Fig. 7.1
The mechanism underlying NO-repressed autophagy. NO inhibits JNK1 phosphorylation by S-nitrosylation. Decrease of phospho-Bcl-2 and increase of Bcl-2-Beclin1 interaction disrupt Vps34-Beclin1 association. NO also inhibits IKKb phosphorylation by S-nitrosylation. Decrease of phospho-AMPK and TSC2 activity alleviate the inhibitory effect of TSC1/2 on Rheb (denoted by “×”), thereby allowing Rheb to activate mTORC1 for inhibition of autophagy. RAP induces autophagy by inhibiting mTORC1, whereas resveratrol and CR induces autophagy by activating SIRT1 or inactivating mTORC1. SIRT1 accelerates mitochondrial biogenesis by activating PGC-1α
While the overexpression of NOS impairs autophagic flux, the inhibition of NO production induces autophagy. The NOS inhibitor l-NMMA also induces autophagy but is independent on mTORC1 activity or Bcl-2 phosphorylation. Interestingly, deprival of NO by l-NMMA or the endogenous dominant-negative NOS regulator NOS4 reduces mutant huntingtin aggregation and neurodegeneration in the fruit fly Huntington disease model.
Accumulation of amyloid-β and tau is an invariant feature of Huntington disease, but RAP-induced autophagy allows a significant reduction in amyloid-β levels (Caccamo et al. 2010). Although S-nitrosylation is able to interpret the NO-enhanced autophagy and alleviated neurodegeneration, it has not determined whether S-nitrosylation is a general mechanism of NO-impaired autophagy or only represents a randomized event. Cho et al. (2009) have demonstrated that the S-nitrosylation of dynamin-related protein 1 (DRP1) rich in the brains of Alzheimer’s disease patients increases GTPase activity and mediates β-amyloid-related mitochondrial fission and neuronal injury. However, Bossy et al. (2010) have refuted that the S-nitrosylation of DRP1 does not affect GTPase activity and is not specific to Alzheimer’s disease. This discrepancy may be attributed to the reversible feature of S-nitrosylation, but another possibility is the alternative modifications of DPR1 or downstream proteins by S-nitrosylation.
NO can also lead to the nitration of tyrosine and other amino acid residues through modification by ONOO− except for the S-nitrosylation of cysteine. Treatment of rats or mice by LPS in vivo or ONOO− in vitro significantly promotes the formation of 3-nitrotyrosine in insulin receptor substrate 1 (IRS-1) and reduces the insulin-dependent tyrosine phosphorylation, eventually leading to the insulin resistance of skeletal muscles (Pilon et al. 2010). The enhanced 3NT modification of mitochondrial proteins occurs with aging, in which the nitration of F1-ATPase at tyrosine 269 leads to ADP binding to the enzyme’s active center, and is associated with a moderate impairment of the mitochondrial function (Lam et al. 2009). If the nitration of tyrosine occurs globally, it seems reasonable to interpret the results of Sarkar et al. (2011) by the nitrosative inactivation of separated signaling proteins of the autophagy machinery. Besides, l-NMMA abrogates neurodegeneration perhaps through directly blocking the misfolding and aggregation of proteins such as Huntingtin due to S-nitrosylation or 3-nitration.
Indeed, S-nitrosylation of protein-disulfide isomerase or the E3 ubiquitin ligase parkin is found to initiate protein misfolding and aggregation in Parkinson’s disease (Gu et al. 2010). Previously, overexpression of nNOS was detected in the brains of Parkinson’s disease patients (Eve et al. 1998). The existence of 3NT was found in the core of Lewy bodies, the pathological hallmark of Parkinson’s disease progression (Good et al. 1998). The observation of nNOS was correlated with the presence of 3NT in circulating neutrophils from Parkinson’s disease patients (Gatto et al. 2000). Recent studies have shown that α-synuclein is one of the major building blocks in Lewy bodies (Ischiropoulos 2009). It is apparent that the majority of Lewy bodies and protein inclusions contain nitrated and oxidized α-synuclein, indicating that oxidation is participated in the formation of these inclusions. Additionally, nitration or nitrosylation of ubiquitin E3-ligases can result in the defective transfer of proteins to ubiquitin. In the proteosome, mutations of the E3-ligase parkin explain the appearance of juvenile Parkinson’s disease by the less degradation of NOS and aggravation of excess NO-mediated mitochondrial damage and complex I abrogation (Boveris et al. 2010).
