2 Principles of herbal pharmacology
Chapter contents
Defining our ground
Pharmacology can be defined as the study of the interaction of biologically active agents with living systems.1 The study of pharmacology is further divided into two main areas. Pharmacodynamics looks at the effects of an agent at active sites in the body. In contrast, pharmacokinetics is concerned with the effects the body has on the medicine, and specifically the concentrations that can be achieved at active sites. The approach used in this chapter, and to some extent in the therapeutic chapters, is to examine the pharmacology of key chemical groups in plants, the ‘archetypal plant constituents’, as much as individual herbs. (For detailed information on the pharmacology of selected herbs see Part Three.)
The chemical nature and classification of these archetypal plant constituents are studied within the discipline known as phytochemistry. Hence, any discourse on herbal pharmacology must be founded on a sound knowledge of phytochemistry. A misconception about ‘why medicinal plants work’ sometimes occurs in lay and even in professional circles. This is that the various nutrients such as vitamins, minerals and so on are largely responsible for the pharmacological activity of plants. (It is perhaps supported by the fact that most herbal products in the United States are regulated as ‘dietary supplements’.) Almost without exception, this is not the case. One notable exception is that certain plants, such as nettle leaf (Urtica species) and horsetail (Equisetum species), are rich sources of the trace element silicon. There is evidence that part of this silicon in horsetail is found intimately associated with cell wall polymers and can be released under mild extraction.2 Another interesting exception comes from the clinical use of a leaf concentrate from alfalfa (Medicago sativa) as an efficacious iron and folate supplement.3
• Bruneton J. Pharmacognosy, Phytochemistry, Medicinal Plants, 2nd ed. Paris: Lavoisier Publishing; 2008.
• Evans WC. Trease and Evans’ Pharmacognosy, 16th ed. London: Saunders Elsevier; 2009.
The field of pharmacognosy (from the Greek pharmacon for medicine and gnosis for knowledge) is the study of the definition, description and phytochemistry of natural drugs (typically medicinal plants or preparations derived from them).4 In fact, the term drug is derived from the old French word drogue, meaning to dry, referring originally to dried herbs. These days the application of pharmacognosy often extends to knowledge about the pharmacology of medicinal plants, and in some circles the term is used to specifically denote the study of herbal pharmacology, although this is technically incorrect.
There are also some primers in phytochemistry aimed at the herbal reader who does not have a strong background in natural products chemistry. In addition to this chapter, they represent a useful starting point for students of phytotherapy.5,6
Why should secondary metabolites have biological activity in animals? One suggestion put forward by Michael Baker is that enzymes in animals can share a common ancestry with enzymes or proteins in plants.7 This evolutionary kinship, when combined with structural similarities between plant and animal substrates for these enzymes, could explain the hormone-like or hormone-modulating effects of several archetypal plant constituents in humans. (See the licorice monograph for one such example.)
The role of secondary metabolites
However, there is still considerable debate on this complex issue. Even the line between primary and secondary metabolites can be arbitrary. They cannot be readily distinguished on the basis of precursor molecules, chemical structures or biosynthetic origins. For example, both primary and secondary metabolites are found among the diterpenes and triterpenes. In the diterpene series, both kaurenoic acid and abietic acid are formed by a similar sequence of related enzymatic reactions; the former is an essential intermediate in the synthesis of gibberellins. These are growth hormones found in all plants.8 The latter is a resin component. Similarly, the essential amino acid proline is classified as a primary metabolite, whereas the six-carbon ring analogue pipecolic acid is considered an alkaloid and hence a natural product. Even lignin, the essential structural polymer of wood and second only to cellulose as the most abundant organic substance in plants, is considered a natural product (secondary metabolite) rather than a primary metabolite.8
It has been suggested that, in the absence of a valid distinction based on chemical structure and biochemistry, a functional definition becomes the logical choice.8 Primary metabolites participate in nutrition and essential metabolic processes inside the plant, whereas secondary metabolites influence ecological interactions between the plant and its environment.
But even here, there is disagreement. Firn and Jones have recently argued that the terms primary and secondary metabolism as applied to plants are misleading and unsatisfactory.9 They suggested that important metabolites such as lipids, polysaccharides and carotenoids do not fit into either class, and proposed that they be classified as supportive metabolites (with the remainder of secondary metabolites classified as speculative metabolites).
By way of elaboration, lipids are essential for the short-term functioning of the cell and all cells contain a mix of lipids. But there are some individual lipids made by only a few species and, when their synthesis is inhibited, the cell suffers no short-term disadvantage.9
Since there is no consensus yet on this issue, the term ‘secondary metabolites’ will still be employed in this chapter. Be they speculative, supportive or secondary metabolites, their specialised functions include:10
• defence against herbivores (insects, vertebrates)
• defence against plant pathogens
• defence against other plants
• signal compounds to attract pollinating and seed dispersing animals
• signals for communication between plants and symbiotic microorganisms
• protection against ultraviolet light, oxidation and other physical stressors.
Based on their biosynthetic origins, plant secondary metabolites (the archetypal plant constituents) can be divided into three major groups: the terpenoids, the alkaloids, and the phenylpropanoid and allied phenolic compounds.8 All terpenoids, including the primary metabolites, are derived from the five-carbon precursor isopentenyl diphosphate. Alkaloids are biosynthesised principally from amino acids, and the phenolic compounds by either the shikimic acid pathway or the malonate/acetate pathway.8
According to Efferth and Koch, two large groups of secondary plant metabolites can be distinguished in terms of their biological/therapeutic activities:11
• A smaller group of highly active compounds possessing a high selectivity for cellular targets
• A larger group of moderately or weakly acting compounds that interact with a broad range of cellular targets (hence possessing molecular promiscuity).
