18 Christopher M. Teaf, Michele M. Garber, and J. Michael Kuperberg For purposes of this chapter, solvent classes and individual examples are presented, as well as selected substances (e.g., vinyl chloride, polycyclic aromatic hydrocarbons (PAHs)) not typically viewed as solvents but that are addressed in conjunction with solvents or are major elements of solvent mixtures. Information is provided concerning solvent chemistry, toxicology, and potential health effects, including: Solvents are defined as chemicals capable of dissolving and dispersing other substances. That fundamental property explains the economic value and potential health significance in residential, commercial, and environmental circumstances for products used as cleaners, degreasers, chemical intermediates, and chemical delivery vehicles. Organic solvents are carbon-based substances representing a very large, chemically diverse group of industrially, occupationally, and ecologically important products. The first historically useful organic solvents (e.g., ethanol, other alcohols, wood resins, turpentine) were derived from vegetable sources, and development of cosmetics containing organic solvent components dates to ancient Egypt. Inorganic solvents (e.g., water, ammonia, inorganic acids) are not addressed in this chapter, but comprise a chemically and economically important group as well. There are thousands of organic solvents and related compounds, both pure compounds and commercial mixtures (e.g., mineral spirits, naphthas). The large numbers often result in generic statements about what solvents are or what they are not, as well as generalization about toxicity. However, there are marked differences among even similarly structured solvents. For example, low-molecular-weight members within a chemical class often exert greater toxicity than higher class members, due to differences in volatility, water solubility, and ability to cross biomembranes. That diversity demands care when comparing chemical and toxicological properties. Solvent exposure in the human environment is common, due to widespread use in occupational settings of many large industries and small commercial operations (e.g., automotive shops), and common potential for exposure via household products. Such exposure can be by oral (e.g., drinking water), dermal (e.g., bathing, industrial direct contact), or inhalation routes (e.g., airborne workplace exposure, residential vapor intrusion), or a combination, depending upon the circumstances. This chapter addresses airborne exposure potential in detail, due to the volatile nature of many solvents. However, as noted, solvent exposure is possible by multiple routes. For example, in situations where groundwater with solvent contamination is used for household purposes, there is the possibility of ingestion from drinking the water, dermal exposure from bathing activities, and inhalation related to volatilized solvents during clothes washing, showering, cooking, and other chores. Similarly, in industrial operations that employ solvents, joint exposure may occur due to splashes onto the skin, inhalation of vapors in work areas, and hand-to-mouth transfer of the chemicals during eating, smoking, or other common activities. Recognition of these multiple potential pathways has led to workplace requirements involving respiratory protection and hygiene practices limiting exposure possibilities. Differences in absorption, and other route-specific considerations for solvents, are further addressed in Section 18.3. Not only is it important to address potential exposures to individual solvent agents but also possible interactive effects of multiple exposures, since these are the rule, rather than the exception. Assessment of the magnitude of exposure is often complex and may require detailed evaluation of inhalation and/or dermal contact, as well as estimates of exposure frequency and duration. Some common volatile organic chemical (VOC) solvents that may be encountered indoors and outdoors include benzene, toluene, xylenes, alcohols, trichloroethylene (trichloroethene, TCE), and formaldehyde. Exposure to organic solvents and their constituents can occur for individuals who live near industrial facilities that presently use or have used these solvents, as well as in the home. Many solvents are found in groundwater, soils, air, and other environmental media near National Priorities List (NPL) sites that are managed under Superfund (Comprehensive Environmental Response, Compensation, and Liability Act, aka CERCLA). Much of this is related to past storage, handling, and disposal practices at numerous industrial facilities. As of September 2014, there were over 1300 listed NPL sites and another 51 proposed sites in the United States, many of which are significant for the presence of variable solvent contamination profiles in soils and groundwater. There have been extensive advances in worker protection standards in the United States; however, industrial solvent exposures remain of global health interest for workers, many of whom are unfamiliar with potential hazards associated with exposures. The Occupational Safety and Health Administration (OSHA) has concluded that millions of U.S. workers are exposed to solvents in varying degrees on a daily basis. In some professions (e.g., painters), nearly all workers may have some degree of exposure, though education and protective measures (e.g., ventilation, spraybooths, respirators), coupled with introduction of water-based paints and adhesives, have reduced such exposure. Japan reports that smaller industries use nearly five times as much solvent volume compared to large enterprises, and they have experienced shifts in solvent use from aromatics to alcohols. Exposures to organic solvents in an occupational setting also may occur in research laboratories, hospitals, and dry cleaners. In histology labs, formaldehyde (preservative) and xylenes (clearing agents) are the most common chemicals used and historically have been associated with pulmonary damage. Recently, housekeeping practices in the healthcare industry, which employ solvent-based cleaning products, have been identified as an occupational risk due to the asthma-like symptoms and dermatitis reported by some workers. A limited epidemiologic database suggests that exposure to solvents and volatile chemicals in service station workers may be associated with restrictive airway disease, but demonstration of persistent lung complications has been inconsistent. A relationship also has been postulated for exposure to some chlorinated solvents during automotive degreasing work and an increase in risk of non-Hodgkin’s lymphoma (NHL); however, that literature also is inconsistent. Ubiquity of solvents in industry, and potential for concurrent or sequential exposure to multiple chemicals, complicates the distinction between chemical causation of adverse effects and simple association of adverse effects with exposure in time and place. Relationships between simple association and chemical causation are discussed elsewhere in this book (see Chapter 21). Industrial practices that result in the evaporative loss of volatile solvents (e.g., metal degreasing, application of surface coatings, chemical separations) are of particular interest in an exposure context. Protective equipment, engineering controls, and work practices can be effective in limiting exposures, but careless or inexperienced handling of solvents may still occur in small facilities (e.g., automobile service and repair, metal fabricators) and during activities in large and otherwise well-run factories and