Pulmonary Function Testing
Pulmonary function testing has come into widespread use since the 1970s. This has been facilitated by several developments.1,2 Because of miniaturization and advances in computer technology, microprocessor devices have become portable and automated with fewer moving parts. Testing equipment, patient maneuvers, and testing techniques have become widely standardized throughout the world through the efforts of professional societies. Widely accepted normative parameters have been established.
PHYSIOLOGY
With a forced exhalation, the early portion of the spirometry maneuver is characterized by high flows, mostly from large airways, and the latter portion is characterized by low flows with a larger contribution from the smaller airways.3 Forced inspiration is generally not flow limited and is a function of overall muscular effort. In contrast, a variety of factors affect expiratory flow, including the overall driving pressure, airway diameter, overall distensibility of the lungs and chest wall, dynamic airway collapse (from a flow-limiting segment), and muscular effort. The overall driving pressure is the pressure head at the alveolus, or PALV, which is the difference between pleural pressure (PPL) and negative transpulmonary pressure (PTP). So:
BATTERY OF MANEUVERS
Spirometry
Spirometry is the most commonly used lung function screening study. It generally should be the clinician’s first option, with other studies being reserved for specific indications. Most patients can easily perform spirometry when coached by an appropriately trained technician or other health care provider. The test can be administered in the ambulatory setting, physician’s office, emergency department, or inpatient setting. The indications for spirometry are diverse (Box 1). It can be used for diagnosing and monitoring respiratory symptoms and disease, for preoperative risk stratification, and as a tool in epidemiologic and other research studies.
Box 1 Indications for Spirometry
Diagnostic
To evaluate abnormal laboratory tests
To measure the effect of disease on pulmonary function
To screen persons at risk for pulmonary diseases
Some routine physical examinations
Monitoring
To assess therapeutic interventions
To describe the course of diseases affecting lung function
To monitor persons in occupations with exposure to injurious agents
To monitor for adverse reactions to drugs with known pulmonary toxicity
Evaluation of Disability or Impairment
To assess patients as part of a rehabilitation program
To assess risks as part of an insurance evaluation
To assess persons for legal reasons
Spirometry requires a voluntary maneuver in which a seated patient inhales maximally from tidal respiration to total lung capacity and then rapidly exhales to the fullest extent until no further volume is exhaled at residual volume3 (Figs. 1 and 2). The maneuver may be performed in a forceful manner to generate a forced vital capacity (FVC) or in a more relaxed manner to generate a slow vital capacity (SVC). In normal persons, the inspiratory vital capacity, the expiratory SVC, and expiratory FVC are essentially equal. However, in patients with obstructive small airways disease, the expiratory SVC is generally higher than the FVC. This difference might, however, be due partly to the difficulty in maintaining a maximum expiratory effort for an extended time period without experiencing dizziness or lightheadedness.

Figure 1 Spirogram with volumes and measurements.
(Adapted from Siberry GK, Iannone R (eds) The Johns Hopkins Hospital Harriet Lane Handbook, 15th ed. St. Louis: Mosby, 1999.)

Figure 2 Normal spirogram and subdivisions of lung volume.
(Adapted from Albert RK, Spiro SG, Jett JR (eds): Comprehensive Respiratory Medicine. St Louis: Mosby, 1999, p 43.)
A spirometer, including the waterless, rolling seal type, and Stead-Wells water seal type is an instrument that directly measures the volume of air displaced or measures airflow by a flow-sensing device, such as a pneumotachometer or a tube containing a fixed resistance to flow (Box 2).2 Today, most clinical pulmonary function testing laboratories use a microprocessor-driven pneumotachometer to measure air flow directly and then to mathematically derive volume.
Box 2 Types of Spirometers
Adapted from Miller WF, Scacci R, Gast LR: Laboratory Evaluation of Pulmonary Function. Philadelphia, JB Lippincott, 1987.