These authors reflect that medicinal plants with highly active phytochemicals represent only a minority of those commonly used (probably because of potential toxicity). However, plants with highly active phytochemicals are sought after in natural products research because they represent prime candidates for new drug discovery. In contrast, 90% of all thoroughly described medicinal plants contain broad-spectrum phytochemicals with weak or moderate bioactivity. Efferth and Koch suggest that plants have learned to cope with the problem of resistance development by producing combinations of pleiotropic multi-targeted phytochemical complexes (see also the concept of intelligent mixtures discussed later).11 They also note that those medicinal plants synthesising certain classes of highly active compounds might protect themselves by producing ‘prodrug’ phytochemicals that are activated only in predators or on damage to the plant.11 Many such molecules are glycosides (for example, see the later discussion of cyanogenic glycosides and glucosinolates).12,13
Alkaloids can play a defensive role in plants against herbivores and pathogens.8,14 Glucosinolates appear to have a role in protecting against insect attack.15 Tannins act to preserve the wood in living trees from microbial decomposition and insects.12 Several classes of secondary metabolites are induced by infection, wounding or grazing, which probably speaks to their defensive roles.16 Variation in the speed and extent of such induction may account, at least in part, for the differences between resistant and susceptible plant varieties.17 Both salicylic and jasmonic acids have been implicated as signals in such responses. Toxic chemicals formed in response to damage, especially from fungal attack, are called phytoalexins.12 In legumes, secondary metabolites are involved in interactions with beneficial micro-organisms (flavonoids as inducers of the Rhizobium symbiosis) and in defence against pathogens (isoflavonoid phytoalexins).8,18
As noted above, plants have also developed chemical defences against other plants, a phenomenon known as negative allelopathy. Many compounds are implicated, including phenolics19 and terpenoids.20,21 Positive biochemical interaction, or facilitation, among plants is also becoming increasingly recognised (positive allelopathy).22
Confusing the use of the term is the fact that ‘allelopathy’ can be applied in a broader context to denote the interactions between plants and animals. In other words, the word is sometimes used to describe the field of study of the broader ecological functions of secondary metabolites, as discussed above. A recent allelopathy textbook reflects this wider application of the term.21
How do herbs differ from conventional drugs?
Synergy and additive effects
‘The body is not a one-note melody, but a symphony of many interactive components functioning synergistically … the active ingredient model does not stem from a strength of the scientific method, as often supposed; rather, it stems from a weakness – from the inability of the reductionist method to deal with complex systems.’23
Synergy is an important hypothesis in herbal pharmacology in the context of the advantage of chemical complexity. It applies if the action of a chemical mixture is greater than the arithmetical sum of the actions of the mixture’s components: the whole is greater than the sum of the individual parts. In other words, there is a cooperative or facilitating effect between the components for a specific outcome. Another helpful definition of synergy is an effect seen by a combination of substances that is greater than would have been expected from a consideration of individual contributions.24 A well-known example of synergy is exploited in the use of insecticidal pyrethrins. A synergist known as piperonyl butoxide, which has little insecticidal activity of its own, interferes with the insect’s ability to break down the pyrethrins, thereby substantially increasing their toxicity. This example emphasises one important possible mechanism behind synergy as applied to medicinal plant components: increased or prolonged levels of key components at the active site. In other words, components of plants that are not active themselves can act to improve the stability, solubility, bioavailability or half-life of the active components. Hence a particular chemical might in pure form have only a fraction of the pharmacological activity that it has in its plant matrix. This key mechanism for synergy, therefore, has a pharmacokinetic basis.
Methods for assessing pharmacological synergy were proposed in an extensive paper by Berenbaum in 1989.25 Later Williamson26 and Houghton27 elaborated on these in the context of medicinal plants. Essentially, there are four mathematical techniques for demonstrating synergy proposed and discussed in these works, but only the isobole method is truly rigorous, although it is not always undertaken because of its complexity. Hence, several of the examples of herbal synergy discussed below need to be viewed in the context that they are only possible examples of synergy, since the isobole method was not performed.
The isobole method has the advantage that it is independent of the mechanism of action involved. Although some explanations of the isobole method can be complex, a simple way to describe it follows. For a series of two-component mixtures, the concentration or dose of component A in each mixture required to give a defined effect (such as an ED50 or minimum inhibitory concentration, MIC) is plotted on the X axis against the concentration or dose of component B in the same mixture on the Y axis. The line joining all the points is then constructed. If there is no interaction between the effects of A and B, and their combination is merely additive, the line will be straight (Fig. 2.1). If there is a synergy between A and B, the line (the isobologram) will be concave. Alternatively, if antagonism exists the isobologram will be convex.
There are some published examples in the literature of the isobole method demonstrating synergy for mixtures of phytochemicals from the same herb. Williamson provides the example of ginkgolides A and B from ginkgo in terms of an anti-PAF (platelet activating factor) effect on platelets in vitro.26 In much earlier work in mice, a potentiating effect of different concentrations sennoside C on the purgative activity of sennoside A was observed.28 In this study an isobologram of ED50 values for laxative activity was not plotted, but one can be readily constructed from the data provided showing a concave line. Interestingly, a mixture of these compounds in the ratio 3:7 (which somewhat reflects the relative levels in senna leaf) gave the lowest ED50 value.
One example of where the combination of two components from the same plant has yielded a significantly greater effect than expected is a study on the upregulation of phase II detoxification enzymes in mice by the glucosinolate breakdown products from broccoli.29 An orally administered mixture of crambene and indole-3-carbinol caused a significantly greater induction of glutathione S-transferase and quinone reductase that could be expected from the activity of oral doses of the individual treatments. Another example is the study where the three major curcuminoids from turmeric (Curcuma longa) were investigated for their in vitro nematocidal activity against second stage larvae of Toxocara canis.30 Each of the three curcuminoids was ineffective on its own, whereas any combination of two, or all three together, was active. The combination of all three demonstrated the highest activity.
Another example identified in early research is the antibacterial activity of major components of lemongrass essential oil. While geranial and neral individually elicited antibacterial action, the third main component, myrcene, did not show any activity in vitro. However, when mixed with either of the other two main components, myrcene enhanced their activity.31 As pointed out in one review11 this example is technically not one of synergy, but rather exemplifies potentiation, where an inactive compound enhances the potency of a bioactive one.
In an interesting study, the impact on serum lipids of four active components of Chinese hawthorn (Crataegus pinnatifida), and their combination, was investigated in mice fed a high fat and cholesterol diet.32 The four compounds (quercetin, hyperoside, rutin and chlorogenic acid), and their combination at percentages reflected in the berry, were each fed orally to the mice at a dose of 2.85 mg/kg. The greatest reduction in total cholesterol and LDL-cholesterol was found for the combination. For LDL-cholesterol, only the combination yielded a significant reduction versus the control group (p<0.01).
Pharmacodynamic synergy has also been demonstrated for combinations of extracts or phytochemicals from different herbs. The in vitro antitumour activity for combinations of extracts of Corydalis and turmeric rhizomes (a traditional formulation) demonstrated a synergistic interplay, as determined using an isobologram.33 Berberine from Coptis chinensis and evodiamine from Evodia rutaecarpa demonstrated synergistic antitumour activity in vitro against a human hepatocellular carcinoma cell line.34 A pronounced convex curve was produced on an isobologram.
