Principles of Pharmacotherapy in Pediatrics
Anita Siu
James C. Thigpen Jr
When treating pediatric patients, many health care practitioners use the terms infant, child, or even kid interchangeably. However, there are currently accepted terms that define the different age categories of pediatric patients (Table 4.1). These terms should be used for accuracy when describing young patients and especially when determining drug dosages. Safe and effective drug therapy in pediatric patients is based on a firm understanding of three concepts:
Ongoing maturation and development in pediatric patients and their effect on a drug’s absorption, distribution, metabolism, and excretion. Interpatient variabilities may be attributed to physiologic changes throughout childhood.
Short- and long-term effects that the prescribed drug will have on a pediatric patient’s growth and development
Effects of underlying congenital, chronic, or current diseases on the prescribed drug, and vice versa
The popular concept that the pediatric patient is merely a “little or small adult,” and therefore, pediatric pharmacokinetics, drug dosing, and even adverse effects can be extrapolated from the results of adult clinical drug trials is a serious misconception. Although many drugs do exhibit similarities between the adult and pediatric populations, the assumption of resemblance should not be applied to all drugs. Several tragic drug misadventures in the 1960s and 1970s illustrate this. Extrapolated data from adult responses to chloramphenicol (Chloromycetin) led to its use in neonates in the 1960s. When given chloramphenicol, these neonates developed gray baby syndrome, hypotension, and hypoxemia, leading eventually to shock and death (Haile, 1977). This occurred because neonates, unlike adults, lack the enzyme needed to metabolize chloramphenicol. Another tragedy in the 1970s involved the topical antimicrobial cleanser hexachlorophene. Used routinely and safely in adults, hexachlorophene caused vacuolar encephalopathy of the brain stem in premature neonates after they were repeatedly bathed in a 3% solution (Anonymous, 1972).
Several barriers exist for pharmaceutical manufacturers in conducting pediatric clinical trials, such as fears of unforeseen adverse events affecting growth or development or difficulties in obtaining informed consent or blood samples. In turn, the lack of clinical trials in pediatric patients prevents the U.S. Food and Drug Administration (FDA) from approving drugs for use in the pediatric population. As such, the prescribing information commonly states “Pediatric Use: Safety and effectiveness in pediatric patients has not been established.”
Without FDA approval or adequate documented information, many practitioners are uncertain how to use drugs in pediatrics. This leaves prescribers little choice but to use drugs in pediatric patients in an off-label capacity, based on adult data, uncontrolled pediatric studies, or personal experience. In 1997, the FDA took the initiative to increase the quantity and quality of clinical drug trials in the pediatric population by proposing alternate ways to obtain FDA approval. The FDA would waive the need for well-controlled clinical drug trials if drug manufacturers provided other satisfactory data for drugs already approved for the same use in adults. These data could include the results of controlled or uncontrolled pediatric studies, pharmacodynamic studies, safety reports, and premarketing or postmarketing studies. Alternatively, the drug manufacturer could provide evidence demonstrating that the disease course and drug effects are sufficiently similar in adult and pediatric patients in order to support extrapolation of data from adult clinical trials. In addition, pediatric pharmacokinetic studies are necessary to provide
data for an appropriate pediatric dosage recommendation, especially age-dependent dosing. An FDA regulation issued in December 1998 required manufacturers to provide additional information about the use of their drug products in pediatric patients. The nature of the studies required to support pediatric labeling will depend on the type of application, the condition being treated, and existing data about the product’s safety and efficacy in pediatric patients. Manufacturers will be required to study the drug in all relevant pediatric age groups (U.S. FDA, 1998a). Over the years, the FDA has encouraged more well-controlled trials on drug efficacy and safety in pediatrics. The FDA Modernization Act of 1997 and the Best Pharmaceuticals for Children Act of 2002 offered support for the pharmaceutical industries to conduct and submit pediatric clinical trials. Companies that conduct appropriate clinical trials are eligible to receive a 6-month patent extension on their product. The Pediatric Research Equity Act of 2003 mandated that drugs used in pediatrics require literature or clinical trials supporting their use, even if the original patent did not have a pediatric indication. As a result, pediatric pharmacotherapy will evolve with additional clinical trials.
