chapter 11 Prokaryotes, viruses and eukaryotes







Life on our planet can be relatively simple or incredibly complex. This chapter explores the likely processes that were needed for life to start, evolve and prosper, and then look at how scientists have attempted to categorise and classify the many forms of life to study the evolutionary relationships between the many diverse forms of life.
How did life start?
The Earth is thought to have formed about 4.5 billion years ago and was initially devoid of life. There appears to be evidence of bacteria-like organisms in rocks laid down approximately 3.5 billion years ago. If these microfossils are actually very early prokaryotes (see below) then it appears that life must have started within the first billion years of the Earth’s existence. The move from a lifeless planet to one now teeming with life is thought to have occurred through a series of major phases (Fig 11-1). Initially, conditions on the primordial Earth were very harsh but were ideal for spontaneous reactions between hydrogen, carbon and nitrogen to occur, leading to the production of ammonia and methane and, later, more complex organic molecules. Eventually the conditions moderated to allow large volumes of liquid water to exist, giving a medium in which reactions between these more complex organic molecules could occur spontaneously. The initial absence of oxygen in the primitive atmosphere was advantageous in that it allowed the newly-formed molecules to be more stable since these reductive conditions permitted large quantities of these molecules to build up, and because oxidation is often deleterious to biological molecules. This led to the development of a non-living ‘primordial soup’ rich in organic molecules.
The next key step was to condense these molecules to give macromolecules. It is believed that over hundreds of millions of years the amino acids and other organic molecules originally produced in the prebiotic stage of the Earth’s existence condensed to give simple proteins, phospholipids and nucleic acids. These molecules became sequestered in membrane-bounded vesicles to generate protocells. Gradually the chemical reactions occurring in the protocells became sufficiently organised for their transition to what can be considered as the first ‘living cells’. Two important facets for this transition were gaining the ability to: (1) capture and harness energy from the environment so that they could carry out synthetic reactions (see Ch 17); and (2) store, replicate and utilise information (see Chs 20 and 21) to make proteins, which became the cellular catalysts to help reactions occur more easily. The appearance of living cells led to an alteration in the driving force behind the changes occurring. Initially, change was driven purely by chemical reactions occurring spontaneously whereas the development of living cells enabled them to pass on a biological blueprint to offspring (see Ch 22), beginning the process of biological evolution—the change in the inherited traits of organisms through successive generations.
Biological evolution, in turn, led to the appearance of all the major features of cellular life. The driving force of evolution is natural selection of advantageous traits. In other words, when a particular organism acquires a novel characteristic that offers it an advantage over those that lack it, that organism reproduces more efficiently. Eventually that advantageous trait becomes common in a population of organisms. Of particular importance in evolutionary terms was the development of a nuclear region to store information as DNA, and systems to copy the DNA, and convert the information it carried to RNA for use in protein synthesis (see Ch 20).
Another important step was the development of ribosomes and the associated enzymes needed to make proteins. To do this, the cells also needed an oxidative system to supply chemical energy for use in protein synthesis and other synthetic reactions occurring in the cells. Later cell division developed to allow an increase in cell number in a manner that evenly distributed the information stored in the DNA to all the daughter cells. The membrane bounding the cell also gradually gained functionality so that it could control the movement of molecules into, and out of, the cell. All of these developments probably occurred in the first billion years after the Earth’s formation. These earliest cells were probably very simple prokaryotes (see below).
It is believed that eukaryotes developed because of associations between early prokaryotes. For example some organelles such as mitochondria and chloroplasts may have originated from endosymbiotic relationships between two prokaryotic cells. The endosymbiotic theory hypothesises that mitochondria may have developed when photosynthetic and non-photosynthetic prokaryotes coexisted in an oxygen-rich atmosphere. These non-photosynthetic prokaryotes fed themselves by ingesting organic material, which probably included other cells, from their immediate environment (Fig 11-2). This made them the earliest predators. These predatory prokaryotes probably included both anaerobes (organisms that do not require oxygen for growth, can react negatively or may even die in its presence) that could not utilise oxygen in energy production and were therefore unable to fully capture their inherent energy, and aerobes (organisms that can survive and grow in an oxygenated environment). It is believed that among the cells that were ingested were some aerobic cells, which instead of being digested, persisted in the predatory cells. These persistent aerobes became endosymbionts, living symbiotically within the other cell.
The main system of classification used by scientists today groups all living organisms into three domains (see below, Table 11-1 and Fig 11-6). One of these domains, the Eukarya, contains all the eukaryotes. The second domain, the Bacteria, contains both photosynthetic and non-photosynthetic prokaryotic bacteria. The final domain, the Archaea, contains bacteria-like prokaryotic organisms that inhabit extreme environments such as hot springs and thermal vents in the deep ocean. All three domains share common fundamental characteristics; they use the same genetic code, and DNA and RNA molecules carry out the same basic functions. This makes it likely that they all evolved from a common ancestral cell line. How Archaea fit into the evolution of both bacteria and eukaryotes has yet to be elucidated since they share characteristics of both groups of organisms.