A recent review highlighted that two key developments are behind growing interest in synergy research in phytotherapy.35 The first is the new methods of analytical chemistry and molecular biology that have become available in the 21st century. The second is an unexpected change of paradigm in chemotherapy that has appeared without drawing much attention as to its radical nature. This is the transition in chemotherapy towards a multi-drug approach. Multi-drug therapy is already widely practised in the treatment of AIDS and other infectious diseases, hypertension, cancer and many other diseases.
In particular, the review notes this multi-drug concept in cancer chemotherapy is often aimed at being biomodulatory, not just at direct destruction of the tumour.35 Instead, suppression of different processes essential for the tumour’s survival are also targeted (such as angiogenesis, oncogene expression, anti-apoptotic mechanisms, immunological tolerance and inflammation). This concept of multi-targeted or polyvalent therapy is not new to phytotherapy and is discussed further below.
There are several examples in the herbal research literature where synergistic interactions are strongly implied, although they have not been absolutely demonstrated using the isobologram method. One example is the research cited by Williamson26 and several others where cannabis extract demonstrated higher antispastic activity in mice than the equivalent dose of pure tetrahydrocannabinol (THC). As pointed out by Houghton,27 this might not represent an example of true synergy. Rather other components in the cannabis extract might also have antispastic activity. In other words, there could be an additive effect between THC and other phytochemical components in the extract. However, the increased activity is so striking that synergy is a very plausible explanation. Testing a THC-free cannabis extract in the same model would have provided clear proof, one way or another.
Another example of a striking result that strongly implies a synergistic interaction is provided by Williamson.26 This is the research where the antiulcer activity of a fraction of ginger extract was found to be 66 times that calculated from the individual components of that fraction. More recent similar examples include:
• analysis of the in vitro butyrylcholinesterase inhibitory activities of essential oils of various Salvia species, which revealed that the activities of isolated major components could not account for the observed activity36
• tea tree oil (Melaleuca alternifolia), which exhibited pronounced in vitro antitumour activity against murine B16 melanoma cells.37 However, a ‘mock’ tea tree oil (containing its five major phytochemical components at the same concentrations found in the oil, and representing 80% of its total content) was only half as active. Of these five major oil components, only terpinen-4-ol (about 40% of the oil) was active in this model.
There are several examples of a pharmacokinetic basis for synergy, where chemical complexity results in enhanced solubility or bioavailability of key phytochemical components in plant extracts. The basic issues were discussed by Eder and Mehnert.38 The isoflavone glycoside daidzin given in the crude extract of Pueraria lobata achieves a much greater concentration in plasma than an equivalent dose of pure daidzin.39 Ascorbic acid in a citrus extract was more bioavailable than ascorbic acid alone.40 More recently, consumption of a preparation of fresh kiwi fruit resulted in up to five times more effective ascorbate delivery to tissues than when ascorbate was administered via the drinking water to vitamin C-deficient mice.41 The kava lactones appear to increase each other’s bioavailability, especially when given as the herbal extract. (For more details, see the kava monograph under Pharmacokinetics.)
Improved pharmacokinetic parameters can also apply to combinations of herbs, although this is not necessarily always the case. The traditional Chinese formulation Huangqin-Tang decoction, together with decoctions of its individual herbal components, were studied in rats after oral dosing.42 The constituents/metabolites baicalin, wogonoside, oroxylin-A-glucuronide, risidulin I and liquiritin demonstrated higher overall bioavailability from the compound formulation than from the relevant single herb decoctions, due largely to a longer residence time.
Often synergy is invoked to explain experimental results that can be readily explained by additive effects. Synergy is not always necessary to justify the use of complex mixtures, be they single herbal extracts or herb combinations. Additive effects can be just as favourable to enhanced therapeutic activity and are probably more common in phytotherapy. A team of German scientists headed by the late Dr Hilke Winterhoff discovered that the procyanidins in St John’s wort extract (Hypericum perforatum) significantly increased the in vivo antidepressant activity of hypericin and pseudohypericin, probably by a solubilising effect (these compounds otherwise have poor solubility).43 The same research team found that the flavonoids in St John’s wort have activity in an animal model of depression, with hyperoside and isoquercitrin showing significant activity.44 The flavonoid perspective was backed up by another in vivo study from Italy that found a flavonoid-enriched St John’s wort extract exhibited a more significant influence on central neurotransmitters.45 Later work from the German researchers demonstrated that a St John’s wort extract free of both hypericin and hyperforin (another phytochemical in the herb thought to play a role in the antidepressant activity) still exerted an antidepressant activity in rodent models.46 Hence, it seems that hypericin, procyanidins (indirectly), hyperforin and the flavonoids can all contribute to the clinically verified antidepressant activity of St John’s wort.
Additive effects also apply to herb combinations. The reduction of amphetamine-induced hypermotility was studied in mice after a single oral dose of passionflower extract (Passiflora incarnata, 250 mg/kg), kava extract (100 mg/kg) or the two combined (250 mg/kg+100 mg/kg, respectively).47 The combination yielded a much greater reduction in hypermotility over 2 hours than each individual herbal extract, which the authors attributed to a synergistic interaction. However, a careful analysis of the numbers suggests that the observed result is more likely to have been due to an additive effect (in terms of the differences observed from the control group). Additive benefits have also been observed at a clinical level. A combination of ginseng (Panax ginseng) and Ginkgo has been extensively investigated in volunteers for its acute and long-term impact on cognitive function. The effect of the combination appears to be significantly greater than either herb alone. This might be an example of synergy (which would be difficult to prove conclusively) but is at least an additive effect. (See the ginseng monograph for more details.)
Polyvalent or multifaceted activity
As touched on above, the concept of multi-agent medicines is a developing theme in modern drug therapy. However, it represents nothing new for phytotherapy. Due to their chemical complexity, even a single herbal extract is a nature-designed multi-agent medicine that can simultaneously target a range of desirable pharmacological effects. There are many examples of this provided in the herbal monographs in Part Three. This helps to explain why identifying the ‘active constituent’ in many herbal extracts has proved to be so difficult. For most if not all herbal extracts the ‘active constituent’ is the whole extract itself, as illustrated by the above discussion of the antidepressant activity of St John’s wort. The potential for chemical complexity to confer polyvalent activity or polypharmacology can also explain the apparent therapeutic versatility of herbal extracts. As an example there is the rather large and novel range of clinical uses for Ginkgo, all somewhat supported by evidence from randomised, controlled trials.