service industries. Methods used for worker exposure characterization and quantification are discussed elsewhere in this book (see Chapter 22). Solvent exposure potential varies among individuals, as well as over time for a specific individual, based on job type, workplace duties, and schedule. Within possible occupational exposure groups, it is important to consider sensitive individuals and populations when evaluating exposure and risk, including those with preexisting health conditions such as weakness of the immune system. Occupational airborne guidelines that are designed to control exposures to solvents and other materials in the workplace may be expressed in units of volume/volume (e.g., parts per million (ppm)), as well as units of mass/volume (e.g., mg per cubic meter (mg/m3)). For vapors and gases, these data if expressed in either form may be interconverted according to the following expressions: where Rearranging this expression provides an opportunity to convert airborne concentrations that are expressed with different units in the other direction as well, as follows: For dose estimates, units of mg/m3 are useful in conjunction with inhalation rates (units of m3/h or m3/day) to determine chemical intake in risk calculations. These unit conversion relationships do not apply for dusts, aerosols, and other nongaseous forms. As noted, in addition to what are considered conventional industrial exposures, potential exposures to household products containing solvents and nonoccupational handling of petroleum products remain significant sources of exposure to hydrocarbon solvents. Residential exposure by all routes can occur when practicing hobbies (e.g., paints, thinners, adhesives), conducting home repairs (e.g., paints varnishes, thinners), using household cleaning products (alcohols, ethers, floor strippers, tub/tile cleaners), using fuels in lawn equipment (gasoline, diesel), and during recreational abuse of volatile inhalants, known as “huffing,” in which a variety of solvents and pressurizing agents are used to attain intoxication-related euphoria, delusions, sedation, and hallucinations. Chronic inhalant abuse may lead to adverse neurologic conditions. Structural variability and the range of physical/chemical properties exhibited by organic solvents limit the number of generalizations that can be made regarding physiological effects and exposure hazards. However, because of their common industrial, commercial, and household use, often in large quantities, it is useful to discuss some fundamental characteristics that are common to the principal classes of organic solvents. Table 18.1 summarizes selected important physical/chemical properties for solvents discussed in subsequent chapter sections. Of particular interest are vapor pressure (i.e., volatility) and water solubility, since these properties greatly influence environmental behavior and exposure potential. Many organic solvents are flammable/explosive, depending on their volatility, and have lower densities than water, except for some halogenated solvents. Solvent toxicity is greatly influenced by the number of carbon atoms, whether as a saturated or unsaturated molecule, as well as the chemical configuration (e.g., straight chain, branched, cyclic). Table 18.1 Physical/Chemical Properties of Representative Solvents and Related Materials Table 18.2 presents occupational guidelines and standards for selected solvents and solvent constituents. These values include the health-based guidelines of the American Conference of Governmental Industrial Hygienists (ACGIH), termed threshold limit values (TLVs), as well as legally enforceable standards developed by the OSHA, termed permissible exposure limits (PELs). These guidelines and standards may be viewed as long-term protective levels, represented by a time-weighted average (TWA), as well as a protective value for a shorter time frame, termed a short-term exposure limit (STEL) or a ceiling (C) concentration. To the extent that they are available, carcinogen classifications from the United States Environmental Protection Agency (U.S. EPA), ACGIH, and National Toxicology Program (NTP) are included as well. Nearly half of the listed chemicals on Table 18.2 have no U.S. EPA carcinogenic classification, due to limitations to what the agency considers acceptable data. Table 18.2 Occupational Exposure Limits for Selected Solvents and Related Materials K, known to be a human carcinogen; NE, not established; NIC, Notice of Intended Changes; PEL, permissible exposure limit; R, reasonably anticipated to be human carcinogens; RfD, reference dose; STEL/ceiling, short-term exposure limit or ceiling; TLV, threshold limit value; TWA, time-weighted average; see Table 18.4 for additional abbreviations. In addition to the occupational air guidelines and standards, the ACGIH has published a series of biological exposure index (BEI) values for substances of specific industrial interest to guide the monitoring and control of health hazards. The BEIs are measures of a specific chemical or its metabolites in biological media (e.g., urine, blood, expired air). In principle, the BEI value is that which would be expected if airborne exposure regularly occurs at the TLV. The BEI is complementary to workplace air monitoring and can be a useful adjunct in situations where information is variable or contradictory. For example, in a situation where the BEI is exceeded but where workplace air levels are low, it would be prudent to investigate potential unrecognized peaks in air levels or to investigate the potential for unrecognized dermal exposure and absorption. Table 18.3 presents BEI values for selected solvents and related materials. Table 18.3 ACGIH Biological Exposure Index (BEI) for Selected Solvents and Related Materials. B, background; Ns, nonspecific; Nq, nonquantitative; Sq, semiquantitative. a With hydrolysis. b After hydrolysis. c Without hydrolysis; metabolite is specific to n-hexane and methyl n-butyl ketone. d Without hydrolysis. Table 18.4 provides definitions and differences among the most common occupational guidelines described in Table 18.2, as well as carcinogen classifications used by the U.S. EPA and ACGIH. As noted, the PEL is the legally enforceable standard for workplace air exposure and governs employers with regard to engineering practices, ventilation, and personnel protective equipment. It considers cost and technical feasibility in its development, as well as health considerations. The TLV is a guideline criterion that is based on health considerations only, derived from available animal and human toxicology information. In that respect, the TLV is analogous to the NIOSH recommended exposure limit (REL). TLVs and RELs often are used for occupational screening purposes. The U.S. EPA has established regulatory benchmark values for many of the substances discussed in this chapter [e.g., reference dose (RfD), cancer slope factor (CSF), and reference concentration (RfC) for air]. The RfD is defined as “An estimate of a daily oral exposure for a given duration to the human population (including susceptible subgroups) that is likely to be without an appreciable risk of adverse health effects over a lifetime. It is derived from a BMDL, a NOAEL, a LOAEL, or another suitable point of departure, with uncertainty/variability factors applied to reflect limitations of the data used.” The oral CSF is defined as “An upper bound, approximating a 95% confidence limit, on the increased cancer risk from a lifetime oral exposure to an agent. This estimate, usually expressed in units of proportion (of a population) affected