Art Meisch
CONTENTS
Role of Process Engineering in the Pharmaceutical Industry
Relationship of Process Engineering to Other Design Disciplines
Impact of cGMPs on Process Engineering
Historical Perspectives on Processing in the Pharmaceutical Industry
Typical Processing Technologies
Liquid and Semisolid Dosage Forms
Evaporation and Crystallization
Interactions with the Facility and Support Systems
Trends and Future Developments
Changes over the Past 10 Years
Expected Changes in the Next 10 Years
Environmental Health and Safety
INTRODUCTION
ROLE OF PROCESS ENGINEERING IN THE PHARMACEUTICAL INDUSTRY
Process engineering forms the bridge between the underlying sciences of chemistry, biology, and pharmacology and manufacturing operations. The process engineer translates the basic science and technology of the process steps into a commercially feasible production process. This task includes scaling up unit operations and converting them into the sizing, specification, and selection of the production equipment systems. These systems must meet the required production capacity for the selected products, while simultaneously meeting the constraints of capital and operating costs. The process engineer must also consider current Good Manufacturing Practice (cGMP) regulations, safety, and environmental issues.
RELATIONSHIP OF PROCESS ENGINEERING TO OTHER DESIGN DISCIPLINES
Every aspect of the pharmaceutical manufacturing facility is focused on supporting the process operation and allowing it to function as intended. The design of these facilities is a team effort; typical teams are comprised of process; instrumentation and control system; mechanical, civil or structural, and electrical engineers; architects; manufacturing personnel; validation and quality operations personnel; and frequently, scientists and engineers from research and development. A key responsibility of the process engineer is to communicate the processing system’s requirements to the other design team members so that they can design a facility that achieves the production objectives.
IMPACT OF CGMPS ON PROCESS ENGINEERING
The cGMPs require that the production processes manufacture products that consistently meet quality, efficacy, and stability requirements. A well-documented scientific basis for process operations ensures that when they are carried out under the documented conditions, the correct drug results.
The process and the facility are designed to prevent both trace contamination and cross-contamination of the drug products. Typical sources of trace contamination are water used in production, equipment and piping systems, and environmental particulates. The responsibility of the process engineer is to specify and design process equipment and piping systems that will prevent contamination and can be easily cleaned, as well as to establish safe environmental conditions within the manufacturing facility to protect the product. The International Society of Pharmaceutical Engineering (ISPE) Baseline Pharmaceutical Guides provide an excellent resource for identifying and addressing cGMP issues.
EXECUTIVE SUMMARY
HISTORICAL PERSPECTIVES ON PROCESSING IN THE PHARMACEUTICAL INDUSTRY
Many of the earliest pharmaceutical chemicals were extracted by the individual user from natural substances (e.g., willow leaves and bark yielded molecules similar to those of acetylsalicylic acid or aspirin). Early manufacturing efforts also extracted pharmacologically active chemicals from plants and animal tissues. Digitalis, for example, is still extracted from plants commercially. Animals were used to produce some of the first vaccines and antibiotics; for example, cows were used to make the smallpox vaccine. Of course, the use of genetically engineered plants and animals, both multicellular and single cellular, is at the forefront of today’s technology for both biologicals and even synthetic pharmaceuticals. Beginning in the late 1800s, chemists began to develop methods to produce naturally occurring chemicals synthetically. Aspirin, for example, was first synthetically manufactured in the 1800s from coal tar. The trend of using chemical reactions to manufacture pharmaceuticals grew throughout the 1900s, especially after World War II, to become the production method of choice for most active pharmaceutical ingredients (APIs). Fermentation has been used since the 1940s to produce antibiotics, and biotechnology has been used with increasing prevalence to produce more targeted molecules since the 1980s. Today, a combination of chemically produced “small molecules” attached to biologically produced “large molecules” is becoming common.
TYPICAL PROCESSING TECHNOLOGIES
Pharmaceuticals are chemicals with health effects that interact with living animals or humans. Production of pharmaceuticals depends on chemical synthesis, extraction from natural material, biological processing, or a combination of these processes. After the pharmaceutical chemicals are produced, they must be formulated for human use, which entails delivery targeted to a particular area of the body and absorption of the appropriate dose. The primary delivery methods include oral (i.e., solid or liquid), topical, inhalant, and injectable.
PHARMACEUTICAL PROCESSES
This section discusses the primary processes used in the production of pharmaceuticals. The dosage forms are discussed first, followed by a discussion of the processes used to manufacture the APIs found in the final dosage forms.