It has also been argued that polyvalence may be behind some effects that have been attributed to synergy (although ultimately such a distinction is probably academic, as both explanations argue in favour of chemical complexity).27 In whole organism or tissue studies, other compounds present in the mixture may be active against a range of targets, all acting to contribute to the observed outcome. Houghton goes on further to assert that:
Polyvalence can be defined as the range of biological activities that an extract may exhibit which contribute to the overall effect observed clinically or in vivo. It is often confused with synergism but the distinction lies in the fact that synergism is strictly concerned with only one pharmacological function, rather than a range of activities resulting in an overall effect.27
As outlined by Houghton, polyvalence can occur at three key levels:
1. Several types of phytochemicals are present that each exert a different biological effect.
2. Phytochemicals of one particular chemical type are present that have more than one biological effect relevant to treating the disease and/or improving the health of the patient.
3. Phytochemicals are present that do not affect the cause or symptoms of the disease itself, but instead modify the side effects, absorption, distribution, metabolism or excretion of active constituents.
In a recent review, Gertsch observed that herbal extracts might be ‘intelligent mixtures’ of secondary plant metabolites that have been shaped by evolutionary pressures.48 As such they could represent complex therapeutic mixtures possessing inherent synergy and polyvalence. Gertsch also notes that another important concept related to polyvalence is that of network pharmacology, as originally proposed by Hopkins.49 In the context of plant extracts (which for commonly used herbs typically contain hundreds of potentially bioactive natural products with only mild activity) it is possible that different proteins within the same signalling network are only weakly targeted. However, this is sufficient to shut down or activate a given process by network pharmacology.48 In other words, network pharmacology can explain how a number of weakly active plant secondary metabolites in an extract may be sufficient to exert a potent pharmacological effect without the presence of a highly bioactive compound.48 In the context of herbal pharmacology, Paul Ehrlich’s concept of the magic bullet is supplanted by one of a ‘green shotgun’, to paraphrase Gertsch and James Duke. However, the herbal research that can verify such theoretical constructs is only in its infancy.
The ‘-omic’ technologies (genomics, transcriptomics, proteomics and metabolomics) are recent developments in molecular biology that have the potential to open new perspectives for our understanding of the multi-faceted activities of complex plant mixtures. However, their meaningful application to medicinal plant research poses several challenges.50 The reviews of Wagner and colleagues and Efferth and Koch11 provide a more complete discussion of the application of these techniques to natural product research.
Intelligent mixtures
Whether it is a manifestation of synergy or by other means, the hypothesis that herbal extracts might act at a pharmacological level as intelligent mixtures is intriguing. One such example could be the root of Pelargonium sidoides. Its phytochemical content is unremarkable, including oligomeric procyanidins (OPCs) and highly oxygenated simple coumarins.51 Yet a liquid preparation of the herb has been shown to be effective in several clinical trials. A meta-analysis published in 2008 reviewed its efficacy for acute bronchitis.52 Six randomised trials were included, providing results for a total of 1647 patients (treatment and control groups): one trial compared Pelargonium against a non-antibiotic treatment (N-acetylcysteine); the other five trials tested Pelargonium against placebo. In two trials the ages of the participants were 6 to 12 years, and 6 to 18 years. Adults were enrolled in the other trials. Key findings were:52,53
• all trials reported clinical results suggesting Pelargonium was beneficial in the treatment of acute bronchitis
• meta-analysis of the four placebo-controlled trials involving adults indicated that Pelargonium root significantly reduced bronchitis symptom scores by day 7
• the duration of illness was significantly shorter for patients treated with Pelargonium compared to placebo (Pelargonium-treated patients were able to return to work nearly 2 days earlier than placebo-treated patients)
• patients noticed a treatment effect earlier under Pelargonium than under placebo.
Pharmacodynamics of the archetypal plant constituents
Simple phenols and glycosides
Phenols comprise the largest group of plant secondary metabolites. They range from simple structures with only one benzene ring to larger molecules such as tannins, anthraquinones, flavonoids and coumarins. Tannins and flavonoids are often referred to as polyphenolics. Phenols are defined as compounds that have at least one hydroxyl group attached to a benzene ring. They differ in their chemical properties from other tertiary alcohols because the presence of the benzene ring stabilises the phenolate ion; they are therefore more acidic and more reactive.
From a pharmacological perspective, the best known simple phenol is salicylic acid. Its precursors are found in willow (Salix species) and poplar (Populus species) barks and salicylic acid is subsequently formed on ingestion (see Pharmacokinetics later). Salicylic acid has recognised antipyretic and anti-inflammatory properties that underlie the use of willow bark for fever and arthritis. Acetylsalicylic acid (aspirin) is a synthetic derivative of salicylic acid that in addition has pronounced antiplatelet properties due to the presence of the acetyl group.54 Salicylic acid lacks this property and consequently willow bark is not suitable as a natural substitute for aspirin in cardiovascular patients. Simple phenols are also powerful antiseptics. Arbutin is a phenolic glycoside that confers bacteriostatic properties to urine (see the monograph on bearberry).
Several studies have shown that extracts of many members of the mint family (Lamiaceae or Labiatae) have considerable in vitro antioxidant activity due to the presence of phenolic acids such as rosmarinic acid. Highest antioxidant activity was found in Prunella vulgaris, which had a rosmarinic acid content of about 5%.55
Caffeic acid, as well as its derivatives such as rosmarinic acid and chlorogenic acid, were found to exert antithyroid activity after oxidation. This activity may form the basis of the clinical use of Lycopus species for hyperthyroidism (see the bugleweed monograph).56 Similar oxidation products of caffeic acid inhibit protein biosynthesis in vitro and these compounds probably account for the clinically established antiviral activity of a topical preparation of Melissa officinalis (see also Chapter 8, p. 142).57
The simple phenol resveratrol is probably the most actively researched phytochemical worldwide, with many properties demonstrated in pharmacological models. There are more than 4000 studies on resveratrol. Resveratrol is a phytoalexin produced by several plants in response to fungal attack. However, well-known sources are grapes (Vitis vinifera) and the Chinese herb Polygonum cuspidatum. It has demonstrated an amazing array of (mainly in vitro) pharmacological activities including antioxidant, cardioprotective, antidiabetic, anticancer, antiviral, neuroprotective, antiplatelet, anti-inflammatory and modulation of fat metabolism.58–60 Resveratrol inhibits cancer development at all the three known phases of chemical carcinogenesis, namely initiation, promotion and progression.61 The development of other chronic diseases might also be reduced by resveratrol, based on the many laboratory studies. These diseases include cardiovascular disease, dementia, type 2 diabetes and osteoarthritis.62,63 In addition to its indirect effects on the ageing process via SIRT1 (hence possibly acting as a calorie restriction mimetic), this one simple molecule has the potential to prevent most of the chronic diseases associated with ageing.64 Resveratrol could well be the best example of the molecular promiscuity of common secondary metabolites: ‘Considering the structural simplicity of this stilbene, the intensity of interest is phenomenal’.61
One important pharmacological study was published in Nature in 2006. Rather than administering resveratrol to normal mice to see if it simulated calorie restriction, the effect of resveratrol on a high calorie diet was studied. Middle-aged (1-year-old) male mice on a high calorie diet (HCD) were given resveratrol and compared to untreated mice on the same diet or a standard diet.65 The administered doses of resveratrol were either 5.2 or 22.4 mg/kg/day for 6 months, but only results for the higher dose were reported.