data for an appropriate pediatric dosage recommendation, especially age-dependent dosing. An FDA regulation issued in December 1998 required manufacturers to provide additional information about the use of their drug products in pediatric patients. The nature of the studies required to support pediatric labeling will depend on the type of application, the condition being treated, and existing data about the product’s safety and efficacy in pediatric patients. Manufacturers will be required to study the drug in all relevant pediatric age groups (U.S. FDA, 1998a). Over the years, the FDA has encouraged more well-controlled trials on drug efficacy and safety in pediatrics. The FDA Modernization Act of 1997 and the Best Pharmaceuticals for Children Act of 2002 offered support for the pharmaceutical industries to conduct and submit pediatric clinical trials. Companies that conduct appropriate clinical trials are eligible to receive a 6-month patent extension on their product. The Pediatric Research Equity Act of 2003 mandated that drugs used in pediatrics require literature or clinical trials supporting their use, even if the original patent did not have a pediatric indication. As a result, pediatric pharmacotherapy will evolve with additional clinical trials.
TABLE 4.1 Age Groups of Pediatric Population | ||||||||||||
---|---|---|---|---|---|---|---|---|---|---|---|---|
|
TABLE 4.2 Age-Related Pharmacokinetic Differences in Children Compared with Adults | ||||||||||||||||||||||||||||||||||||||||||||||||||||||||||||||||||||||||||||||||||
---|---|---|---|---|---|---|---|---|---|---|---|---|---|---|---|---|---|---|---|---|---|---|---|---|---|---|---|---|---|---|---|---|---|---|---|---|---|---|---|---|---|---|---|---|---|---|---|---|---|---|---|---|---|---|---|---|---|---|---|---|---|---|---|---|---|---|---|---|---|---|---|---|---|---|---|---|---|---|---|---|---|---|
|
PEDIATRIC PHARMACOKINETICS
Pediatric patients differ from adults, anatomically and physiologically. For safe use of drugs in pediatrics, prescribers and other caregivers need to recognize the potential for very different pharmacokinetics in pediatric patients as opposed to adults. The differences are based on developing body tissues and organs, which affect a drug’s absorption, distribution, metabolism, and excretion.
Changes in a pediatric patient’s body proportions and composition and the relative size of the liver and kidneys can alter the pharmacokinetics of a drug. During the first several years of life, a child undergoes rapid changes in growth and development, most rapid during infancy. Growth is a quantitative change in the size of the body or any of its parts, and development is a qualitative change in skills or functions. Maturation, a genetically controlled development independent of the environment, is a slower process, lasting until late childhood. Table 4.2 summarizes pharmacokinetic differences in pediatric patients.
By the end of the first year of life, an infant’s weight triples, whereas body surface area (BSA) and length double. Accompanying these changes in growth and development are changes in body composition, intracellular and extracellular body water, fat, and protein. Approximately 75% to 80% of a full-term neonate’s body weight is total body water (Friis-Hansen, 1957). By age 3 months, total body water constitutes
approximately 65% of the patient’s body weight. Extracellular water progressively declines and intracellular water increases faster than total body water, exceeding extracellular water (Friis-Hansen, 1957). The decreasing percentage of body weight from total body water is replaced by an increase in body fat during the first 5 months of life. In fact, the percentage of body weight from fat doubles in these 5 months. The protein percentage increases during the second year of life as fat is lost, primarily because of ambulation. The liver and kidney reach their maximum size relative to body weight by age 2, producing a “peak” in the child’s metabolism and elimination. After age 2, the child’s liver and kidney size/body weight ratios steadily decrease until adult liver and kidney ratios are reached by adolescence.
approximately 65% of the patient’s body weight. Extracellular water progressively declines and intracellular water increases faster than total body water, exceeding extracellular water (Friis-Hansen, 1957). The decreasing percentage of body weight from total body water is replaced by an increase in body fat during the first 5 months of life. In fact, the percentage of body weight from fat doubles in these 5 months. The protein percentage increases during the second year of life as fat is lost, primarily because of ambulation. The liver and kidney reach their maximum size relative to body weight by age 2, producing a “peak” in the child’s metabolism and elimination. After age 2, the child’s liver and kidney size/body weight ratios steadily decrease until adult liver and kidney ratios are reached by adolescence.
Oral Absorption
The extent of a drug’s absorption in a pediatric patient depends on a variety of factors: gastric pH, gastric and intestinal transit time, gastrointestinal surface area, enzymes, and microorganism flora or any combination thereof.