per mg/kg-day, is generally reserved for use in the low-dose region of the dose–response relationship, that is, for exposures corresponding to risks less than 1 in 100.” The RfC is “an estimate (with uncertainty spanning perhaps an order of magnitude) of a daily inhalation exposure of the human population (including sensitive subgroups) that is likely to be without an appreciable risk of deleterious effects during a lifetime.” Inhalation CSF values are available for some substances. These toxicological benchmarks often are used in calculating risk-based exposure targets and regional screening levels (RSLs) for solvents to be used in evaluation of risks posed by contaminated sites. They change periodically based on new information and can be acquired directly from online databases such as the U.S. EPA Integrated Risk Information System (IRIS). Table 18.4 Occupational Exposure Guideline Definitions As a point of comparison, Table 18.5 presents common ranges of concentrations encountered in environmental media and in occupational airborne circumstances. Table 18.5 Concentration Ranges for Selected Solvents and Related Substances in Environmental and Occupational Circumstances As noted, solvent exposure may occur via oral, dermal, and inhalation routes, as well as a combination of these. Absorption can occur from direct liquid contact, and abraded or cut skin may enhance dermal absorption. While dermal penetration of solvents typically is negligible at low air concentrations, the ACGIH and OSHA note that this route for some substances may be significant at high air concentrations, hence an occupational “skin” designation, applicable in confined spaces or areas where respiratory protection (e.g., use of air-purifying or air-supplied respirators) limits potential for inhalation. For example, exposure to 2-butoxyethanol vapor may cause dermal uptake that exceeds inhalation. Solvent absorption by the lungs is dependent on several factors, including air concentration, as well as ventilation rate, depth of respiration, and pulmonary circulation, all of which are influenced by workload. Once absorbed, solvents may be transported to other areas of the body, including organs where biotransformation may occur, resulting in formation of metabolites. Significant route-specific differences exist between uptake and the potential for adverse effects from solvents. Intake via the oral and dermal routes causes absorption into the venous circulation, which allows what is known as “first-pass” degradation or clearance by enzymatic process of the liver. Inhalation intake results in absorption via the alveoli into the arterial circulation, which distributes absorbed solvents to various locations prior to hepatic metabolism. For this reason, as well as the fact that airborne concentrations may be quite high in some cases, the inhalation route often is of greater toxicological concern on a strict numerical dose comparison. Many volatile solvents can be eliminated either as the parent compound or in metabolized form in exhaled breath, varying with workload. This can be independent of exposure route and is the basis for sampling expired air as a measure of occupational exposure (see Table 18.3). Less water-soluble substances (e.g., chlorinated solvents) may penetrate more deeply into the lungs. Given heterogeneity among solvents, numerous potential metabolic pathways exist, as described elsewhere in this book. The P450 enzyme system and the glutathione pathways often are involved, catalyzing oxidative reactions and conjugation to form water-soluble substances excretable in urine or bile. Several pathways may exist for the biotransformation of a specific solvent, depending on exposure route and concentration. Excretion of metabolites (e.g., s-phenylmercapturic acid from benzene, trichloroacetic acid from TCE, mandelic acid from styrene) forms the basis for biological monitoring programs in exposure characterization. Although the liver is the primary site of metabolism, other organs (e.g., kidney, lung) exhibit biotransformation capacity as well. Formation and accumulation of metabolites can occur if initial steps of biotransformation are present, but not later steps. For example, aldehydes may be metabolized readily in the liver, while the same aldehyde may accumulate in the lung and cause pulmonary damage due to limited aldehyde dehydrogenase. Beyond generally beneficial aspects of biotransformation (i.e., detoxification) and excretion, solvent metabolism occasionally may generate products that are more toxic than the parent compound. Such metabolic “activation,” also termed “bioactivation,” and resultant reactive intermediates (e.g., epoxides and radicals) are considered responsible for some toxic effects of solvents, especially with chronic exposure. This is illustrated by TCE, which exhibits variable metabolism in some rodents with dose, and that metabolism may produce novel toxicity elsewhere. Metabolic enzymes may be increased in activity (“induced”) by previous or concomitant chemical exposures, such as therapeutic drugs, foods, alcohol, cigarette smoke, and industrial exposures. Competitive interactions, which may affect enzyme activity for solvents in industrial scenarios, also may influence toxicity, such that exposure to multiple chemicals is not always worse than individual exposures (e.g., toluene inhibits benzene metabolism and toxicity). Saturation of normal metabolic degradation pathways may cause qualitative shifts in metabolism to other pathways. While a normal pathway may cause detoxification, saturation or overwhelming of the pathway may cause “shunting” to activation pathways (e.g., 1,1,1-trichloroethane, n-hexane, perchloroethylene (PERC), and 1,1-dichloroethene). Partition coefficients (Kp) for air–blood, fat–blood, and brain–blood can be calculated and used to describe differential solvent behavior in various tissues. Such values are useful to understand the preferential uptake and storage of solvents and to explain greater sensitivity of some organs to adverse effects. As discussed subsequently in greater detail by chemical class, solvents can have both acute, short-term effects based upon one or a few high-level exposures (e.g., irritation, neurological effects) and chronic effects that may be expressed in delayed fashion following prolonged exposures at lower levels (e.g., kidney disease, liver injury, cancer). Acute effects typically result from the rapid overwhelming of existing detoxification and excretion mechanisms, and they may be transient/reversible. Chronic effects often are related to repetitive, unrepaired, low-level damage that is accumulative over lengthy periods of exposure, and these effects are less likely to be reversible. The intermediate case, where more frequent or regular high-level exposures may occur, is difficult to predict, given individual sensitivities and resilience. For some solvents (e.g., aldehydes, ketones), the ability to detect odor or irritation at low air concentrations is a useful characteristic that can act as an “early warning” tool against acute and chronic exposure potential. However, for most industrially and environmentally important solvents (e.g., benzene, chlorohydrocarbons), odor does not serve as a sufficient warning property, and significant exposures can occur before they are detectable by smell. Avoidance of such exposure circumstances is a foundation of occupational and environmental monitoring programs. A common physiological effect associated with short- or long-term, high-level exposure to some organic solvents is depression of central nervous system (CNS) activity, causing