One of the issues with resveratrol is that it is rapidly metabolised and has limited bioavailability as such. However, resveratrol metabolites (mainly phase II conjugates) might also be bioactive, or act as a reservoir of resveratrol at target tissues. One study found that to maximise plasma resveratrol levels it should be taken with a standard breakfast and not with a high fat meal.66 The high fat breakfast was observed to reduce its bioavailability by about 45%.
Cyanogenic glycosides
Cyanogenic glycosides are capable of generating hydrocyanic acid (prussic acid, cyanide). Structurally they are glycosides of 2-hydroxynitriles that can be hydrolysed by the enzyme beta-glucosidase into cyanohydrin. This is unstable and dissociates to hydrocyanic acid. Common cyanogenic glycosides include amygdalin found in bitter almonds and peach kernels (both used in Chinese medicine), and prunasin in wild cherry bark (Prunus serotina). The small quantities of cyanide generated from this bark are said to be responsible for its antitussive properties, although this has not been confirmed in modern pharmacological experiments. Both amygdalin and prunasin yield benzaldehyde on hydrolysis, which accounts for the characteristic almond-like aroma of wild cherry bark. The cyanogenic glycosides linustatin, neolinustatin and linamarin (trace) are found in linseeds (flax, Linum usitatissimum).67
Although hydrocyanic acid is a violent poison, oral intake of cyanogenic glycosides (for example via food, especially in primitive diets) is not necessarily toxic, particularly in the short-term. Hydrolysis of the glycosides in the digestive tract or by the liver leads to a slow release of hydrocyanic acid that is readily detoxified by the body. Addition of 10% apricot kernels to the diet of rats for 18 weeks showed only moderate toxic effects.68 Amygdalin given orally to humans at 500 mg three times a day produced no toxic effects and only moderately raised blood cyanide levels.69 However, co-administration of beta-glucosidase with amygdalin to rats substantially increased its toxicity.70 Acute poisoning has occurred in grazing animals.71
Considerable interest arose in the 1970s regarding the use of a synthetic cyanogenic glycoside, patented as Laetrile (mandelonitrile beta-glucuronide), as an alternative anticancer compound.72 However, most of what was subsequently sold as Laetrile was in fact amygdalin (which probably had similar properties on injection or ingestion).73 The theory proposed was that cancer tissues contain beta-glucosidase and circulating cyanogenic glycosides can therefore act as selective cytotoxic agents. However, amygdalin did not prove to be an effective anticancer agent either in animals74 or in humans,75 presumably because the beta-glucosidase activity of cancer cells is quite low.76 In an interesting development of the Laetrile theory, beta-glucosidase was conjugated to a tumour-associated antibody. When combined with amygdalin in vitro, the cytotoxicity of amygdalin to tumour cells was increased 36-fold.77
Longer periods of intake of subacute amounts of cyanogenic glycosides in daily food have led to chronic intoxication. Products of human detoxification processes, such as the formation of rhodanide and cyanocobalamine, lead to severe diseases, especially neurotoxic syndromes.67 This is thought to be involved in tropical ataxic neuropathy in Nigeria. In other African countries, consumption of cassava root (Manihot esculenta, also known as tapioca) together with a diet deficient in sulphur amino acids is apparently responsible for an endemic upper motor neuron disease known as konzo.67 The peeled root contains much lower levels of cyanogenic glycosides. Konzo typically occurs in epidemics in Mozambique, which may reflect inadequate processing of the root in times of drought or war.78
Mucilages
Mucilages are generally not chemically well defined. They are large, highly branched polymeric structures built from many different sugar and uronic acid units (uronic acids are carboxylic acids derived from sugars). They are very hydrophilic (water loving) and are capable of trapping water (and other molecules) in their cage-like structures to form a gel. Consequently, when a mucilage is mixed with water it swells to many times its original volume as it absorbs water. The saccharide linkages are in a beta configuration, which means that human digestive enzymes cannot break down mucilages. However, they can at least be partially decomposed by bowel flora into beneficial metabolites such as short-chain fatty acids (SCFA). This may explain the traditional use of slippery elm bark (Ulmus rubra) as a food for convalescents. Not only would the mucilage soothe a disturbed digestive tract, the SCFA formed in the colon would provide a source of readily absorbed and assimilated nourishment. There are some clinical and experimental studies that support the concept that mucilages can act as prebiotics, especially after their partial processing by the upper gastrointestinal tract.79–81
Mucilaginous remedies have been primarily used for their topical emollient and internal demulcent properties and their direct, if temporary, benefits in the management of inflammatory conditions of the digestive tract. This anti-inflammatory effect is probably more than just mechanical, although the protective benefits of a layer of mucilage on the digestive mucosa are obvious, especially as an extra barrier to gastric acid. The protective effect of mucilage isolated from Plantago major leaves against aspirin-induced gastric ulcer has been demonstrated in rats.82 Similar gastroprotective activity has been demonstrated for guar gum.83 It has also been shown that guar gum forms a layer closely associated with the intestinal mucosal surface when given to rats, providing a protective barrier.84
In order to assess the activity of mucilages on epithelia, a test system based on porcine buccal membranes was devised.85 While mucilages from marshmallow (Althea officinalis) and ribwort (Plantago lanceolata) showed moderate bioadhesion to epithelial tissue, polysaccharides from bladderwrack (Fucus vesiculosus) and Calendula exhibited strong adhesion. Histological studies of membranes indicated the presence of distinct polysaccharide layers on the apical membrane surface. In vitro investigations of an aqueous marshmallow root extract and its isolated mucilage on human epithelial KB cells (originating from the nasopharyngeal epithelia) and skin fibroblasts found a stimulating effect on the cell viability and proliferation of the former only.86 The marshmallow mucilage was internalised into epithelial cells, but not into fibroblasts, although it did form a bioadhesive layer on the latter. Microarray analysis indicated an upregulation of genes related to cell adhesion proteins, growth regulators, extracellular matrix, cytokine release and apoptosis. The authors concluded that their findings were consistent with the traditional use of marshmallow root for irritated mucous membranes.