DOSAGE FORM PROCESSING
Drug products are administered in oral solid, oral liquid, topical, inhalant, and injectable forms. Oral solids comprise the largest volume of drug products. Below is a brief overview of the key processing steps and equipment used for each dosage form. For all, the starting point in the process is the API, produced by chemical synthesis or biological processing. Generally, dosage form processing focuses on bringing about physical, not chemical, changes.
Oral Solid Dosage Forms
The fundamental process steps in oral solid dosage forms include dispensing, granulation, drying, milling, blending, tableting, coating, encapsulation, and packaging (Figure 8.1).
Dispensing is the accurate weighing of the solid and liquid ingredients that constitute the dosage form; these include APIs, excipients, lubricants, disintegrants, and coatings. As corrosion is not a major concern, dosage form equipment is generally fabricated from 316L stainless steel. Containment of dust, however, is a major issue throughout solid dosage form processing, starting with the dispensing operation. High-potency APIs may be handled in isolators, downflow booths, or exhaust hoods; nonpotent materials are generally handled with exhaust hoods or downflow booths.
Once dispensed, the finely divided powders are granulated to form a larger particle (agglomerate) that contains a uniform concentration of all of the constituent solids. Granulations are often created with a liquid to aide in agglomeration, although for some products, dry granulation (without a liquid) is possible. This liquid may be United States Pharmacopoeia (USP) water, or it may be a flammable solvent, depending on the final product. Since most APIs dissolve in water, the amount of liquid used in granulation is very small and is added while the solids are blended. Granulations are performed in a wide range of equipment, including rotating blenders, agitated stationary blenders, and fluid-bed processors.
FIGURE 8.1 Oral solid dosage form process flowchart.
After a wet granulation is formed, the liquid must be removed by drying. If a fluid-bed processor or a jacketed blender is used, then drying is done in the same equipment. Tray- or truck-drying ovens are still used; however, they are becoming less popular because they require extensive manual handling and because potent materials are difficult to contain during processing. Microwave drying is used with highly potent compounds to provide contained drying as part of a high-shear granulator (see below).
The dried granulation is milled with limited energy input to produce a uniform particle size for tableting operations. There is a trend toward “single-pot processing” for potent compounds, in which granulation, drying, and milling are performed in a single integrated equipment train. After milling, the granulated materials are blended to develop a uniform concentration. Blending can take place in an intermediate bulk container (IBC) or in a fixed piece of equipment (e.g., a V-blender or twin-shell blender). The blended material is usually transported in an IBC to the tablet press, where the final tablet is formed. Tablet presses are very complicated machines that depend on uniform flow properties of the granulation to produce the uniform composition of tablets (Figures 8.2 and 8.3).
Often, many of the ingredients in the blend are included to allow the tablet press to perform its function consistently. Because of this complexity, small-volume products are sometimes produced as capsules, which simplifies the granulation steps. The tablet is usually coated, either in a coating pan or in a fluid-bed coater. Coating solutions can be aqueous or solvent based, with some tablets requiring more than one coating step. Some coatings (e.g., enteric coatings) contain a different API from the tablet itself to provide an initial pharmacological effect before the tablet disintegrates in the digestive tract. Coating solutions are prepared in jacketed, agitated tanks. The solution is usually heated slightly to promote dissolution of the solid ingredients and then cooled to room temperature before being added to the coater. Tablet coaters use large volumes of filtered, conditioned air to dry the coated tablets. Occasionally, when flammable solvents are used, nitrogen is used in place of air in the coating operation. Because of the large-volume requirements and process economics, the nitrogen is normally recycled after the solvents and dust particles are removed. In general, large, sophisticated air (or nitrogen) handling systems are required to support each coating pan or fluid-bed unit. Coated tablets are printed with the manufacturer’s product information and then packaged (Figure 8.4).
FIGURE 8.2 Fluid bed. (From Glatt Air Techniques, Inc.)
FIGURE 8.3 Tablet press. (From Korsch America, Inc.)
Liquid and Semisolid Dosage Forms
This broad category includes oral liquid, topical, inhalant, and injectable dosage forms. While there are significant differences in facility design for oral liquids and topicals compared to inhalants and injectables, the basic process unit operations are similar. Most of these product types start with dispensing and then proceed to a liquid-phase blending step, using a jacketed, agitated vessel. After the blending step, the product containers are filled and then packaged.
FIGURE 8.4 Coating pan. (From Glatt Air Techniques, Inc.)