Due to reaction with the excessive O2 − leading to generation of ONOO−, the scarcely available NO should exist during aging. Indeed, senescent endothelial cells display higher mTORC1 activity, increased O2 − production and decreased bioactive NO levels than young endothelial cells. This is contributed by the so-called “uncoupling” of NOS that does not produce NO but O2 −. Silencing mTORC1 in senescent cells reduces O2 − generation and enhances NO production, whereas the overexpression of a constitutively active mTORC1 mutant in young endothelial cells mimics endothelial dysfunction of senescent cells through NOS uncoupling and induces premature cellular senescence. RAP and resveratrol, by inhibiting mTORC1 signaling, result in the decrease of O2 − levels, but increase of NO levels in the senescent cells as well as in the aortas of old rats (Rajapakse et al. 2011). Likewise, short-term CR initiated in old age reverses age-associated vascular endothelial dysfunction by restoring NO bioavailability, reducing NADH oxidase-mediated O2 − production, stimulating antioxidant enzyme activity, and upregulating SIRT1 (Rippe et al. 2010).
7.2 Low-Grade Inflammation as an Essential Consequence of Obesity?
In regard to obesity, there are many unsolved problems. Is obesity a disease? Is the fat deposited in the subcutaneous adipose tissue (SAT) benign, but the fat deposited in the visceral adipose tissue (VAT) malignant? Does the brown adipose tissue (BAT) or the white adipose tissue (WAT) distinguish the healthy or unhealthy obesity? Does chronic inflammation induce obesity and insulin resistance? Does the antihypoxic intervention reduce body weight and improve insulin sensitivity?
There has a definition of obese/lean not strictly based on the body mass index (BMI), but has no specific standards to distinguish the composition of an individual that is composed of the adipose and muscle tissues. However, some above mentioned terms such as SAT or VAT as well as BAT or WAT have been employed to describe the features of adiposity. SAT localizes under the skin, arms, breasts, buttocks, hips, and thighs, while VAT distributes around or within the liver, heart, muscles, and pancreas. BAT rather than WAT has an extraordinary number of mitochondria and numerous capillaries, allowing it to look dark and gland-like. BAT is more active than WAT in lipid degradation and energy expenditure.
Although the American Medical Association (AMA) declare obesity to be a disease, Katz (2014) recently argued that obesity is not a disease, but a risk factor of other chronic diseases. This is because not only can chronic diseases develop in the absence of obesity, but not every obese person develops any such conditions. A BMI-based thin person might have an increased fat depot in VAT. In similar, those individuals with a normal BMI might possess a higher body fat proportion. Compared with people with a normal body fat composition, those persons with VAT have a nearly fourfold risk of prediabetes, and nearly twice as likely to have high blood pressure or heart disease (Levine and Levine 2011). As to BAT and WAT, while adults have much WAT, new born babies have much BAT. Obese individuals have more WAT than do lean individuals.
Is low-grade inflammation originated from obesity? There are much discrepant opinions regarding the origin and cause of obesity-induced inflammation. Some authors thought that inflammation might be derived from the activated macrophages within the adipose tissue (Weisberg et al. 2003), some authors suggested that increased adipocyte O2 consumption would trigger HIF-1α, causing inflammation and insulin resistance in obesity (Lee et al. 2014), and other authors supposed that hypoxia-induced heme oxygenase-1 (HO-1) might drive the chronic inflammation and insulin resistance (Jais et al. 2014). However, it was also believed that macrophage activation induces proinflammatory cytokines, which can in turn activate iNOS to produce NO and drive hypoxia via HIF-1α.