Gastric pH
Basal and stimulated secretion of gastric acid controls the pH of the stomach. The stomach pH is alkaline at birth (greater than 4) because of residual amniotic fluid and the immaturity of parietal cells. As gastric acid is produced, the pH falls. By the end of the first day of life, the basal and stimulated rates are equal, although lower than the rates in adults. An increased stomach pH (alkaline) adversely affects the absorption of weakly acidic drugs and improves the absorption of weakly basic drugs. This phenomenon results from increased ionization of the weakly acidic drug, producing more ionized (polar) drug, which moves poorly across the nonpolar gastric membrane, and vice versa for weakly basic drug. For example, the bioavailability of phenobarbital (a weak acid) is decreased in neonates, infants, and young children because their alkaline gastric pH produces more ionized phenobarbital, which crosses the gastric membrane poorly.
For weakly basic drugs, the alkaline stomach pH increases the nonionized form of the drug, which then easily moves across the gastric membrane. By the second year of life, the child’s gastric acid output on a per kilogram body weight basis is similar to that observed in the adult (Deren, 1971). As a result, gastric pH affects the degree of drug ionization, thus changing the amount of drug absorbed.
Gastric Emptying Time and Surface Area
The gastric emptying time is delayed in both preterm and full-term neonates during the first 24 hours of life. No studies have been conducted beyond the immediate neonatal period. The combination of delayed gastric emptying time and gastroesophageal reflux can result in the regurgitation of orally administered drugs, producing irregular drug absorption. In general, gastric emptying is more prolonged in neonates and infants than in children.
The characteristics of a drug’s movement through the intestines can drastically affect the rate and extent of drug absorption because most drugs are absorbed in the duodenum. Both neonates and infants have irregular peristalsis, which can lead to enhanced absorption. In addition, the type of feeding an infant receives can affect intestinal transit time. For instance, the intestinal transit time in breast-fed infants is greater than in formula-fed infants (Cavell, 1981).
The relative size of the absorptive surface area in the duodenum can significantly influence the rate and extent of drug absorption. In the young, the greater relative size of the duodenum compared with adults enhances drug absorption.
Gastrointestinal Enzymes and Microorganisms
The absorption of drugs that are fat soluble or carried in fat vehicles depends on lipase. Premature neonates have low lipase concentrations and no alpha amylase. The reduced activity of bile acids, lipase, alpha amylase, and protease continues until approximately age 4 months. Vitamin E absorption is decreased in neonates because of the diminished bile acid pool and biliary function; therefore, supplementation of this vitamin may be necessary.
The development of the intestinal microorganism flora depends more on diet than on age (Yaffe & Juchau, 1974), which may account for the more rapid development of flora in breast-fed infants than in formula-fed infants. The reduction of digoxin (Lanoxin) to inactive metabolites by anaerobic intestinal bacteria can be used as a marker for the development or changes in intestinal flora (Lindenbaum et al., 1981). Digoxin metabolites are not detected in children until 16 months, and an adult-like reduction of digoxin does not occur until age 9 (Linday et al., 1987).
Rectal Absorption
The rectal route of administration is seldom used; it usually is reserved for patients who cannot tolerate oral drugs or who lack intravenous access. In rectal administration, the drug is absorbed by the hemorrhoidal veins, which are not part of the portal circulation, therefore avoiding first-pass hepatic elimination. Unfortunately, most drugs administered by this route are erratically and incompletely absorbed. Feces in the rectum, frequent bowel movements in neonates and infants, and lack of anal sphincter muscle contribute to the poor absorption profile of drugs administered rectally.
Although rectal administration may not be appropriate for routine dosing of drugs, the rectal administration of diazepam (Valium), valproic acid (Depakote), or midazolam (Versed) has been used to control seizures when intravenous access could not be quickly established in infants or children with status epilepticus (Brigo et al., 2015; Graves & Kriel, 1987).
Intramuscular and Subcutaneous Absorption
Both the characteristics of the patient and the properties of the drug influence the absorption of intramuscularly or subcutaneously administered drugs. Patient characteristics include blood
flow to the muscle, muscle mass, tone, and activity. Important properties of the drug are its solubility, the pH of extracellular fluid, its ease in crossing capillary membranes, and the amount of drug administered at the injection site.
flow to the muscle, muscle mass, tone, and activity. Important properties of the drug are its solubility, the pH of extracellular fluid, its ease in crossing capillary membranes, and the amount of drug administered at the injection site.
In pediatric patients, all the patient characteristics are highly variable. Neonates have decreased muscle mass, and their limited muscle activity decreases blood flow to and from the muscle. Collectively, these factors produce erratic and poor intramuscular drug absorption. On the contrary, infants possess a greater density of skeletal muscle capillaries than older children, allowing for more efficient drug absorption. Some drugs, such as erythromycin, can cause pain at the injection site and should not be administered intramuscularly. However, many drugs, such as the penicillins, reach concentrations in the serum with intramuscular administration that are comparable with those achieved after intravenous administration, with minimal adverse effects.