A number of spirometry standards have been developed over the years. The American Thoracic Society standardization guidelines for acceptability and reproducibility criteria are shown in Box 3.4 A well-trained pulmonary function technician usually coaches the patient through the session until the demonstrated reproducibility of key parameters suggests the results represent the best possible measure of lung function at that time.
Box 3 Acceptability and Reproducibility Criteria for Spirograms
Adapted from American Thoracic Society: Single-breath carbon monoxide diffusing capacity (transfer factor). Recommendations for a standard technique—1995 update. Am J Respir Crit Care Med 1995;152:2185-2198.
Forced Vital Capacity
FVC is a measure of lung volume and is usually reduced in diseases that cause the lungs to be smaller. Such processes are generally termed restrictive and can include disorders of the lung parenchyma, such as pulmonary fibrosis, or of the bellows, including kyphoscoliosis, neuromuscular disease, and pleural effusion. However, a reduction in FVC is not always due to reduced total volumes and can occur in the setting of large lungs hyperinflated due to severe airflow obstruction and air trapping, as in emphysema. In this setting, the FVC is decreased due to reduced airflow, air trapping, and increased residual volume, a phenomenon referred to as pseudorestriction. Reduced FVC can occur despite a normal or increased total lung volume. Therefore, FVC is not a reliable indicator of total lung capacity or restriction, especially in the setting of airflow obstruction. The overall accuracy of the FVC for restriction is about 60%.5
Volume-Time Tracing and Flow-Volume Loop
The volume-time tracing and flow-volume loop ascertain the technical adequacy of a maneuver and therefore the quality of the data (see Box 3) as well as identifying the anatomic location of airflow obstruction. The volume-time tracing is most useful in assessing whether the end-of-test criteria have been met, whereas the flow-volume loop is most valuable in evaluating the start-of-test criteria. The technique of back-extrapolation of the start of the test to establish a zero time point on the volume-time tracing has been carefully defined and provides a uniform start point for timed measurements. It corrects for delayed or hesitant starts that might otherwise be mistaken for a falsely reduced FEV1. Standards for acceptability define limits for the degree of hesitation that can still yield an acceptable FEV1 (see Box 3). The loss of elastic recoil characteristic of emphysema results in airflow limitation during the maximal forced exhalation that may be grossly underestimated if the patient applies less than maximal expiratory force. Such efforts may still be deemed acceptable using the criteria of extrapolated volume. The time to peak flow appears to have excellent usefulness in identifying such efforts in this population (time to peak flow will be greater than 120 msec when effort is submaximal), but it is not yet a recommended acceptability criterion (Fig. 3).

Figure 3 Spirogram illustrating the back extrapolation method of determining time zero.
(Adapted from Miller MR, Hankinson J, Brusasco V, et al: American Thoracic Society/European Respiratory Society Task Force: Standardization of spirometry. Eur Resp J 2005;26:319-338.)
The shape of the flow-volume loop can indicate the location of airflow limitation, such as the large upper airways or smaller distal airways (Fig. 4). With common obstructive airflow disorders, such as asthma or emphysema, the disease generally affects the expiratory limb and can reduce the effort-dependent peak expiratory flow as well as subsequent airflows that are independent of effort. The descending limb of the expiratory loop is typically concave. In contrast, several unusual anatomic disorders that narrow the large airways can produce a variety of patterns of truncation or flattening of either one limb of the loop (variable upper airway obstruction) or both limbs of the loop (fixed upper airway obstruction).