general anesthesia, decreased brain/spinal cord activity, and lowered sensitivity to external stimuli, with unconsciousness or death as the severest consequence. Many solvents are lipophilic (“fat loving”), a feature typically coupled with a low affinity for water (hydrophobic). Those compounds tend to accumulate in lipid-rich areas, including blood, brain, and depot (storage) fats. Accumulation in or direct damage to nerve cells can disrupt normal nerve excitability and adversely affect nerve impulse conduction. While organic solvents with few or no functional groups generally are lipophilic and exhibit some limited degree of CNS-depressant activity, this property generally increases with carbon chain length. Changes in toxicity are most evident when larger functional groups are added to small organic compounds, since the increase in molecular size generally decreases water solubility and increases lipophilicity. In practical terms, this applies only to chemicals up to carbon chain length of about six. As molecular size increases beyond this point for a functional class (e.g., amines, alcohols, ethers), vapor pressure decreases and exposure issues (e.g., inhalation) decrease dramatically. Unsaturated chemicals (i.e., where hydrogens have been lost, forming double or triple bonds between carbon atoms) typically are more potent CNS-depressant chemicals than their saturated (i.e., single bond) counterparts. Similarly, CNS-depressant properties of organic solvents generally are enhanced by an increasing extent of halogenation (e.g., chlorine, bromine) and, to a lesser extent, by addition of alcohol (OH) groups. For example, while methane and ethane are gases, are not anesthetics, and act as simple asphyxiants at high concentrations, both of the corresponding alcohol analogs (i.e., methanol and ethanol) are liquids and are potent CNS depressants. Methylene chloride has anesthetic properties, but chloroform (CHCL3) is more potent than methylene chloride, and carbon tetrachloride (CCL4) is the most potent anesthetic of the group. Toluene encephalopathy has been reported in high-level inhalant abuse, characterized by severe neurotoxicity. Evidence is limited that such a syndrome occurs at low-level exposures and there is considerable doubt as to whether “solvent-induced chronic encephalopathy” exists in reasonable exposure circumstances. That syndrome, described in the late 1970s, was explored more fully in the 1980s, though recent reviews do not conclude that chronic low-level solvent exposure typically results in CNS or peripheral nervous system (PNS) injury. To illustrate the nonspecificity of solvent neurotoxic effects, and related problems that may be faced by a health specialist in trying to diagnose poorly characterized exposure situations, the following constellations of symptoms are described for a few common agents. It should be noted that, in contrast to these acute effects, effects of chronic exposure to these agents may differ dramatically, as discussed elsewhere in the chapter: The literature is clear that select organic solvents (e.g., n-hexane, methyl n-butyl ketone, carbon disulfide) can cause “distal axonal peripheral neuropathy,” also often termed “dying-back axonopathy” as defined by degradation of the axon or nerve cell body. It typically affects the feet and lower legs before the hands and may be partially reversible if identified sufficiently early in the process. The condition is associated with weakness, alteration or loss of sensation, impairment of reflexes, and eventual generalized muscle wasting. Occupational development of the condition has been reported in painters, automotive technicians, and workers in the shoe and furniture manufacturing sectors. Development of the condition is slow, though it may be accelerated in cases of inhalant abuse, and deterioration arguably may progress beyond the time at which exposure ceases, though the mechanism for such progression is not described. It is necessary to diagnose these disorders carefully while keeping in mind the possibility of other potential etiologies for the peripheral neurological observations (e.g., trauma, lead exposure, inflammation, diabetes, autoimmunity, heredity). Nephrotoxic effects have been associated with both acute and chronic exposures to halogenated hydrocarbons (e.g., chloroform, tetrachloroethylene). The primary target of chloroform is the proximal kidney tubule where metabolism via renal cellular P450 produces reactive intermediates that cause damage followed by protein and glucose leakage to the urine, as well as increased blood urea nitrogen (BUN) levels. PERC is one of a group of chemicals that cause serious sex-specific and species-specific α2-microglobulin nephropathy in animals. However, humans are not at risk of this particular nephropathy because we do not synthesize the α2-microglobulin protein. As described in subsequent sections, organic solvents known to damage the liver include ethanol and chlorinated hydrocarbons. Enzymes (e.g., P450) and other factors that affect solvent transformation play a role in determining the extent of hepatotoxicity associated with these and related chemicals. Factors that activate P450 increase toxicity of many chlorinated solvents, and those that inhibit (decrease) P450 activity tend to reduce toxicity. Coexposure to some alcohols and chlorinated solvents has been associated with exacerbation of toxicity compared with that expected from either agent alone. Cell membranes are composed principally of a protein–lipid matrix, and organic solvents may act to dissolve that matrix or extract the fat (lipid) portion from the membrane, causing membrane and tissue irritation. This “defatting” process, when applied to skin, causes drying, irritation, and cell damage. Injury to the lungs and eyes may be caused by similar processes. As described previously, addition of some functional groups to organic molecules predictably influences toxicological properties. For example, amines, organic acids, alcohols, aldehydes, and ketones may cause cell membrane damage by precipitation and denaturation of proteins following sufficient exposures. As is true for CNS depression, irritation by unsaturated compounds generally is stronger than for the corresponding saturated analogs. As molecular size increases, irritant properties typically decrease, and solvent defatting action of the hydrocarbon portion is altered. Table 18.6 presents relative potency of selected functional groups regarding CNS-depressant effects and irritation. The rankings become less applicable as the larger, more complex, multisubstituted compounds are considered. A number of solvents are inhalation irritants, though irritation often is restricted to the upper airways due to the high degree of water solubility of the substances and the tendency to dissolve in moisture of mucous membranes. As with toxicological evaluations of other potential solvent-related adverse effects, the complex nature of industrial exposure scenarios complicates the objective evaluation of malignancy attributed to a specific solvent. Thus, many occupational studies end up considering solvent exposure as a general “risk factor” for neoplasia but stop short of establishing “cause and effect” relationships. While in some occupational exposure circumstances it is possible to establish a causative element of human cancer risk for industrial activities (asbestos and mesothelioma in some shipyard employees; angiosarcoma in concentrated vinyl chloride exposure), such is not the case for most solvents. There is historical documentation for benzene as a human