The evolution of multicellular life
The first eukaryotes were unicellular organisms but later gave rise to multicellular versions. Molecular analysis of modern eukaryotes suggests that the first multicellular eukaryotes appeared about 900–1000 million years ago, and there is evidence in the fossil record of such organisms around 600–800 million years ago. It is thought that multicellular eukaryotes initially arose through cells of the same type congregating into a colony (Fig 11-3). Later the cells gained the ability to act in a coordinated manner such that these colonies were better able to adapt to environmental changes. Subsequently, cells within the colonies differentiated into specialised cell types with diverse but distinct functions. This gave these colonies a wider range of capabilities and adaptability. Over time the division of function among cells led to the evolution of the tissues and organs of complex eukaryotes.
Living organisms: classification and naming
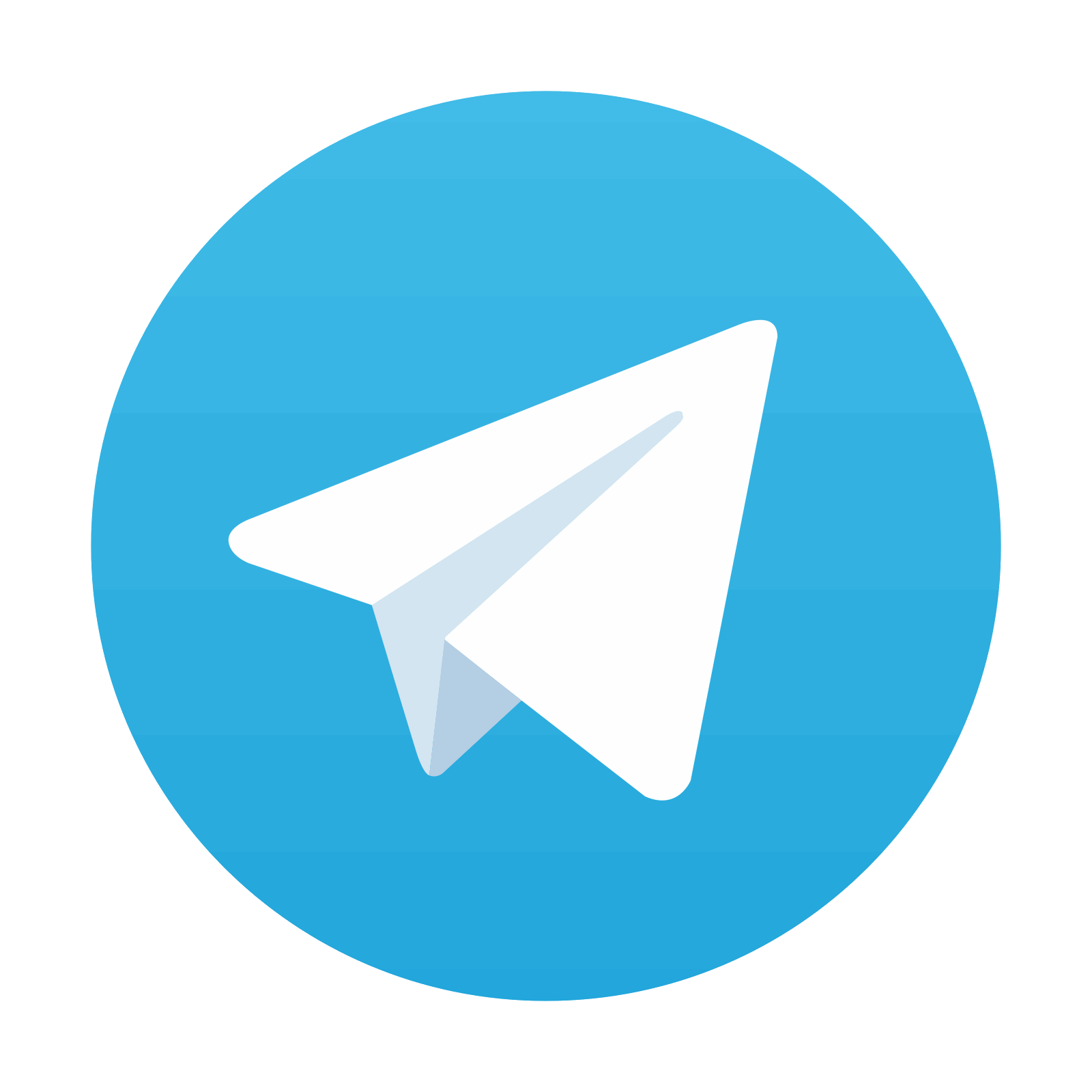
Stay updated, free articles. Join our Telegram channel

Full access? Get Clinical Tree
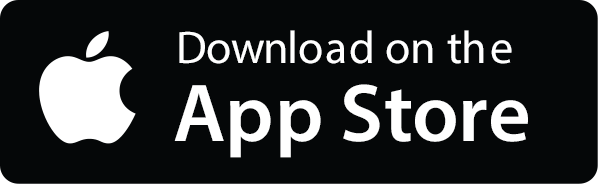
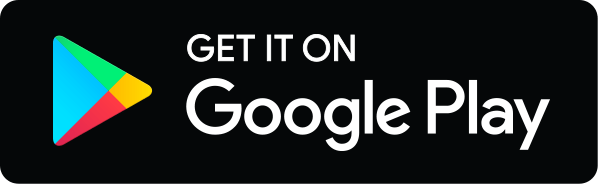