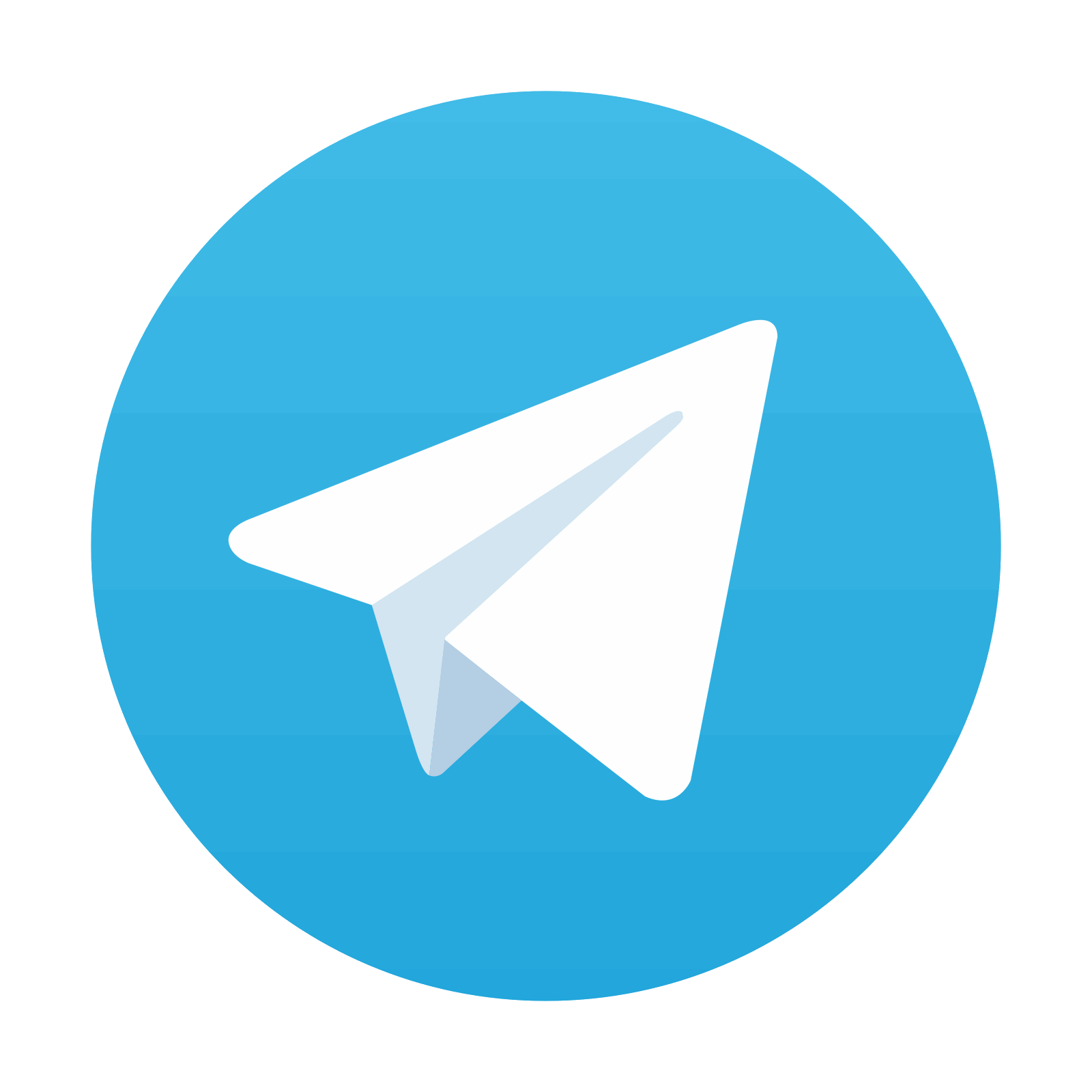
Stay updated, free articles. Join our Telegram channel

Full access? Get Clinical Tree
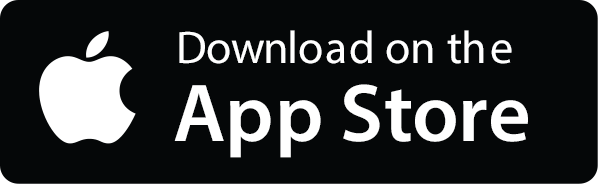
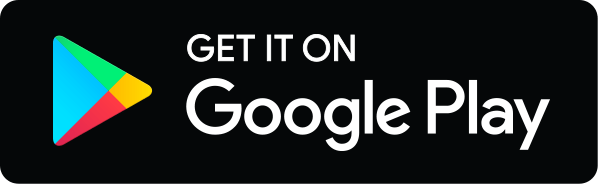