A logical relationship is inflammation cause hypoxia, hypoxia results in reduced energy expenditure, and reduced energy expenditure leads to obesity. Indeed, BAT is usually “whitening” due to decreased mitochondria and compromised angiogenesis (Shimizu et al. 2014). So it can be anticipated that anti-inflammation and antihypoxia are generally effective for weight reduction. It was found that chronic blockade of iNOS by l-NMMA reduces adiposity and improves insulin resistance in HFD-induced obese mice (Tsuchiya et al. 2007). Through anti-inflammation, salisylate as a degraded product of aspirin was found to reduce circulating lipids in obese rats and to improve insulin sensitivity (Yuan et al. 2001). Like metformin, aspirin was shown to able to treat type II diabetes as an activator of AMPK (Hawley et al. 2012). Nitroaspirin was suggested to have therapeutic potential for NAFLD (Ibrahim et al. 2011). Overexpression of or supplementation with EPO induces reduced blood glucose levels and body mass in mice (Katz et al. 2010). Similarly, it was also noticed that EPO treatment can inhibit WAT inflammation, normalized insulin sensitivity, and reduced glucose intolerance in mice (Alnaali et al. 2014).
What are the primary and cardinal reasons triggering adipose, muscular, and systemic inflammations? Such issues are debating in either noninfectious origin or infectious origin. Nevertheless, some scientists seem to insist a dual origin pattern, by which the free fatty acids (FFA) and LPS can equally activate proinflammatory cytokines and induce insulin resistance (Heinrichsdorff and Olefsky 2012). Although some authors supposed FFA-mediated inflammation in obesity, they are unable to reasonably explain how can FFA do this. While the interaction of LPS with Toll-like receptor 4 (TLR4) via CD14 has been confirmed, an interaction of FFA with TLR4 is illy elucidated for a long time. Eventually, the liver secretory protein Fetuin A (FetA) was found to function as an adapter between FFA and TLR4, by which the triple interaction of FFA with TLR4 via FetA has been established (Pal et al. 2012). Nevertheless, it remains awaiting for answering why short-chain fatty acids (SCFAs) prevent rather than promote obesity (Blaut 2014), and how can FetA distinguish and selectively bind to saturated FFA but not unsaturated FFA.
Interestingly, a previous finding delineated that the disruption of FatA expression renders animals more susceptible to endotoxemia, whereas the supplementation of FetA confers protection against lethal endotoxemia (Li et al. 2011). This result implies that elevation of FetA might be due to LPS accumulation in obesity because FetA can competitively bind to TLR4 with LPS. In fact, it seems that the LPS-originated inflammation theory has evoked much enthusiasms in recent years. Ding et al. (2010) indicated that intestinal inflammation precedes and correlates with HFD-induced obesity, adiposity and insulin resistance. They suggested that HFD interact with gut microbiota to trigger the expression of TNF-α and NF-κB. The absence of gut microbiota in germ-free mice blunts the upregulation of primary inflammatory indicators. A relevance of HFD to gut dysbiosis was revealed as that HFD increases the ratio of Firmicutes to Bacteriodetes, and induces the overgrowth of Enterobecteriaceae, which exacerbates inflammation and obesity in mice via the TLR4 signaling pathway (Kim et al. 2012). A recent work indicated that adipocyte inflammation is essential for healthy adipose tissue expansion and remodeling, in which visceral fat is deposited for effectively filtering the gut-derived LPS (Asterholm et al. 2014). This finding seems imply an association of gut bacterial dysbiosis with visceral adipose storage.
Recently, we investigated the effects of different dosages of LPS on the expression levels of proinflammatory cytokines in mice fed with HFD. Surprisingly, we found that low-dose (0.25 mg/kg) LPS enhances the low-grade inflammation in the visceral tissue, whereas high-dose (1.2 mg/kg) LPS does not induce or even suppresses inflammation in the peripheral tissue. For example, intramuscular injection of HFD-induced mice with 1.2 mg/kg LPS extremely downregulates proinflammatory cytokines in the skeletal muscle. In contrast, intraperitoneal injection of HFD-induced mice with 0.25 mg/kg LPS mildly upregulates proinflammatory cytokines for only 2–3 folds.
Accordingly, we also noticed that the serum NO and 3NT levels that represents the extent of iNOS activation by proinflammatory cytokines are gradually declined during high-dose LPS injection. In contrast, low-dose LPS can mimic the gastrointestinal infection by G− bacteria that produce and leak LPS into the blood stream. It could be concluded that low-grade inflammation is originated from low-dose LPS, but we are currently unknown why high-dose LPS exerts an immunosuppressive effect. We found that ART, DNP, and NG exert weight-reducing effects through anti-inflammation and mitochondrial biogenesis. For example, ART, DNP, and NG were found to downregulate iNOS and downstream genes, and upregulate eNOS and downstream genes, suggesting activated eNOS/inactivated iNOS might be a pivotal switch for weight loss.