Percutaneous Absorption
Adverse effects resulting from the inadvertent systemic absorption of percutaneously administered hexachlorophene emulsion, salicylic acid ointment, and hydrocortisone creams in neonates have limited the use of this route of drug administration. The absorption of compounds is inversely related to the thickness of the stratum corneum and directly related to hydration of the skin (Morselli et al., 1980). Relative to body mass, the BSA is greatest in the infant and young child compared with older children and adults. The decreased thickness of the skin with increased skin surface hydration relative to body weight produces much greater percutaneous drug absorption in neonates than in adults. The percutaneous administration of drugs in neonates does pose some risks of toxic effects. Neonatal skin is structurally immature, resulting in less subcutaneous fat and a thinner stratum corneum and epidermis (Rutter, 1987). Since a greater skin surface area/body weight ratio is observed during the neonatal period, percutaneous drug absorption is also superior. Both the advantages and subsequent disadvantages of enhanced percutaneous absorption disappear after infancy, however.
Mucosal Absorption
Mucosal administration of medications, whether via the nasal or buccal route, has become a viable method for use in children. Some medications, such as nasal corticosteroids, are intended for a local effect and have almost no systemic absorption or effects. However, some medications, such as midazolam (Versed) and ketamine (Ketalar), have been administered by nasal aerosolization with good absorption and systemic effect (Hosseini Jahromi et al., 2012; Klein et al., 2011). Administration by these routes avoids the trauma of placing an intravenous line and the associated costs. Also, in urgent situations where there may be considerable difficulty placing an intravenous line (i.e., status epilepticus), nasal administration can be utilized with great effectiveness (Thakker & Shanbag, 2013).
Pulmonary Absorption
Aerosolized drug delivery to the lungs continues to be a favorite technique in many respiratory disorders, such as asthma. Factors affecting drug deposition in the lungs include particle size, lipid solubility, protein binding, drug metabolism in the lungs, and mucociliary transport (American Academy of Pediatrics, 1997). Aerosol particle size and lipid solubility are factors in determining whether the drug is deposited in the upper or lower airways; smaller particle size and lipid-soluble drugs are more likely to be absorbed and deposited in the lower airways (Bond, 1993).
Besides drug considerations, pediatric characteristics also affect aerosol drug delivery. Infants and children have lower tidal volumes and increased respiratory rates (especially while crying), reducing drug delivery and absorption in the lungs. Studies have shown that less than 2% of aerosolized drugs are deposited in young infants and toddlers (Fok et al., 1996; Salmon et al., 1990). Therefore, adult dosing may be necessary to counteract these effects.
Distribution
Six factors affect drug distribution in the pediatric population: vascular perfusion, body composition, tissue-binding characteristics, physicochemical properties of the drug, plasma protein binding, and route of administration (Stewart & Hampton, 1987). During the neonatal period, most of these factors are significantly different from those in the adult population, while children and adolescents are very similar to or the same as adults.
Vascular Perfusion
Changes in vascular perfusion are common in neonates. For example, in neonatal respiratory distress syndrome and postasphyxia, a right-to-left vascular shunt may occur and divert blood from the lungs to the tissues and organs, potentially changing the Vd of some drugs.
Body Composition
Neonates have increased total body water (75% to 80%) with decreased fat compared with adults, resulting in a higher water-to-lipid ratio. After the neonatal period, fat increases and total body water decreases steadily until puberty, especially in girls. For instance, neonates and infants have increased total body and extracellular water, creating a larger volume of distribution and affecting the pharmacokinetics of some drugs, such as aminoglycoside. The larger volume, in turn, requires administering a larger milligram-per-kilogram dose of aminoglycosides to neonates and infants than to adults.
Tissue-Binding Characteristics
The mass of tissue available for binding can affect drug distribution. Drugs extensively bound to tissues exhibit increased “free” blood levels when the mass of tissue is reduced by disease or degeneration or immaturity, as in pediatrics.
Physicochemical Properties
The physicochemical properties of a drug include lipid solubility (ionized vs. nonionized) and molecular configuration. These properties affect the ability of a drug to move across membranes into target cells or tissues. Drugs that display favorable properties for absorption may pose a greater risk for toxicity in neonates, who have enhanced percutaneous drug absorption.