carcinogen under well-described intense exposure circumstances. Multiple factors may be responsible for the observed effects, but metabolism of benzene to one or more reactive metabolites (e.g., epoxides) is likely to be responsible for the myelotoxicity. A complementary hypothesis suggests that a depressant effect by benzene or its metabolites on cell-mediated immunity may influence the carcinogenic process. It is interesting that the substituted benzene analogs toluene and xylenes are noncarcinogenic, while styrene (or vinylbenzene) forms reactive metabolites, as does benzene, notably styrene oxide. Styrene recently was listed as reasonably anticipated to be a human carcinogen in the NTP 12th Report on Carcinogens. That listing was based on limited evidence in human studies, sufficient evidence in animals, and supporting data on carcinogenesis mechanisms. Some chlorinated solvents, such as carbon tetrachloride, chloroform, tetrachloroethylene (PERC), TCE (trichloroethylene), and vinyl chloride, exhibit carcinogenic potential, notably hepatic tumors, in animals. Carcinogenic potential associated with TCE exposure has been of interest since the mid-1970s, when the National Cancer Institute (NCI) reported increases in liver cancer in male mice receiving TCE by gastric intubation. TCE, like some other chlorinated hydrocarbons, exhibits limited, inconsistent, and controversial mutagenic activity in bacterial test systems after microsomal activation, so the mutagenic effect is likely dependent on the products of metabolism, which has influenced recent interest in actual TCE potency. In the recent 2011 Toxicological Review, the U.S. EPA characterizes TCE as “carcinogenic to humans” based upon procedures outlined in the U.S. EPA 2005 Guidelines for Carcinogen Risk Assessment. That classification relies on evidence of a putative causal association between TCE exposure in humans and kidney cancer, in addition to carcinogenicity in the liver and lymphoid tissues. Other groups (e.g., ACGIH) continue to classify TCE as A2 (“suspected human carcinogen”), while the International Agency for Research on Cancer (IARC) considers TCE in class 2A “probably carcinogenic to humans.” PERC is considered “likely to be a human carcinogen by all routes of exposure” as published in the 2012 Toxicological Review released by the U.S. EPA. Apart from leukemogenic effects associated with chronic benzene exposure, the literature available to document specific cancer hazards from exposure to organic solvents is inconsistent, though some epidemiological observations have been published regarding cancer and chlorinated solvent exposure. For example, Hodgkin’s lymphoma and NHL arguably have been linked to occupational exposure to some aliphatic, aromatic, and chlorinated solvents. Recently, a study of nearly 15,000 aircraft maintenance workers with exposure to TCE and other solvents reported a decrease in overall cancer mortality but a small excess in NHL, multiple myeloma, and bile duct cancer. The U.S. EPA inhalation unit risk and oral CSF for TCE were predicated partly on NHL risk. For most solvents, reproductive effects are not among the most sensitive measures of toxic effects. Reproductive toxicity has been reported following exposure to 2-methoxyethanol (2-ME), 2-ethoxyethanol (2EE), and n-hexane. 2-ME may damage the testes and cause infertility at extreme doses. It also has been shown to be teratogenic in animal studies, as has exposure to 2-bromopropane, carbon disulfide, and ethylene glycol monomethyl ether. Reproductive dysfunction was reported following exposure at high levels to toluene and other petrochemicals, though more sensitive measures of exposure occur at lower levels. Toluene abuse in pregnancy can lead to teratogenic effects labeled “fetal solvent syndrome.” Organic solvent exposure in pregnant women reportedly has been associated with poor cognitive development and neuromotor functioning in childhood, as well as low childhood birth weight. Retail products containing ethanol require federal warning labels directed at notification of potential birth defects and other elements of reproductive consequences. The chemical class of the saturated aliphatic hydrocarbons (alkanes, also termed paraffins) has many members and generally ranks among the least potentially toxic solvents based on acute effects. The group is comprised of straight chain or branched hydrocarbons containing only single bonds. Vapors of these solvents are mildly irritating to mucous membranes at the high concentrations required to induce their relatively weak anesthetic properties. The four chemicals in this series with the lowest molecular weight (i.e., methane, ethane, propane, butane) are gases with negligible toxicity, and their hazardous nature is limited almost entirely to flammability, explosivity, and basic asphyxiant potential. The chemicals are found in natural gas and can be released into the environment from the exhaust of gasoline and diesel engines, from municipal waste incinerators, and from many other combustion sources. Higher-molecular-weight class representatives are liquid at ambient temperature (i.e., 20–25 °C) and have some CNS-depressant, neurotoxic, and irritant properties, but this is primarily a concern of the lighter, more volatile compounds in the series (e.g., pentane, hexane, heptane, octane, nonane). Higher-viscosity liquid paraffins, beginning with the 10-carbon compound decane, are fat solvents and primary irritants capable of dermal irritation and dermatitis after repeated, prolonged, or intense contact. Many of these substances occur naturally in crude and refined petroleum products. Symptoms of acute poisoning are similar to those previously described as generally present in solvent intoxication (e.g., nausea, vomiting, cough, pulmonary irritation, vertigo/dizziness, slow and shallow respiration, narcosis, coma, convulsions, and death), with severity of symptoms dependent on the magnitude and duration of exposure. Ingestion of large liquid quantities (i.e., exceeding several ounces, about 1–2 ml/kg body weight) may produce systemic toxicity. If less than 1–2 ml/kg is ingested, the therapeutic approach typically involves a cathartic used in conjunction with activated charcoal to limit GI absorption. In either situation, pulmonary aspiration of the solvent is the primary concern from a medical perspective. Low-viscosity hydrocarbons attract particular attention in this context because their low surface tension allows them to spread over a large surface area, with the potential to damage the lungs after exposure to relatively small quantities. These chemicals may sensitize the heart to epinephrine (adrenaline), but that feature is not often a practical issue given the narrow range separating cardiac sensitization from fatal narcosis. Chronic exposure to some alkanes (e.g., hexane, heptane) may cause polyneuropathy in humans and animals, characterized by lowered nerve conduction velocity and a “dying-back” degenerative change in the distal neuron sheath. Symptoms may include muscle pain and spasms, weakness, and paresthesias, characterized by tingling or numbness in the extremities. Common metabolites have been implicated as the causative agents, with 2,5-hexanedione and 2,6-heptanedione as toxic degradation products of hexane and heptane, respectively. Since the metabolites are oxidative products, first to the alcohol and then to the diketone, there is concern that structurally similar alcohols and ketones may produce similar neuropathies compared to the parent aliphatic hydrocarbons. Alkanes generally are not considered carcinogenic.