Since the API is normally a solid, the same dispensing issues exist as discussed above for oral solid dosage forms. For oral liquids, the API is usually blended in either ethanol, which is flammable, or USP purified water. Most oral liquids are blended at ambient temperature. Topical dosage forms range from low-viscosity liquids to moderate-viscosity lotions to high-viscosity creams and ointments. Lotions, creams, and ointments frequently are emulsions formed by intense agitation of two distinct liquid phases—one aqueous based and the other oil based. Each liquid phase is first prepared in separate jacketed, agitated vessels by dissolving the required solid ingredients in water or oil while heating (to aid dissolution). After each liquid phase is prepared, both the water and oil phases are combined, using intense agitation (a homogenizer) to disperse the phases and form a stable emulsion. Containers for highly viscous topicals are filled at elevated temperatures to improve flow during filling.
Injectables must be sterile, as they directly enter the body, bypassing the protection offered by the digestive tract. Therefore, while the actual process steps for injectables are relatively simple, those that ensure that the product is sterile and stable are not. The product, either liquid or solid, is usually filled into small glass or plastic containers. Since most injectables are water based, the processing starts by dissolving the API in water for injection (WFI). After this formulation is prepared, it is normally filtered through 0.2-micron filters to ensure sterility, before filling a vial, syringe, or other container. If the API can tolerate the heat, then the filled, stoppered containers are steam sterilized (terminal sterilization). Containers of injectable liquids that cannot be terminally sterilized must be filled under aseptic conditions. Many injectable products are then dried after filling, using a vacuum freeze-drying process called lyophilization. Vials and all items that come in contact with the sterile product must also be sterile. Chapter 11 provides further discussion on sterile facilities.
Inhalants, like injectables, bypass the digestive tract. They must have a low bioburden but may not need to be sterile. Inhalants require the means to provide a dose of a fixed, repeatable size (metered dose), as well as the means to propel the dose into the throat. Most commonly, a solution or a suspension of the API is prepared in a liquid, which is then placed into the dosage container and a propellant added to pressurize the container. When used with an engineered nozzle, this assembly provides consistent doses of the API. Processing starts with dispensing the API and any other ingredients, and then adding the API to a liquid to form a solution or a uniform suspension. If water is used, it is USP purified or, in some cases, WFI, to reduce the bioburden. After the blending step, the liquid is filtered prior to filling containers. The use of dry powder inhalants, rather than the liquid solution or suspension, has grown considerably over the past 15 years.
PROCESSING OF APIS
APIs are produced primarily by chemical synthesis, biological processing, or a combination of both. Extraction of natural materials, from either plants or animals, can be completed by one or both of these processes. The paragraph below describes chemical synthesis, and Chapter 12 provides a discussion of biological processing and the facilities in which it occurs (Figure 8.5).
Chemical synthesis describes a series of chemical reactions that produce the API; these chemical reactions are accompanied by a number of other unit operations, which separate and purify the final API. The primary chemical synthesis unit operations are reaction, heat transfer, extraction, distillation, evaporation, crystallization, filtration, drying, and size reduction. Most chemically derived APIs complete the initial reaction in a liquid phase in organic solvents; they are then solidified, separated from the solvent and other impurities by filtration, and finally dried under vacuum to remove the last traces of solvent. The dried API is then milled to reduce its particle size range for formulation into the final dosage form. Pharmaceutical plants that produce APIs require multiproduct, flexible equipment trains. A brief discussion of the unit operations and the equipment commonly used at these plants follows (Figures 8.6 and 8.7).
Reactions
Most reactions are liquid-phase batch reactions, carried out in a pressure vessel with an agitator and an external jacket. The final API frequently requires from 3 to 10 separate reaction steps, depending on the complexity of the API molecule and on the commercially available intermediate chemicals. Each of these reaction steps usually requires separation and some purification. The early steps also generally require a greater volume of reaction materials, and therefore a larger reactor than later steps; for example, in these multistep processes, it is not uncommon for the first step to take place in a reactor four to five times the size of the final-step reactor. Typical reactor volumes used for production processes range from 500 to 5,000 gallons. Research and development reactors generally range from 5 to 500 gallons.
FIGURE 8.5 API process flowchart.