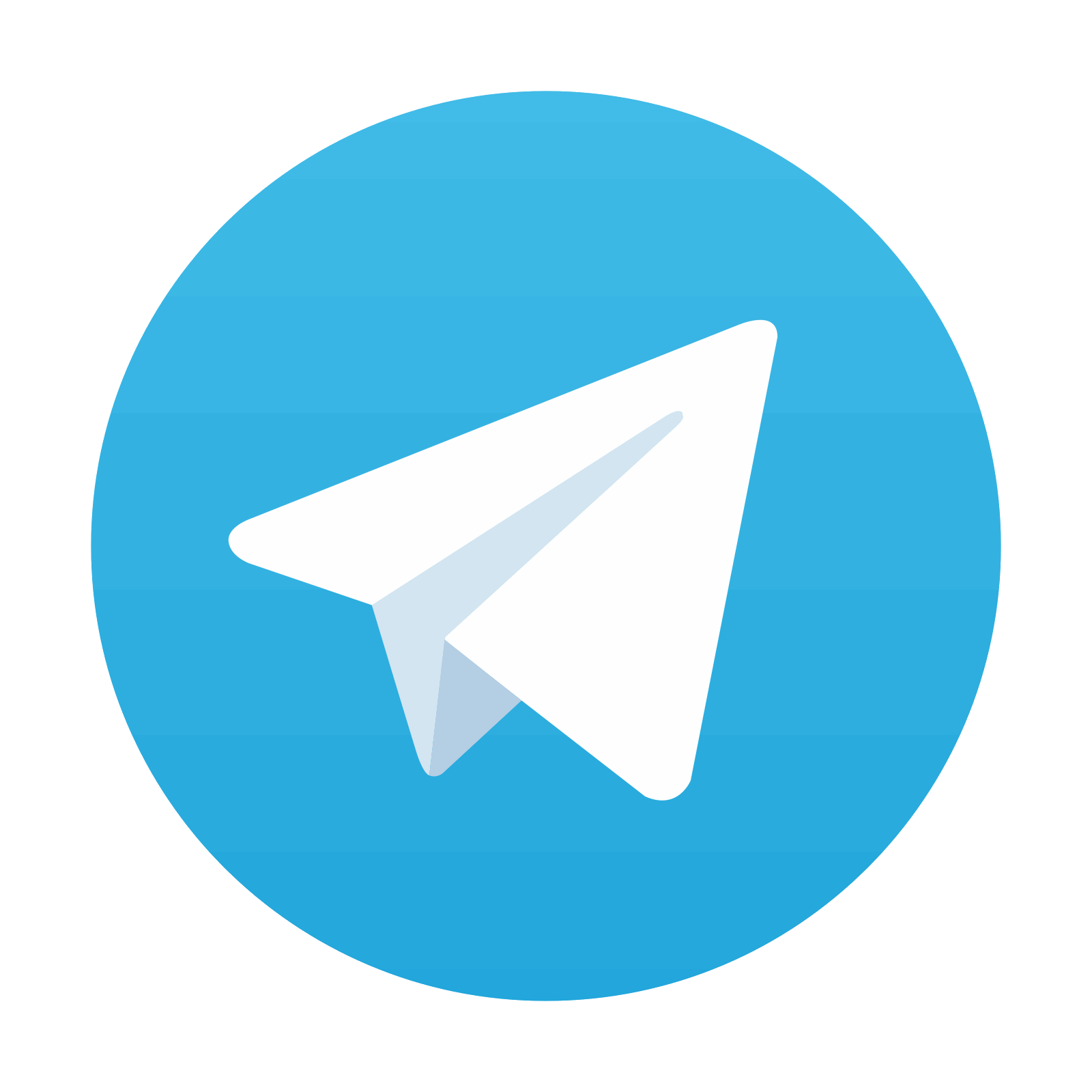
Stay updated, free articles. Join our Telegram channel

Full access? Get Clinical Tree
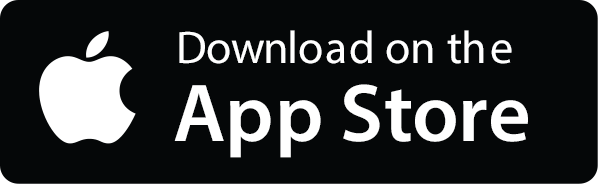
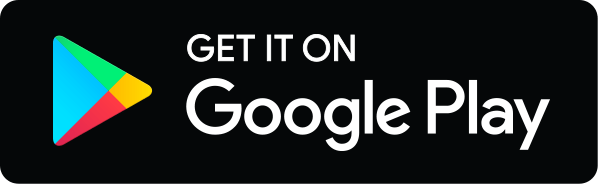
