. These cells are also called somatic stem cells and begin to divide and create new cells only when they are activated by tissue injury, disease, or anything else that makes the body need more cells. Many tissues have been reported to possess somatic stem cells such as bone marrow, blood vessels, and skeletal muscles.
3.
Other adult stem cell sources
Many researchers have reported the presence of certain kind of adult stem cells which can transform, or differentiate, into apparently unrelated cell types. This phenomenon is called transdifferentiation and has been reported in some animals. Differentiation of brain stem cells into blood cells and blood forming cells into cardiac muscle cells is the best example of transdifferentiation process. Many questions are unanswered with this process such as how versatile they are, whether this is present in human stem cells, can these be synthesized reliably in laboratory. Stem cells are also present in tumors which are responsible for the growth of cancers. Hence, stem cells can be isolated from tumor which is removed from the body by surgical procedure.
Unlike ESCs, the use of adult stem cells in research and therapy is not controversial, because the production of adult stem cells does not require the creation or destruction of an embryo. However, the demand for autologous, patient-specific stem cells for regenerative therapies outstrip their supply. Several types of adult stem cells have been described which are given below:
(a)
Hematopoietic stem cells
Blood stem cells have the ability to regenerate all types of blood cells; therefore can recreate an entire new blood system. Stem cells derived from bone marrow, when transplanted by intravenous injection to patients produce an entirely new blood system which helps the patients in recovering from cancer. There is a room for research that how and why stem cells find their way to the bone marrow site and regenerate a new blood system.
Recently, clinicians and scientists have focused on CXCR4 as the protein that provides the “navigation system” to allow human blood stem cells to find their way to the bone marrow and regenerate blood. The positive expression for CXCR4 has been widely hailed as a definitive marker for blood stem cells. Two distinct subgroups of blood stem cells called CXCR4-positive and CXCR4-negative have been identified. Their investigations showed that both subgroups possess the same blood cell regeneration capabilities but show other unique functional and physiological features including how they respond to growth factors. The two subgroups also preferentially differentiate into different types of blood cells: CXCR4-positive to disease fighting immune cells and CXCR4-negative to red blood cells (http://www.robarts.ca/discovery-new-stem-cell-properties-advance).
HSCs are very rare, hence, their isolation and tracking in patients is difficult. Blood stem cells are present in bone marrow stromal stem cells (BMSCs) that have become a standard in the field of adult stem cell biology and in regenerative medicine due to their high differentiation potentials and low morbidity during harvesting. Nevertheless, harvesting of BMSCs by bone marrow aspiration is a painful procedure and the number of cells acquired is usually low.
(b)
Mammary stem cells (MaSCs)
MaSCs provide cells for growth of the mammary gland during puberty and gestation and play an important role in carcinogenesis of the breast. The mammary gland is a dynamic organ undergoing significant developmental changes during puberty, pregnancy, lactation, and involution. Numerous studies have provided strong evidence for the existence of MaSCs based on the fact that the mammary gland can be regenerated by transplantation of epithelial fragments in mice. Shackleton et al. (2006) isolated discrete populations of mouse mammary cells on the basis of cell-surface markers and identified a subpopulation (Lin-CD29hiCD24+) that is highly enriched for MaSCs. MaSCs have been isolated from human- and mouse- tissue as well as from cell lines derived from the mammary gland.
These stem cells capable of self-renewal and differentiation into the basal and luminal lineages comprise the functional mammary epithelium and have the ability to regenerate the entire organ in mice. The self-renewing capacity of these cells was demonstrated by serial transplantation of clonal outgrowths.
Recently in 2010, it was reported that MaSCs are highly responsive to steroid hormone signaling. However, they lack estrogen and progesterone receptors. Ovariectomy markedly diminished MaSC number and outgrowth potential in vivo, whereas MaSC activity increased in mice treated with estrogen plus progesterone (Labat et al. 2010).
(c)
Mesenchymal stem cell
Mesenchymal stem cells (MSCs) are of stromal origin and may differentiate into a variety of tissues/cells including chondrocytes, which produce cartilage. MSCs have been isolated from placenta, adipose tissue, lung, bone marrow and blood, Wharton’s jelly from the umbilical cord and teeth (perivascular niche of dental pulp and periodontal ligament).