I propose here a novel hypothesis of healthy/unhealthy obesity based on the comparison of low-grade inflammation, insulin resistance, SAT/VAT, and BAT/WAT (Fig. 7.2).


Fig. 7.2
A hypothesized mechanism of adipogenesis/obesity. ARG l-arginine; BAT brown adipose tissues; BMI body mass index; CO carbon monoxide; eNOS endothelial nitric oxide synthase; EPO erythropoietin; G − Gram negative bacteria; G + Gram positive bacteria; HIF-1α hypoxia inducible factor α; HO–1 heme oxygenase 1; iNOS inducible nitric oxide synthase; IR insulin receptor; LFD low-fat diets; LPS lipopolysaccharide; nNOS neuronal nitric oxide synthase; NO nitric oxide; SAT subcutaneous adipose tissues; SCFA short-chain fatty acids; VEGF vascular endothelial growth factor; VAT visceral adipose tissues; WAT white adipose tissues
Healthy obesity was assumed to be germ-free (without LPS leakage) or G+ bacteria-induced adiposity with a high BMI but without VAT and insulin resistance, whereas unhealthy obesity was supposed to be G− bacteria-induced adiposity with high BMI, VAT and low-grade inflammation, probably exhibiting insulin resistance or type II diabetes. Chronic inflammation is most likely derived from an interaction of HFD with gut dysbiosis, leading to the overgrowth of G− bacteria and the leakage of bacterial LPS. Upon activation of proinflammatory cytokines, LPS can upregulate the expression of iNOS and HO-1, which directly lead to the overproduction of NO and CO. Both gas molecules can competitively bind to hemoglobin and myoglobin with O2, leading to metabolic hypoxia. The hypoxic condition can subsequently induce HIF-1α, VEGF, and EPO, thereby driving angiogenesis and adipogenesis. The commonly occurred hyperinsulinemia/hyperleptinemia in obesity might be explained by the ONOO−-mediated nitrosylation/nitration of insulin/leptin receptors. For BAT whitening to WAT in obesity, it might be explained by the dramatic decline of NO that is derived from eNOS/nNOS and the suppression of mitochondrial biogenesis.
By comparison of the gut microbiota between the healthy adults and the patients with type II diabets, Qin et al. (2012) found the hypoproliferation of butyrate-producing bacteria and the overgrowth of sulfate-reducing bacteria among other abnormalities of gut microbiota. The butyrate-producing bacteria are fiber-digested bacteria, whereas the sulfate-reducing bacteria are meat-addicted bacteria. For example, the δ-proteobacteria, Desulfovibrio piger, without any forms of sulfatases can only survive in the gut depending on the sulfate released from Bacteroides thetaiotaomicron. It was found that chondroitin sulfate and mucin are the main sources of sulfate uptaken by B. thetaiotaomicron. Interestingly, enhanced mucus degradation was considered a couse of reduced gut lining integrity (Rey et al. 2013).
The gastrointestinal tracts of centenarians were recently found to have less abundant sulfate-reducing bacteria such as Desulfovibrio, but have much plentiful butyrate-producing bacteria such as Clostradia (Wang et al. 2015). Nevertheless, it is currently inconclusive whether obesity is caused by the dysbiosis between sulfate-reducing bacteria and butyrate-producing bacteria. However, we can image that the trace amount of hydrogen sulfide that is released by less abundant sulfate-reducing bacteria might be beneficial to extend lifespan (Qabazard et al. 2014), but overproduction of hydrogen sulfide by the overgrown sulfate-reducing bacteria might induce the cancerous alteration (Attene-Ramos et al. 2007) and initiate an adipogenetic process (Tsai et al. 2015) directly or indirectly through conversion of nitrate/nitrite to large-amount NO (Vermeiren et al. 2012).