Mucilages can also function as bulk laxatives and the most widespread use in this regard is ispaghula or psyllium husks, widely sold as a number of proprietary products. These are derived from the seeds of Plantago psyllium or P. ovata. However, the traditional use of mucilages such as linseed (flaxseed) and fenugreek (Trigonella foenum-graecum) as bulk laxatives often provides a valuable alternative,87 particularly where psyllium causes the characteristic side effects of bloating, abdominal pain and flatulence.88 Slippery elm powder (Ulmus rubra) can also be useful here. Mucilages can also be employed as weight loss agents and presumably act by creating a sensation of fullness.88 Since they are known to cause oesophageal obstruction, mucilages should be taken with plenty of water and not prescribed in tablet form.89 Even then, Health Canada issued a 2010 warning that glucomannan (a fibre very similar to mucilage) as a powder or capsule needs to be taken with copious amounts of water as otherwise it may cause serious choking.90
Mucilages are also used by phytotherapists to create reflex demulcency, especially to ease irritable and ticklish dry coughs. It is clear that there is no readily recognised pharmacological model for the transfer of demulcent properties directly to the bronchial mucosa: mucilages are too large to be absorbed and transported to this remote site. The emetic effect in reverse, that is the reflex effects on the tracheobronchial musculature of a soothing effect on the upper digestive tract, is instead postulated, mediated by the vagus nerve. Similar associations are used to justify the use of mucilages in painful conditions of the urinary tract.
Reflex demulcency does have experimental support. An extract of marshmallow root and its isolated mucilage demonstrated significant antitussive activity in an animal test. Doses were administered orally (50 and 100 mg/kg of mucilage) and cough from both laryngopharyngeal and tracheobronchial stimulation was depressed.91 The mucilage was as potent as some non-narcotic antitussive drugs. More recently, marshmallow mucilage was shown to be the most effective of a range of plant polysaccharides in terms of in vivo cough-suppressant activity.92 There was no negative impact on expectoration, unlike for codeine. A mechanistic study in guinea pigs demonstrated that marshmallow root mucilage (25 and 50 mg/kg, oral) produced an antitussive effect that was comparable to codeine (10 mg/kg, oral) in the model used.93 The effect was partially suppressed by a 5-HT2 receptor antagonist, suggesting an involvement of these serotonergic receptors thought to participate in the cough reflex.
There is a complex association now recognised between gastro-oesophageal reflux (GORD) and chronic cough and asthma.94–96 The link between reflux and asthma has long been suspected. In fact, there is quite long-standing evidence that reflux is an important cause of asthma in some asthmatics. Monitoring of oesophageal acidity revealed reflux in 7 out of 9 patients with persistent asthma.97 In another early study 61% of patients with asthma had reflux.98 Treatment of reflux by surgery or drugs can result in improvement or cure of asthma.98,99
While aspiration of gastric acid has been proposed as a possible cause, an effect from the reflux itself also has currency.100 One paper reported an investigation into the link between acid reflux into the oesophagus and coughing or wheezing fits in asthmatic patients.101 The scientists asked a question debated for some time among respiratory and gastrointestinal physicians: ‘Does cough cause reflux or does reflux cause cough?’ They studied more than 100 chronic adult asthmatics and found that half of all their coughs and wheezes occurred at the same time as when acid refluxed from the stomach into the oesophagus. But more importantly, they concluded that in the majority of cases it was the reflux that caused the coughing and not the other way around. The chronic cough that often follows a respiratory infection and seems to persist long after the infection is gone is probably due to acid reflux, which in turn is induced by the weakening of the oesophageal sphincter as a result of the violent coughing during the infection. Here the role of reflex demulcency is particularly valuable. Even further, if there is acid in the oesophagus, then the direct demulcent activity of the mucilage on the oesophageal wall will also soothe the irritation and thereby allay the very stimulus driving the cough or wheeze.
Animal models have confirmed that the association with GORD and cough is most likely a reflex mediated by the oesophageal afferent nerve fibres carried by the vagus nerve.102 The nocioceptive C-fibres are stimulated by the acid; however, multiple neural pathways are likely to be involved. In clinical studies, inhalation of a low concentration of a C-fibre stimulant leads to an irritating, itchy, urge-to-cough sensation that mimics the sensations associated with cough linked to respiratory tract infection, post-infection, GORD and asthma.103
Mucilages are also a class of viscous soluble fibre and in this context the properties of psyllium husks have been well studied. In particular, the mucilage from psyllium is effective at lowering blood cholesterol, as evidenced by reviews of the clinical data.104,105 Trial results suggest that it must be taken with food to be effective.106 The effective dose is around 10 g/day, with recent trials suggesting more modest effects of around a 7% reduction in LDL-cholesterol.
Viscous soluble fibre helps to retain glucose in the gut and reduce blood insulin levels after eating. Probably the main effect here is delayed gastric emptying. Psyllium seed was shown to have particular benefits in this regard, with a clear dose-related response on the effects of a glucose challenge.107 Results were not highly robust and obviously it needs to be taken with meals.105
Mucilages may compromise the absorption of nutrients and drugs (see Appendix C). However, the potential for psyllium to decrease the absorption of minerals is not proven.105
Essential oils
Phytochemistry
Essential oils are water-insoluble oily liquids that are usually colourless. Despite their being called oils, they are not related chemically to lipid oils (fixed oils) such as olive oil, corn oil and so on. Although often hydrocarbon in nature, they are unrelated to the hydrocarbon oils from the petrochemical industry. They will slowly evaporate if left in an open container, and placing a drop of oil on blotting paper can be used as a simple technique to test for adulteration with a fixed oil. If a fixed oil is present, an oily smear will remain on the paper a few days later.
Adulteration is an important issue in the trading of essential oils and many sophisticated techniques have been developed to imitate and extend essential oils. In some cases, such as oil of wintergreen, trade in the synthetic oil has completely supplanted the natural product. One survey found a large variability between the biological activities of different samples of oils and groups of oils under the same general name, for example lavender, eucalyptus or chamomile.108 This reflected on the blending, rectification and adulteration that occurs with commercial oils. Of course, this issue does not apply for oils prescribed as part of the whole plant extract, as used by phytotherapists.