MSCs cultured on regular flat surfaces such as petridishes, rapidly lose their multipotent properties which render them useless for stem cell experiments or clinical use. This problem is solved by using nanopatterned surfaces for the culturing of MSCs which helps in maintaining their phenotypic characteristics such as multipotency over long culture periods (McMurray et al. 2011). Generally, MSCs cultured with osteogenic media have very great efficiency of producing bone mineral in vitro. Similar efficiency can be achieved by the use of nanoscale disorder to stimulate human MSCs even in the absence of osteogenic supplements. However, it was reported that topographically treated MSCs have a distinct differentiation profile compared with those treated with osteogenic media, which has implications for cell therapies. This shows that stem cell differentiation to become osteoblasts occurs in the absence of chemical treatment without compromising material properties.
MSCs are involved in osteoblast differentiation. Metabolic acidosis can alter osteoblast differentiation from MSCs through its various effects on osteoblastic genes. Furthermore, osteoblastic genes produce different proteins which result in an impairment of bone formation.
Defect in MSCs can be corrected by genetic modification. After genetic modification, MSCs can be maintained in healthy and undifferentiated state. Furthermore, the genetically modified MSCs are able to engraft into many tissues of unconditioned transgenic mice making them an attractive therapeutic tool in a wide range of clinical applications. Chronic MSCs are also attractive for clinical therapy due to their ability to differentiate, provide trophic support, and modulate innate immune response. Researchers from Boston University’s School of Dental Medicine proved that bone marrow-derived MSCs can relieve orofacial pain in rats within one day of treatment by either intravenous injection or direct injection of cells to the injured area.
(d)
Endothelial progenitor cells (EPCs)
Endothelial progenitor cell is a type of multipotent adult stem cell found in the bone marrow. These cells are found to line blood vessels and originated from the hESCs. The differentiation of hESCs into endothelial cells avoids the formation of an embryoid-body intermediate.
Normal bone marrow sinusoidal endothelium, umbilical cord endothelium, and human telomerase reverse transcriptase (hTERT)-immortalized endothelial cell lines can support long-term proliferation and differentiation of human stem cells. On transducing these cells with adenovectors expressing various hematopoietic growth factors (thrombopoietin; erythropoietin; granulocyte–macrophage colony-stimulating factor, GM-CSF; and c-kit Ligand, flt3/flk-2 ligand), lineage appropriated expansion of mature hematopoietic cells (red cells, megakaryocytes, and neutrophils), as well as prolonged expansion of pluripotent stem cells is observed. These transduced endothelial cells are used by researchers for support of stem cell expansion on a clinical scale in a bioreactor system (http://www.mskcc.org/research/lab/malcolm-moore/lab/endothelial-cell-biology).
Bone marrow-derived endothelial stem cells (angioblasts) also exist in the circulatory system. In a study, at first dogs had been transplanted with allogeneic marrow and further grafted with artificial arteries which rapidly became lined with endothelium that was of the marrow donor genotype. This formation of new blood vessels is known as angiogenesis.
A rare population of cells comprising <1 % of the total CD34 positive population of human bone marrow, mobilized blood, or umbilical cord blood were found to express the vascular endothelial growth factor (VEGF) Receptor-2 (VEGFR2), CD34, and the HSCs antigen AC133 (http://www.mskcc.org/research/lab/malcolm-moore/lab/endothelial-cell-biology). Purified CD34 possessing hematopoietic progenitor cells, isolated from adults, can differentiate ex vivo to an endothelial phenotype. These cells were called as EPCs which showed expression of various endothelial markers, and incorporated into neovessels at sites of ischemia. Hence, EPCs are positive for both hematopoietic stem cell markers (CD34) and an endothelial marker protein (VEGFR2). However, CD34 is not an exclusive marker of HSCs and found to be present also on mature endothelial cells. In later studies, CD133, also known as prominin, was found to be suitable marker for immature hematopoietic stem cell which helps stem cells to differentiate in endothelial cells in vitro (Urbich and Dimmeler 2004). CD133 or AC133 is a highly conserved antigen with unknown biological activity. It is not expressed on mature endothelial cells and monocytic cells but present on HSCs.
(e)
Neural stem cells
Neural stem cells exist not only in the developing mammalian nervous system but also in the adult nervous system of all mammalian organisms, including humans. The number of viable locations for neural stem cell is limited in the adult. Neural stem cells can also be derived from primitive ESCs. These are thought to be existed in the adult brain. The presence of stem cells in the mature primate brain was first reported in 1967. The clue for this has been found by the process of neurogenesis, the birth of new neurons that continues to adulthood in rats. Normally, adult neurogenesis is restricted to two areas of the brain—the subventricular zone, which lines the lateral ventricles, and the dentate gyrus of the hippocampus. However, the presence of true self-renewing stem cells has been debated. In ischemia, neurogenesis can be induced in other parts of brain such as neocortex following tissue damage. It has since been shown that new neurons are generated in adult mice, songbirds and primates, and including humans.