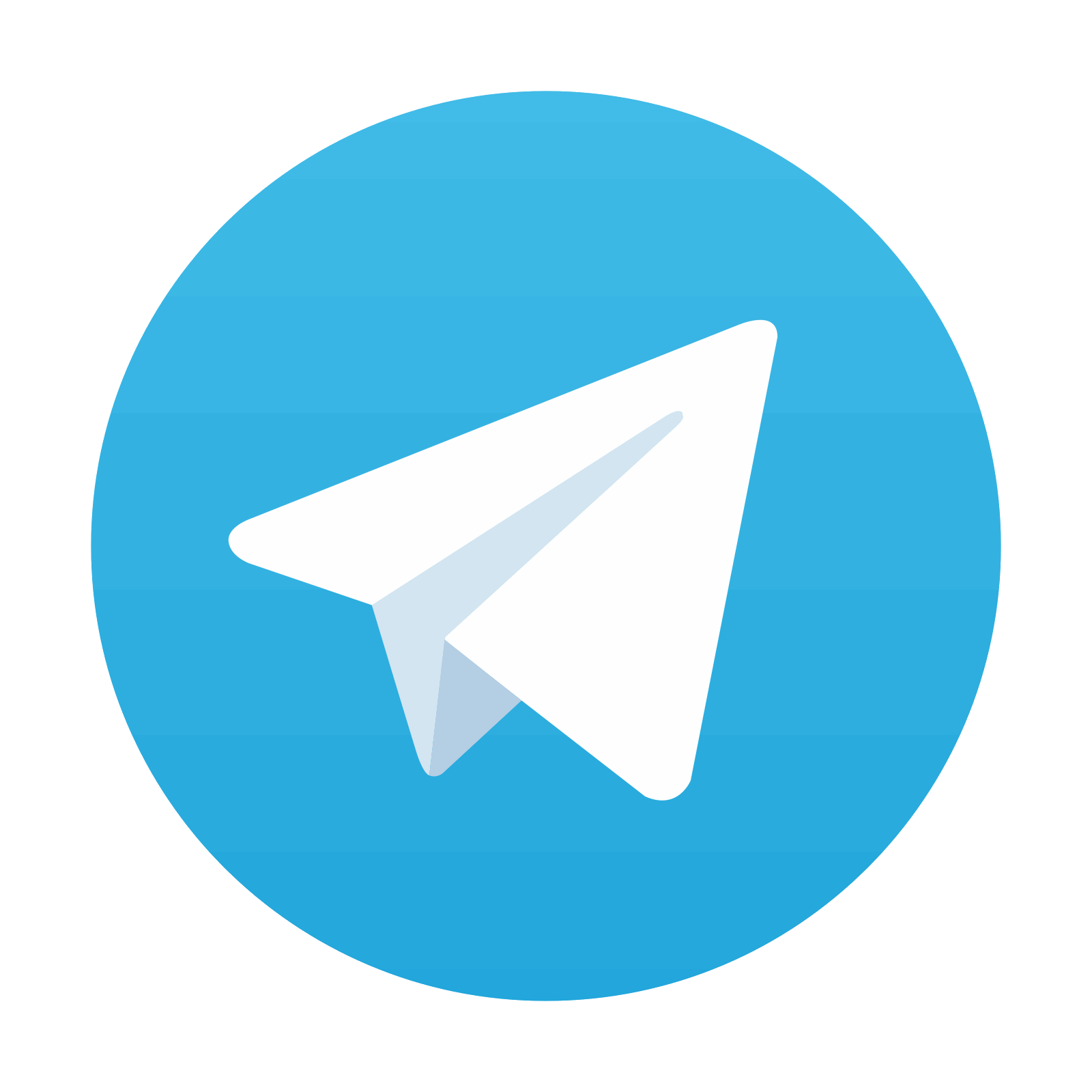
Stay updated, free articles. Join our Telegram channel

Full access? Get Clinical Tree
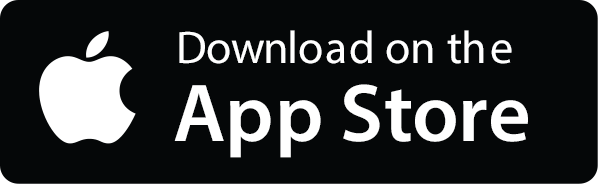
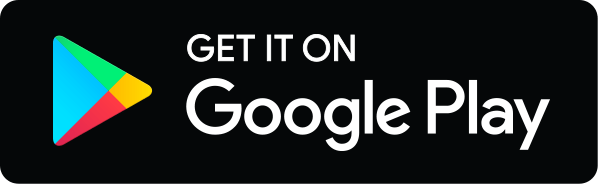