Pharmacodynamics
Aromatherapy is a treatment system based on the use of essential oils. The oils may be inhaled, applied to the skin or orifices, added to baths or ingested. There is no doubt that ingested oils or those applied to the skin or added to baths are absorbed into the bloodstream in significant quantities.109 However, the use of essential oils by inhalation, mainly to influence mental function, is more controversial. In fact, in the German-speaking world, aromatherapy is typically defined as the therapeutic use of fragrances only by means of inhalation. Evidence is accumulating that this form of aromatherapy also has a pharmacological basis and is not placebo, nor is it a manipulation of emotions via the sense of smell. A recent review of the therapeutic properties of lavender essential oil exemplifies this, with 14 clinical studies demonstrating possible beneficial effects from its inhalation, including enhanced sleep, decreased anxiety and improved cognition.110
Most of the evidence for the antibacterial and antifungal activities of essential oils comes from in vitro tests, although case reports and clinical trials are scattered throughout the literature (see below). A review of the published in vitro work between 1976 and 1986 found that results were difficult to compare.111 Test methods used differed widely and important factors influencing results were frequently neglected. One of these factors was the composition of the essential oil being tested (given the existence of chemotypes and adulteration). This was highlighted in another study of commercial essential oils that found a wide variation in the antimicrobial activities of commercial samples of thyme oil, eucalyptus oil and geranium oil, among others.108
A review of more recent investigations summarised the antibacterial and antifungal activity data from around 50 studies.112 Most tests assessed either MICs or the concentration required to kill 50% of the test micro-organism culture (EC50). Many of the reviewed publications tested a range of oils and essential oil components. Wide variations in activity, depending on the test method, the essential oil tested and the test organism were tabulated.
Of 53 essential oils tested against four organisms, only a few oils exhibited remarkable activity, particularly thyme and origanum.113Pseudomonas aeruginosa was the least susceptible organism and Candida albicans the most susceptible. These oils stand out because they contain the highly active phenols thymol and carvacrol.114
Attempts have been made to identify the mechanisms behind the antifungal and antibacterial activities of essential oils and the key components responsible for this activity. Antimicrobial activity was said to parallel cytotoxic activity, suggesting a common mode of action, most probably exerted by membrane-associated reactions.115 In simple terms, it appears that the mobile and lipophilic nature of essential oil components, especially the monoterpenes, enables them to penetrate and disrupt cell membranes. Concentrations of tea tree oil that inhibit or decrease growth of Escherichia coli also inhibit glucose-dependent respiration and stimulate the leakage of intracellular potassium.116 According to a recent review, essential oils seem to have no specific cellular targets because of their great number of constituents.112 This suggests a very low risk of the development of microbial resistance against essential oils. Being lipophilic (fat-soluble) they pass through the cell wall and membranes, disrupting their structures. In bacteria, permeabilisation of the membranes is associated with the loss of ions and a reduction of membrane potential, collapse of the proton pump and depletion of the ATP pool. Essential oils can also coagulate the cytoplasm and damage lipids and proteins. Damage to the cell wall and membrane can lead to the leakage of macromolecules and eventually to lysis.
In eukaryotic cells, essential oils can provoke depolarisation of mitochondrial membranes by decreasing the membrane potential, impact ionic calcium cycling and other ionic channels and reducing the pH gradient, affecting the proton pump and the ATP pool. Chain reactions from the cell wall or membrane invade the whole cell, leading to widespread oxidative damage.112
Of five components tested for antibacterial activity, cinnamic aldehyde was the most active, followed by citral, geraniol, eugenol and menthol.117 Essential oils with high concentrations of thymol and carvacrol usually inhibit Gram-positive bacteria better than Gram-negative bacteria,118 although they still possess a good broad-spectrum activity. In another study, linalool was the most active antibacterial agent and citral and geraniol were the most effective antifungal agents.119 Essential oils with a high monoterpene hydrocarbon level were very active against bacteria but not against fungi, with the exception of dill.108 There was a negative correlation observed between cineole content and antifungal activity. In the case of tea tree oil, terpinen-4-ol was identified as the most important antimicrobial compound120,121 and cineole detracted from its antifungal activity.121
Recent research has highlighted the significant in vitro antiviral activity of essential oils.122 Most of the studies have been conducted against herpes simplex virus (HSV)-1 and HSV-2, with quite potent activities (at the parts-per-million level) being observed. A viral envelope is necessary, as growth of non-enveloped (naked) viruses is not affected by essential oils. They appear to act on enveloped viruses by affecting the viability of the free virus (virion), probably by interfering with the viral envelope (which like the cell membrane is lipid in nature).122
Clinical studies of the antimicrobial activity of essential oils have been published, with a focus on Australian tea tree oil used only topically. In an early double blind trial, 10% tea tree oil cream reduced symptoms of tinea pedis but was not effective in eradicating the fungus.123 However, a 5% tea tree oil gel was as effective as a 5% benzoyl peroxide lotion in the treatment of acne and patients experienced few side effects.124
A 2006 review of tea tree oil included published clinical trials.125 In addition to the trials summarised above, tea tree oil demonstrated efficacy as an antibacterial mouthwash or gel in three trials (with a residual effect on oral bacteria), reduced methicillin-resistant Staphylococcus aureus (MRSA) carriage, and successfully treated fungal infections of the nails, mouth and skin. Since then, topical tea tree oil has demonstrated clinical efficacy in another randomised, double blind trial assessing its value in acne patients126 and improved healing in recurrent herpes labialis after application of a 6% gel (although the results did not achieve statistical significance in this small trial).127 Tea tree oil as a topical application is also quite effective for head lice infestation.128,129
One review noted that other essential oils show bactericidal activity against oral and dental pathogenic micro-organisms and have been incorporated into mouth rinses.118
Essential oils certainly have a promising role in the management of intestinal pathogens, bacterial or otherwise. In an open label trial, oil of oregano was orally administered to 14 adult patients whose stools tested positive for the enteric parasites Blastocystis hominis, Entamoeba hartmanni and Endolimax nana.130 After 6 weeks of 600 mg/day of emulsified oil, there was a substantial reduction in detectable pathogens that correlated somewhat with an improvement in gastrointestinal symptoms. The clinical anthelmintic action of the (toxic) essential oil of wormseed (Chenopodium ambrosioides) is well documented, although this has been questioned.131
The spasmolytic activity of essential oils has been observed many times on isolated smooth muscle preparations and forms much of the basis of their use in functional gastrointestinal disorders.132 The effects of essential oils from 22 plants and some of their constituents on tracheal and ileal smooth muscle were investigated in one study.133 All of the oils had relaxant effects on tracheal smooth muscle, the most potent being angelica root, clove and elecampane root. Sixteen oils inhibited the phasic contractions of the ileal muscle preparation, the most potent being elecampane root, clove, thyme and lemon balm. Two oils (anise and fennel) increased the phasic contractions. Spasmolytic activity has also been confirmed clinically. For example, peppermint oil added to barium sulphate suspension significantly relieved colonic muscle spasm (p<0.001) during barium enema examination in a double blind, placebo-controlled study involving 141 patients.134 For more examples of the clinical spasmolytic activity of peppermint oil and its value in irritable bowel syndrome, see the peppermint monograph.