PROPERTIES AND EFFECTS OF SOLVENTS AND SOLVENT-LIKE CHEMICALS
18.1 GENERAL CONSIDERATIONS
18.2 EXPOSURE POTENTIAL, MONITORING, AND RISK CONSIDERATIONS
Exposure Routes
Industrial Exposures
X ppm (or ppb)
= concentration in units of volume/volume
Y mg/m3 (or µg/m3)
= concentration in units of mass/volume
MW
= molecular weight of the chemical
24.45
= molar volume of an ideal gas at standard temperature and pressure
Household and Other Exposures
18.3 BASIC PRINCIPLES AND REVIEW OF TARGET ORGAN SYSTEMS
Chemical
CAS #
Molecular Formula
Molecular Weight (g/mole)
Freezing or Melting Point (°F)
Boiling Point (°F)
Vapor Pressure (20 °C) (mm Hg)
Water Solubility (20 °C) mg/l
Specific Gravity (unitless)
Halogenated
Carbon tetrachloride
56-23-5
CCl4
153.8
−9
170
91
500
1.59
Chloroform
67-66-3
CHCl3
119.4
−82
143
160
5,000 (25 °C)
1.48
Methylene chloride
75-09-2
CH2Cl2
84.9
−139
104
350
20,000
1.33
Tetrachloroethene
127-18-4
C2Cl4
165.8
−2
250
14
200
1.62
Trichloroethylene
79-01-6
C2HCl3
131.4
−99
189
58
1,000
1.46
Vinyl chloride
75-01-4
C2H3Cl
62.5
−256
7
2508
1,000 (25 °C)
2.21
Nonhalogenated
Acetaldehyde
75-07-0
C2H4O
44.1
−190
69
740
Miscible
0.79
Acetone
67-64-1
C3H6O
58.1
−140
133
180
Miscible
0.79
Acrolein
107-02-8
C3H4O
56.1
−126
127
210
400,000
0.84
Aniline
62-53-3
C6H7N
93.1
21
363
0.6
40,000
1.02
Benzene
71-43-2
C6H6
78.1
42
176
75
700
0.88
Benzidine
92-87-5
C12H12N2
184.3
239
752
low
400 (12 °C)
1.25
Carbon disulfide
75-15-0
CS2
76.1
−169
116
297
3,000
1.26
N,N-Dimethylaniline
121-69-7
C8H11N
121.2
36
378
1
20,000
0.96
1,4-Dioxane
123-91-1
C4H8O2
88.1
53
214
29
Miscible
1.03
Ethanol
64-17-5
C2H5OH
46.1
−173
173
44
Miscible
0.79
Ethyl acetate
141-78-6
C4H8O2
88.1
−117
171
73
100,000 (25 °C)
0.9
Ethyl ether
60-29-7
C4H10O
74.1
−177
94
440
80,000
0.71
Ethylene glycol
107-21-1
C2H6O2
62.1
9
388
0.06
Miscible
1.11
Formaldehyde
50-00-0
CH2O
30.0
−134
−6
>760
Miscible
1.04
n-Hexane
110-54-3
C6H14
86.2
−219
156
124
20
0.66
Hydrazine
302-01-2
N2H4
32.1
36
236
10
Miscible
1.01
Isopropanol
67-63-0
C3H8O
60.1
−127
181
33
Miscible
0.79
Isopropyl ether
108-20-3
C6H14O
102.2
−76
154
119
2,000
0.73
Methanol
67-56-1
CH4O
32.1
−144
147
96
Miscible
0.79
Methyl ethyl ketone
78-93-3
C4H8O
72.1
−123
175
78
280,000
0.81
Naphthalene
91-20-3
C10H8
128.2
176
424
0.08
30
1.15
Nitrobenzene
98-95-3
C6H5NO2
123.1
42
411
0.3 (25 °C)
2,000
1.2
Nitromethane
75-52-5
CH3NO2
61.0
−20
214
28
100,000
1.14
Phenol
108-95-2
C6H6O
94.1
109
359
0.4
90,000 (25 °C)
1.06
Pyridine
110-86-1
C5H5N
79.1
−44
240
16
Miscible
0.98
Styrene
100-42-5
C8H8
104.2
−23
293
5
300
0.91
Tetrahydrofuran
109-99-9
C4H8O
72.1
−163
151
132
Miscible
0.89
Toluene
108-88-3
C7H8
92.1
−139
232
21
700 (23.3 °C)
0.87
ACGIH TLV (ppm)
OSHA PEL (ppm)
U.S. EPA Oral
Carcinogen Classification
Compound
CAS #
TWA
STEL/Ceiling
TWA
STEL/Ceiling
RfD (mg/kg-day)
ACGIH
U.S. EPA
NTP
Acetaldehyde
75-07-0
NE
25
200
NE
NE
A2
B2
R
Acetone
67-64-1
250 (NIC)
500 (NIC)
1000
NE
0.9
A4
I
NE
Acrolein
107-02-8
NE
0.1
0.1
NE
0.0005
A4
I
NE
Aniline
62-53-3
2
NE
5
NE
NE
A3
B2
NE
Benzene
71-43-2
0.5
2.5
1
5
0.004
A1
A
K
Benzidine
92-87-5
NE
NE
NE
NE
0.003
A1
A
K
Carbon disulfide
75-15-0
1
NE
20
30
0.1
A4
NE
NE
Carbon tetrachloride
56-23-5
5
10
10
25
0.004
A2
L
R
Chloroform
67-66-3
10
NE
NE
50
0.01
A3
B2;L (acute); NL (low dose)
R
N,N-Dimethylaniline
121-69-7
5
10
5
NE
0.002
A4
NE
NE
1,4-Dioxane
123-91-1
20
NE
100
NE
0.03
A3
L
R
Ethanol
64-17-5
NE
1000
1000
NE
NE
A3
NE
K
Ethyl acetate
141-78-6
400
NE
400
NE
0.9
NE
NE
NE
Ethyl ether
60-29-7
400
500
400
NE
NE
NE
NE
NE
Ethylene glycol
107-21-1
10 (NIC)
100 (NIC)
NE
NE
2
A4
NE
NE
Formaldehyde
50-00-0
NE
0.3
0.75
2
0.2
A2
B1
K
n-Hexane
110-54-3
50
NE
500
NE
0.7
NE
II
NE
Hydrazine
302-01-2
0.01
NE
1
NE
NE
A3
B2
R
Isopropanol
67-63-0
200
400
400
NE
NE
A4
NE
NE
Isopropyl ether
108-20-3
250
310
500
NE
NE
NE
NE
NE
Methanol
67-56-1
200
250