Given that gut bacteria, especially G−, are responsible of LPS leakage and inflammation induction, how to prevent such an adverse outcome? The most important consideration should be prevent the dysbiosis of gut microbiota. For this purpose, you should maintain a balanced daily diet that contains low fat and high fiber. If you are an obese person or a patient with type II diabetes, you should restrict your calorie uptake, and recover your microbiota homeostasis. For example, it is beneficial to eat more fiber-rich vegetables and fruits to nourish the butyrate-producing bacteria, but eat less meats or chondroitin sulfate-rich foods to avoid the overgrowth of sulfate-reducing bacteria.
Interestingly, dietary yeast has been reported to reduce hepatic steatosis, obesity, and type II diabetes in mice (Everard et al. 2014) as well as neuroinflammation in mice (Takata et al. 2015), suggesting that yeast might narrow the occupying space by bacteria, and compromise LPS-induced inflammatory diseases. If this situation is also confirmed in human, supplementation with dietary yeast as an probiotic alternative to bacterial prebiotics might be helpful to decrease the risk of obese, fatty liver, and type II diabetes.
7.3 The Origin of CSC: Next Breakthrough on Tumorigenesis/Carcinogenesis?
Regardless of debating on the concept of CSC, tumorigenesis/carcinogenesis must experience the extensive genetic alteration and chromosome instability. It is known that the chronic inflammation is linked to cancer by rehearing the scenarios of tumor initiation, promotion, malignant conversion, invasion, and metastasis (Grivennikov et al. 2010). How inflammation causes tumor/cancer remains largely unknown, but many types of tumor/cancer are known to be originated from a long-term immune activation that upregulates proinflammatory cytokines and triggers NO burst. Given that NO is implicated in inflammation-originated tumor/cancer, how it acts on, directly or indirectly, maturated cells or stem sells remains obscure. Nevertheless, we could still anticipated that the origin of tumor/cancer should at least engage the following effectors: (1) NO-driven hypoxia. Sustained NO burst due to chronic inflammation may cause hypoxia, angiogenesis and hyperplasia that are seen in the benign tumor. (2) ONOO−-mediated mutagenesis and modification. The concomitant occurrence of high-level NO and O2 − promotes the formation of ONOO− and enables the denaturation of proteins and DNA, which are frequently seen in either benign or malignant tumor/cancer. (3) NO-confered cytoprotection. Tumor/cancer cells are characterized by extreme longevity, and there must be an association of NO with antiapoptosis, antiautophagy, antisenescence, and anticytotoxicity in tumor/cancer cells.
Hypoxia promotes tumor development through multiple mechanisms including initiating tumor angiogenesis (Choi et al. 2003), causing genome mutagenesis (Papp-Szabo et al. 2005), maintaining anaerobic metabolism (Gillies and Gatenby 2007), and modifying acidic microenvironment (Fang et al. 2008; Chen et al. 2010; Hjelmeland et al. 2011). Early in 1998, Wink et al. had reviewed that chronic inflammation can lead to the de novo production of many kinds of chemical intermediates, among which ONOO− that mediates nitrogen stress is able to make DNA damage. Previously, it was detected that purine and pyrimidines deamination, strand breaks and purine modification, and nitroguanine adduction can occur upon exposure of DNA to ONOO− (Yermilov et al. 1995; Zingarelli et al. 1996). Usually, DNA damage necessarily leads to DNA repair by tumor suppressors, thereby uneasily leading to genetic mutagenesis. Surprisingly, why are genetic mutations not rare in tumor/cancer? The most possibility is that ONOO− inactivates DNA repair responsible enzymes such as tumor suppressors, p53 and BRCA1, via nitrosylation/nitration. Therefore, genes are highly prone to be mutated when DNA repair responsible tumor suppressor genes have been mutated. Indeed, evidence for ONOO−-mediated p53 modification in human gliomas had been declared (Cobbs et al. 2001).