In vitro cultivation of neural stem cells leads to the formation of neurospheres which are floating heterogeneous aggregates of cells, containing a large proportion of stem cells. Neural cells can be propagated for extended periods of time and differentiated into both neuronal and glial cells and hence these are considered as stem cells. This behavior of neural cell is induced by the culture conditions in progenitor cells. The progeny of stem cell division normally undergoes a strictly limited number of replication cycles in vivo. Furthermore, neurosphere-derived cells do not behave as stem cells when transplanted back into the brain. Neurosphere-derived cells can differentiate into various cell types of the immune system if these are injected in blood. Potential uses of these stem cells in repair include transplantation to repair missing cells and the activation of endogenous cells to provide “self-repair.”
(f)
Olfactory adult stem cells
Olfactory stem cells have been successfully harvested from the human olfactory mucosa cells of the nose. These cells are also accessible in humans from nasal biopsies. If the appropriate chemical environment is provided to these cells, they are able to act as multipotent type of stem cells which can develop into many different cell types. Hence, they are easily grown in in vitro conditions and can be expanded to large numbers and stored frozen.
Olfactory stem cells hold the potential for therapeutic applications because these can be harvested with ease without harm to the patient. This means they can be easily obtained from all individuals, including older patients who might be most in need of stem cell therapies. The olfactory neuroepithelium undergoes continual neurogenesis and after extensive lesions or damage, fully regenerates to maintain sensory function. The olfactory epithelium is one of the few sites of adult neurogenesis, but the identity of its stem cell has been debated. Pathogens and other noxious substances constantly bombard the primary olfactory receptor neurons in the nose which kills them and results in the loss of the sense of smell. After extensive injuries resident neuronal precursors are depleted. This results in the loss of function of olfactory epithelium, if these neurons are not replaced. At this moment, horizontal basal cells (HBCs) transiently proliferate and their progeny fully reconstitutes the neuroepithelium. The pool of progenitor stem cells, that resides at the base of tissues, act as source of new neurons and can be used for the treatment of diseases.
(g)
Testicular cells
Scientists at the School of Medicine, Stanford University and at University of California, San Francisco have succeeded in isolating stem cells from human testes. Previously, it was considered as these cells show resemblance to ESCs and they can differentiate into each of the three main types of tissues of the body (Science Daily 2009). However, it is proved that the testes stem cells are not pluripotent and have different patterns of gene expression and regulation. Firstly, due to the expression of genes differ from ESCs, testicular stem cells expressed many, but not all, genes associated with pluripotency. Detailed analysis of testicular stem cells reveals the different pattern of methylation. Testicular stem cells show methylation at different region compared to ESCs which leads to the modification to DNA that affects gene expression. Secondly, they do not proliferate and differentiate as aggressively as hESCs. Finally, testicular stem cells have limited ability to form teratoma when injecting into immune-compromised mouse. These cells differ in gene expression, methylation, and in their ability to form teratomas. Together, these results suggest that the stem cells isolated from male testes have some, but not all the characteristics of true pluripotent cells. Hence, these testicular stem cells are called as multipotent stem cells.
These multipotent testicular stem cells have been derived from spermatogonial progenitor cells found in the testicles of laboratory mice. Similar types of cells were isolated from the testicles of humans by the researchers of Germany and the United Kingdom. The extracted stem cells are known as human adult germline stem cells (Waters 2008).
(h)
Umbilical cord blood stem cells
Recently, scientists have identified stem cells in umbilical cord blood and the placenta of newborn baby that are multipotent in nature. These cells can give rise to the various types of blood cells but not able to differentiate into all cell types of the body. These stem cells are found to be genetically similar to the cells of newborn. Researchers at the University of Minnesota recently found that they were able to reverse the effects of stroke in lab rats using stem cells found in human umbilical cord blood (http://news.nationalgeographic.com/news/2006/04/0406_060406_cord_blood.html). The transplanted stem cells took on properties of brain cells and seemed to be able to establish connection with brain of rat. These experiments show the possibility of using these cells in the future for therapeutic purpose. However, extensive research is required in this field. Therefore, umbilical cord blood is often banked, or stored for future use.
(i)
Adipose stem cells
Adipose tissue is an attractive alternative source of stem cells. Stem cells can be collected in large quantities from adipose tissue fragments. Human adipose stem cells (ASCs) have therapeutic applicability in pre-clinical studies in diverse fields, due to their ability to readily expand and their capacity to undergo adipogenic, osteogenic, chondrogenic, neurogenic, and myogenic differentiation in vitro. Furthermore, ASCs have been shown to be immune privileged and appear to be more genetically stable in long-term culture compared to BMSCs. The safety and efficacy of ASCs for tissue regeneration or reconstruction is currently under assessment in clinical trials.