200
NE
2
NE
NE
NE
Methylene chloride
75-09-2
50
NE
25
125
0.006
A3
L
R
Methyl ethyl ketone
78-93-3
200
300
200
NE
0.6
NE
I
NE
Naphthalene
91-20-3
10
NE
10
NE
0.02
A3
CBD
R
Nitrobenzene
98-95-3
1
NE
1
NE
0.002
A3
L
R
Nitromethane
75-52-5
20
NE
100
NE
NE
A3
NE
R
Phenol
108-95-2
5
NE
5
NE
NE
A4
I;D
NE
Pyridine
110-86-1
1
NE
5
NE
0.001
A3
NE
NE
Styrene
100-42-5
20
40
100
200
0.2
A4
NE
R
Tetrachloroethylene
127-18-4
25
100
100
200
0.006
A3
L
R
Tetrahydrofuran
109-99-9
50
100
200
NE
0.9
A3
S
NE
Toluene
108-88-3
20
NE
200
300
NE
A4
II
NE
Trichloroethylene
79-01-6
10
25
100
200
0.0005
A2
CaH
R
Vinyl chloride
75-01-4
1
NE
1
5
0.003
A1
A
K
Compound
CAS #
Recommended BEI
Sampling Medium
Sampling Time
ACGIH Notation
Acetone
67-64-1
50 mg/l
Urine
End of shift
Ns
Aniline
62-53-3
1 mg/l (tentative)
Urine
End of shift
Nq
Nonquantitative
Released from hemoglobin
End of shift
Nq
50 mg/l
p-Aminophenola in urine
End of shift
Ns, Sq, B
Benzene
71-43-2
25 µg/g creatinine
S-Phenylmercapturic acid in urine
End of shift
B
500 µg/g creatinine
t,t-Muconic acid in urine
End of shift
B
2-Butoxyethanol
111-76-2
200 mg/g creatinine
Butoxyacetic acid (BAA) in urine
End of shift
—
Carbon disulfide
75-15-0
0.5 mg/g creatinine
2-Thioxothiazolidine-4-carboxylic acid (TTCA) in urine
End of shift
Ns, B
Chlorobenzene
108-90-7
100 mg/g creatinine
Total 4-chlorocatechol in urineb
End of shift at end of workweek
Ns
20 mg/g creatinine
Total p-chlorophenol in urineb
End of shift at end of workweek
Ns
2-Ethoxyethanol
110-80-5
100 mg/g creatinine
2-Ethoxyacetic acid in urine
End of shift at end of workweek
—
n-Hexane
110-54-3
0.4 mg/l
2,5-Hexanedionec in urine
End of shift at end of workweek
—
Methanol
67-56-1
15 mg/l
Urine
End of shift
B, Ns
2-Methoxyethanol
109-86-4
Nonquantitative
2-Methoxyacetic acid in urine
End of shift at end of workweek
Nq
Methylene chloride
75-09-2
0.3 mg/l
Urine
End of shift
Sq
Methyl ethyl ketone
78-93-3
2 mg/l
Urine
End of shift
Ns
Methyl n-butyl ketone
591-78-6
0.4 mg/l
2,5-Hexanedionec in urine
End of shift at end of workweek
—
Methyl isobutyl ketone
108-10-1
1 mg/l
Urine
End of shift
—
Nitrobenzene
98-95-3
5 mg/g creatinine
Total p-nitrophenol in urine
End of shift at end of workweek
Ns
1.5% hemoglobin
Methemoglobin in blood
End of shift
B, Ns, Sq
Phenol
108-95-2
250 mg/g creatinine
Urine
End of shift
B, Ns
2-Propanol
67-63-0
40 mg/l
Acetone in urine
End of shift at end of workweek
Ns, B
Styrene
100-42-5
400 mg/g creatinine
Mandelic acid plus phenylglyoxylic acid in urine
End of shift
Ns
0.2 mg/l
Venous blood
End of shift
Sq
Tetrachloroethylene
127-18-4
3 ppm
End-exhaled air
Prior to shift
—
0.5 mg/l
Blood
Prior to shift
—
Toluene
108-88-3
0.02 mg/l
Blood
Prior to last shift of workweek
—
0.03 mg/l
Urine
End of shift
—
0.3 mg/g creatinine
o-Cresol in urinea
End of shift
B
1,1,1-Trichloroethane
71-55-6
40 ppm
End-exhaled air
Prior to last shift of workweek
—
10 mg/l
Trichloroacetic acid in urine
End of workweek
Ns, Sq
30 mg/l
Total trichloroethanol in urine
End of shift at end of workweek
Ns, Sq
1 mg/l
Total trichloroethanol in blood
End of shift at end of workweek
Ns
Trichloroethylene
79-01-6
15 mg/l
Trichloroacetic acid in urine
End of shift at end of workweek
Ns
0.5 mg/l
Trichloroethanold in blood
End of shift at end of workweek
Ns
Screening only
Blood
End of shift at end of workweek
Sq
Screening only
End-exhaled air
End of shift at end of workweek
Sq
Xylenes
1330-20-7
1.5 g/g creatinine
Methylhippuric acids in urine
End of shift
—
ACGIH—American Conference of Governmental Industrial Hygienists (ACGIH, 2011)
TLV–TWA
Threshold limit value–time-weighted average. Time-weighted average concentration for a normal 8 h workday and a 40 h workweek, to which nearly all workers may be repeatedly exposed, day after day, without adverse effects
STEL