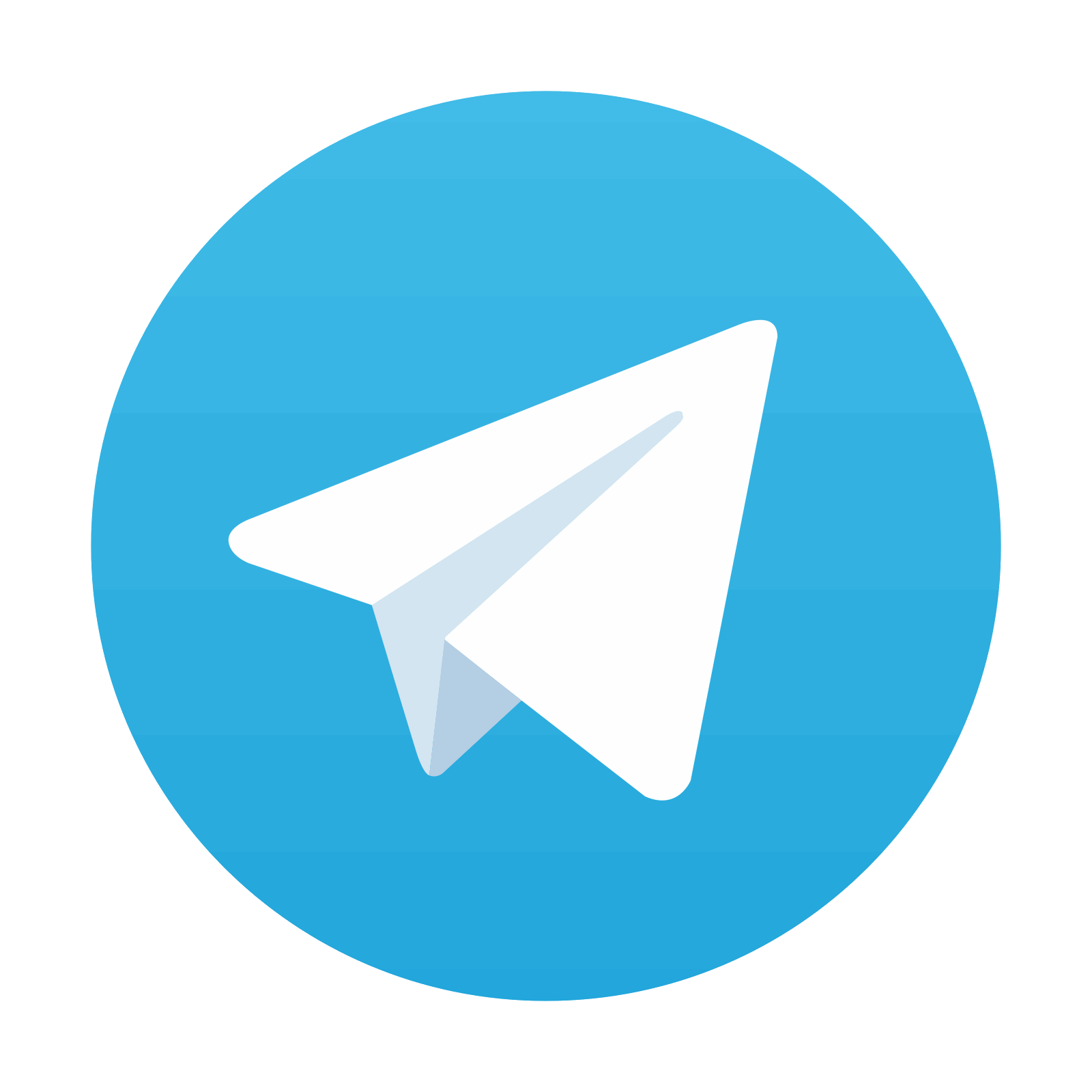
Stay updated, free articles. Join our Telegram channel

Full access? Get Clinical Tree
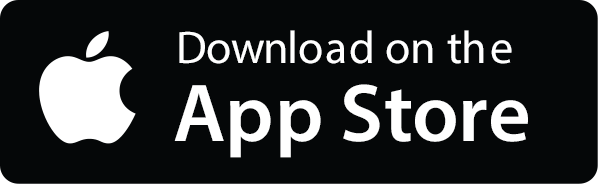
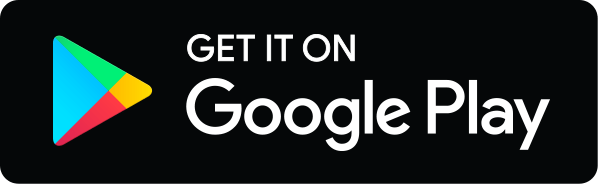