(j)
Lung stem cells
Lung stem cells include basal cells and mucus-secreting cells in the trachea, Clara cells in the bronchioles, and type II pneumocytes in the alveoli. Mesenchymal cells predominate in fetal lung development. In postnatal life, the Clara cell is the most actively dividing cell in the tracheobronchial epithelium while type II pneumocytes are the progenitor cells for the alveolar epithelium. Under specific circumstances, basal cells and pulmonary neuroendocrine cells may also proliferate and act as stem cells.
(k)
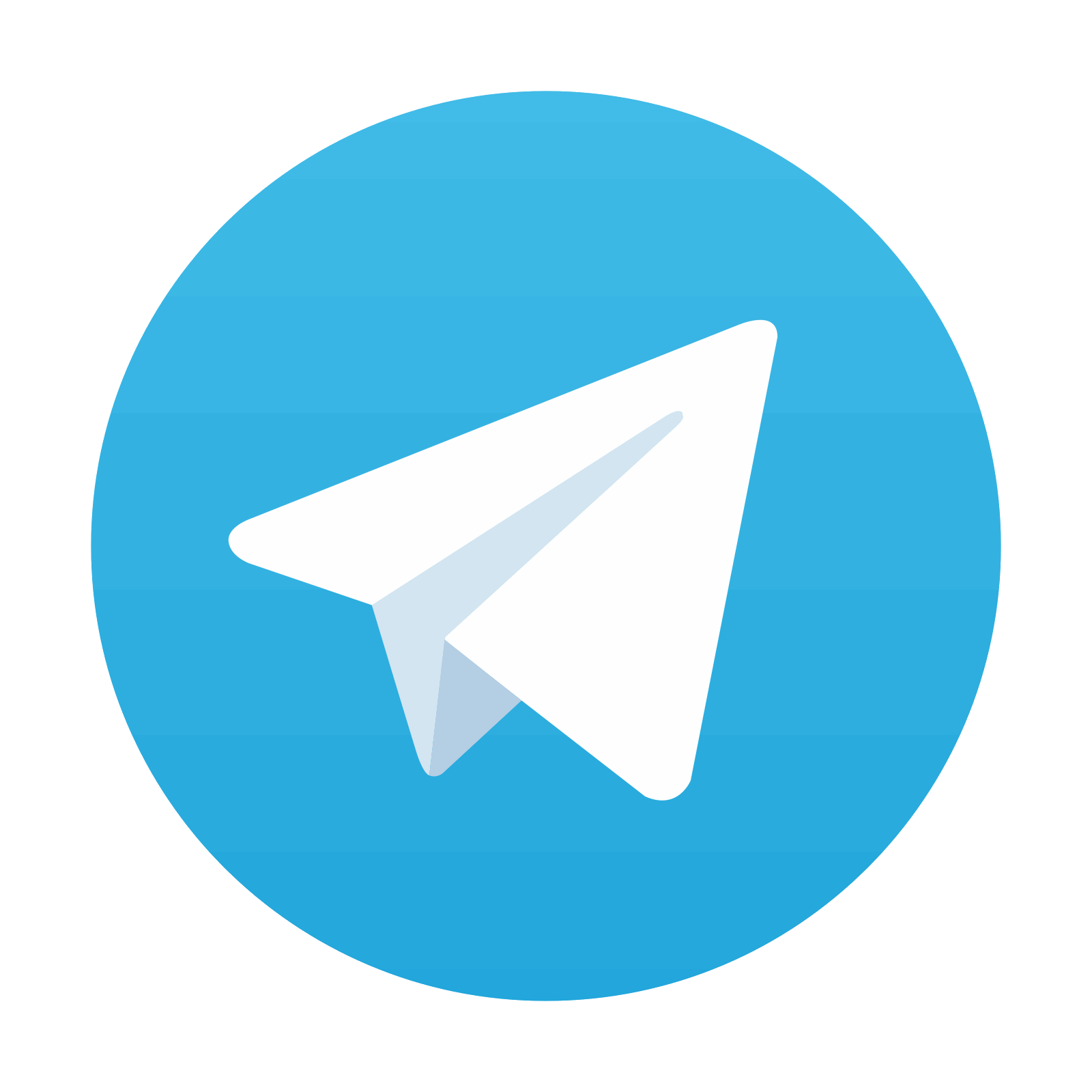
Induced pluripotent stem cells
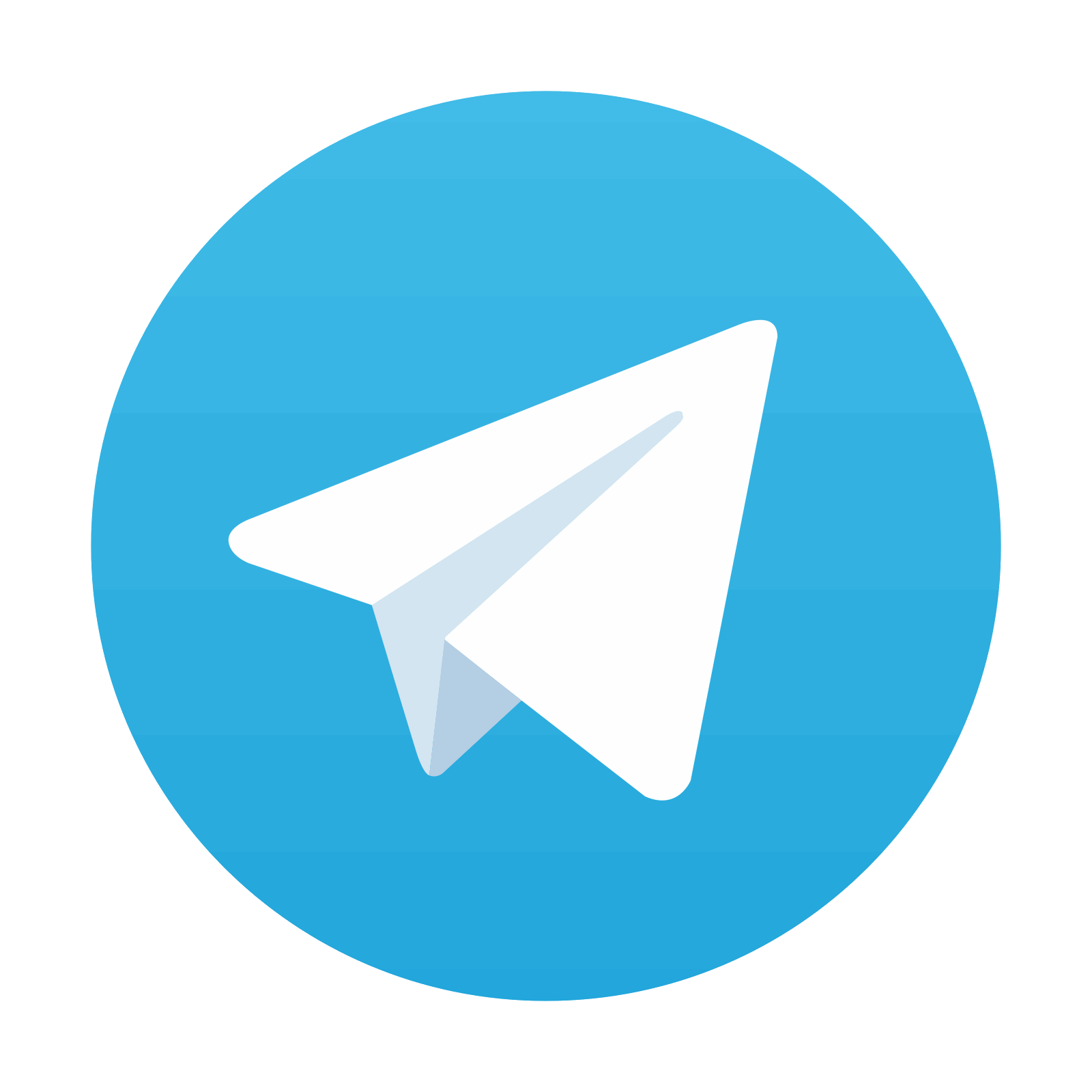
Stay updated, free articles. Join our Telegram channel

Full access? Get Clinical Tree
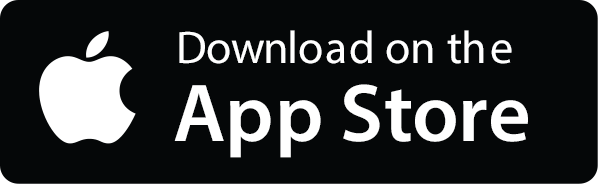
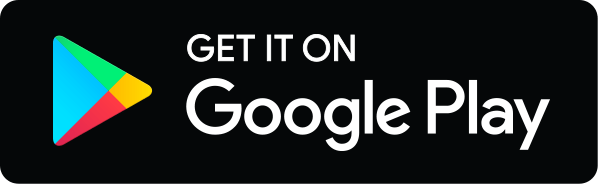