Short-term exposure limit. Defined as 15 min TWA exposure not to be exceeded during a workday. Concentration to which workers can be exposed continuously for a short period without suffering irritation, chronic/irreversible tissue damage, or narcosis to increase likelihood of injury, impair self-rescue, or materially reduce work efficiency
Categories for Carcinogenic Potential
A1
Confirmed human carcinogen
A2
Suspected human carcinogen
A3
Animal carcinogen
A4
Not classifiable as a human carcinogen
A5
Not suspected as a human carcinogen
OSHA—Occupational Safety and Health Administration (ACGIH, 2011)
PEL–TWA
Permissible exposure limit–time-weighted average. Concentration not to be exceeded during any 8 h work shift of a 40 h workweek
C
Ceiling concentration not to be exceeded any part of workday; if instantaneous monitoring not feasible, ceiling assessed as 15 min TWA
U.S. EPA—United States Environmental Protection Agency
Cancer Classification (1986–1996)
A
Known human carcinogen
B1
Probable human carcinogen. Based on human data
B2
Probable human carcinogen. Based on animal data
C
Possible human carcinogen
D
Not classifiable as to human carcinogenicity
Guidelines for Carcinogen Risk Assessment (Final in 2005)
CaH
Carcinogenic to humans
L
Likely to be carcinogenic to humans
S
Suggestive evidence of carcinogenic potential
I or II
Inadequate information to assess carcinogenic
NL
Not likely to be carcinogenic to humans
Chemical Class
Compound
CAS #
Common Occupational Exposure Range
Common Environmental Exposure Range: Air
Common Environmental Exposure Range: Water
Alkanes
n-Hexane
110-54-3
3–200 ppm
<50 ppb outdoor
Low; BDL, 10 µg/l
<10 ppb indoor
Aromatic hydrocarbons—monocyclic
Benzene
71-43-2
0.05–83 ppb
0.02–34 ppb
Moderate; BDL, 100 µg/l
Styrene
100-42-5
6.9–51 ppm
0.07–11.5 ppb indoor air
Low; BDL, 20 µg/l
0.06–4.6 ppb outdoor air
Toluene
108-88-3
5–50 ppm
8 ppb indoor air
High; BDL, 1000 µg/l
Xylenes
1330-20-7
0.5–1300 ppb
1–30 ppb outdoor air
High; BDL, 1000 µg/l
1–10 ppb indoor air
Aromatic hydrocarbons—polycyclic
Naphthalene
1.9–3.7 ppb
0.2 ppb or less outdoor air
Moderate; BDL, 200 µg/l
<1 ppb indoor air
Aliphatics—halogenated
Methylene chloride
75-09-2
1–1000 ppm
<1–11 ppb outdoor
Moderate; BDL, 200 µg/l
Tetrachloroethylene
127-18-4
16.9–48.4 ppm
<10 ppb outdoor air
Moderate; BDL, 200 µg/l
<15 ppb indoor air
74–428 ppb indoor air, newly dry-cleaned clothes
1,1,1-Trichloroethane
71-55-6
Up to 1300 ppm
0.1–1 ppb outdoor air
Low; BDL, 200 µg/l
0.3–4.4 ppb indoor air
Trichloroethylene
79-01-6
50–100 ppm
0.08–12 ppb outdoor air
High; BDL, 1000 µg/l
<5 ppb indoor air
Aromatics—halogenated
Chlorobenzene
108-90-7
Up to 4 ppm
<1 ppb outdoor air
Moderate; BDL, 100 µg/l
Alcohols
Phenol
108-95-2
0.03–3.2 ppm
0.5–44 ppb outdoor air
Low; BDL, 1–20 µg/l
Aldehydes, ketones
Acetone
67-64-1
1.5–166 ppm
7 ppb outdoor air
Moderate; BDL, 100 µg/l
8 ppb indoor air
Methyl ethyl ketone
78-93-3
0.3–11 ppm
<10 ppb outdoor air
Low; BDL, 20 µg/l
Esters, ethers, epoxides
2-Butoxyethanol
111-76-2
0.1–169 ppm
<10 ppb outdoor air
Low; BDL, 10 µg/l
Amines—aromatic
Aniline
62-53-3
<2 ppm
1–5 ppb indoor air
Low; BDL, 10 µg/l
Nitro compounds
Nitrobenzene
98-95-3
1 ppm
0.01–5.7 ppb outdoor air
Low; BDL, 20 µg/l
Sulfur-containing compounds
Carbon disulfide
75-15-0
1–47 ppm
<10 ppb outdoor air
Low; BDL, 20 µg/l
Absorption, Distribution, Metabolism, and Excretion
Acute versus Chronic Effects
Central Nervous System Effects
PNS Effects
Renal and Hepatic Effects
Irritation of Tissues and Membranes
Cancer
Reproductive Effects
18.4 TOXIC PROPERTIES OF REPRESENTATIVE ALIPHATIC AND ALICYCLIC HYDROCARBON SOLVENTS
Aliphatic Solvents
Alkanes (CnH2n + 2 )
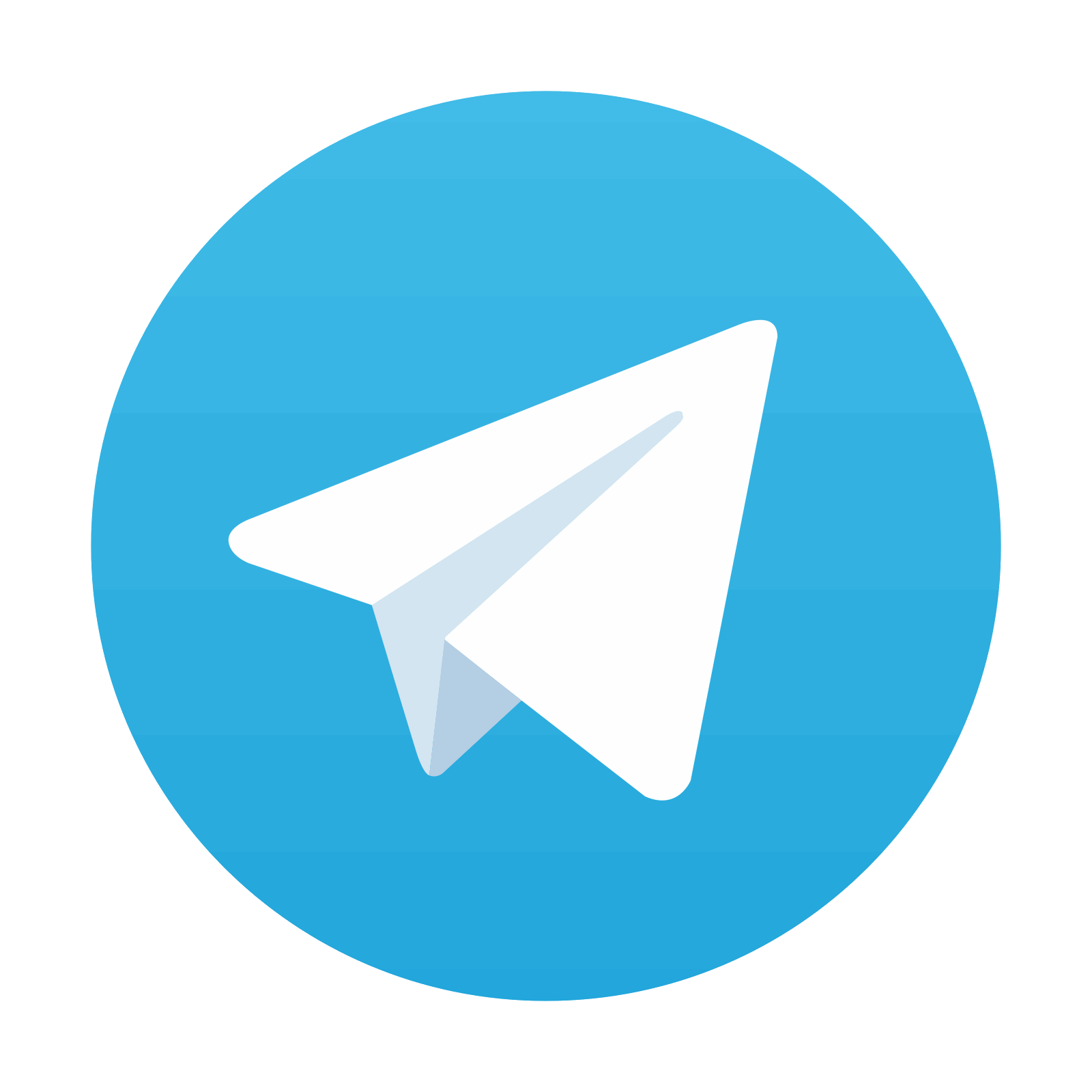
Stay updated, free articles. Join our Telegram channel

Full access? Get Clinical Tree
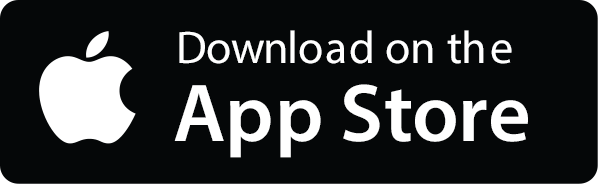
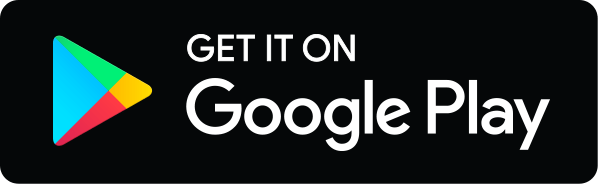