Carminatives relax sphincters and assist in the expulsion of intestinal gas. Their activity is somewhat related to spasmolytic activity. Certain essential oils or essential oil-containing herbs have been traditionally used as carminatives over many years. Oils of peppermint, sage and rosemary all relaxed Oddi’s sphincter, but peppermint was the most active.135 The carminative activity of cardamom and dill was confirmed in human studies.136 However, they were also shown to cause oesophageal reflux and should be used cautiously in susceptible patients.
Some essential oils are traditionally regarded as diuretics because they act as ‘kidney irritants’. The infusion and essential oil of juniper berries as well as terpinen-4-ol were tested for diuresis response in rats.137 On initial dosing, all three test substances exhibited an antidiuretic effect, but a significant diuretic effect was established on repeated doses, with the infusion having the strongest effect. The ‘irritant’ effect of juniper oil on the kidneys was also investigated, since there are concerns in the literature about its long-term use. No nephrotoxic effects were observed in an animal model and the authors suggested that provided high-quality oil is used (distilled from the ripe berries), concerns about the kidney irritant effects of juniper are unfounded.138 Essential oil terpenes used orally had shown some promise in dissolving small kidney stones in small, uncontrolled trials in adult patients, but these findings were not supported by controlled trials.139 However, a positive pilot trial in children suggests further investigations are desirable.
Certain essential oils (or the herbs that contain them) are used as expectorants. A proprietary product containing myrtle oil was popularly prescribed by doctors in Germany as an expectorant and mucolytic agent for acute and chronic bronchitis and sinusitis. The oil contains limonene, cineole and alpha-pinene. An expectorant activity for this oil was confirmed in a clinical trial in patients with chronic obstructive airways disease.140 Earlier animal experiments by Boyd suggested an expectorant activity for essential oils probably via influencing goblet cells to secrete more respiratory tract fluid (RTF) and mucus.141 In the 1940s, Boyd studied the effects of several essential oils in various experimental models.142,143 The most pronounced increase of RTF was seen after ingestion of oil of anise. Interestingly ingestion of oil of eucalyptus had a moderate effect that was not eliminated by cutting efferent gastric nerves. This finding supports the premise that essential oils do not act as reflex expectorants (see later).
One intriguing aspect of Boyd’s research is that he was able to demonstrate that inhaled essential oils also acted as expectorants. These results are summarised in Table 2.1.
Table 2.1 Expectorant effect of inhaled essential oils or their components
Year of study | Expectorants studied | Results |
---|---|---|
1968580 | Thuja oil | RTF markedly increased, as was the soluble mucin content of RTF. Effect was most marked in fall |
Anise oil | No effect at normal doses | |
Eucalyptus oil | No effect at normal doses | |
1969581 | Menthol | No change in amount of RTF, but its soluble mucin content was increased |
Thymol | No effect at normal doses | |
1970582 | Lemon oil | RTF and its soluble mucin content increased |
1970583 | Nutmeg oil | RTF and its soluble mucin content moderately increased. Effect most pronounced in fall |
1970584 | Citral, geraniol | RTF and its soluble mucin content moderately increased |
RTF = respiratory tract fluid
The inhalation of essential oils into the lungs might exert a direct antimicrobial activity. A case report from Australia describes the successful use of inhaled eucalyptus oil to effect clinical improvement in a patient with tuberculosis.144 A 28-year-old woman presented with cough, shortness of breath, fever, night sweats, weight loss and malaise. She had been unwell for 12 months. A sputum sample was positive for Mycobacterium tuberculosis. X-ray of the chest confirmed the diagnosis of pulmonary tuberculosis.
A sedative activity following the ingestion of essential oils such as lavender is commonly recognised and was supported by early animal studies.145 More recently, a proprietary essential oil of lavender at 80 mg/day was found to be clinically effective in sub-threshold anxiety disorder. Three randomised, controlled, double blind clinical trials were identified in a review, demonstrating significant improvements in anxiety scores after 10 weeks of treatment with the lavender oil.146
Stimulant activity has also been attributed to some essential oils. Inhalation and oral doses of rosemary oil increased locomotor activity in mice.147 Infusions of essential oil-containing herbs are often taken as diaphoretics, especially during acute respiratory infections. In this context, it is interesting to note that a case report describes a patient who exhibited pronounced diaphoresis attributed to up to 10 cups a day of sassafras tea (not recommended because of potential carcinogenicity).148
Essential oils and essential oil components have been extensively studied in animal models for analgesic-like activity.149 Overall, 43 bioactive essential oil components were identified in one review, mainly monoterpenes. However, these findings are yet to translate into clinical applications, apart from topical use of peppermint oil or menthol (for example) in headache and joint pain (see the peppermint monograph). In contrast, the local anaesthetic activity of oil of cloves is well described and the local anaesthetic activity of lavender oil has been demonstrated in an in vivo model.150
Given their cytotoxic properties on bacterial and fungal cells, it is not surprising that essential oils have exhibited a wide range of antitumour activities against cancer cell lines.112,122 Essential oils exert cytotoxicity at levels considerably lower than their antibacterial activity (in the parts-per-million range). As in bacterial cells, the cell membrane is one of the sites of cytotoxic activity.122 This antitumour research is particularly well developed (at least at the in vitro level) for thymoquinone, a component of the essential oil from the seeds of the black cumin (Nigella sativa).151
For more information about the pharmacology of essential oils and associated anti-inflammatory, antiulcer, spasmolytic, oestrogenic, expectorant, antimicrobial and analgesic activities, see the monographs on chamomile, fennel, peppermint and thyme in Part Three.
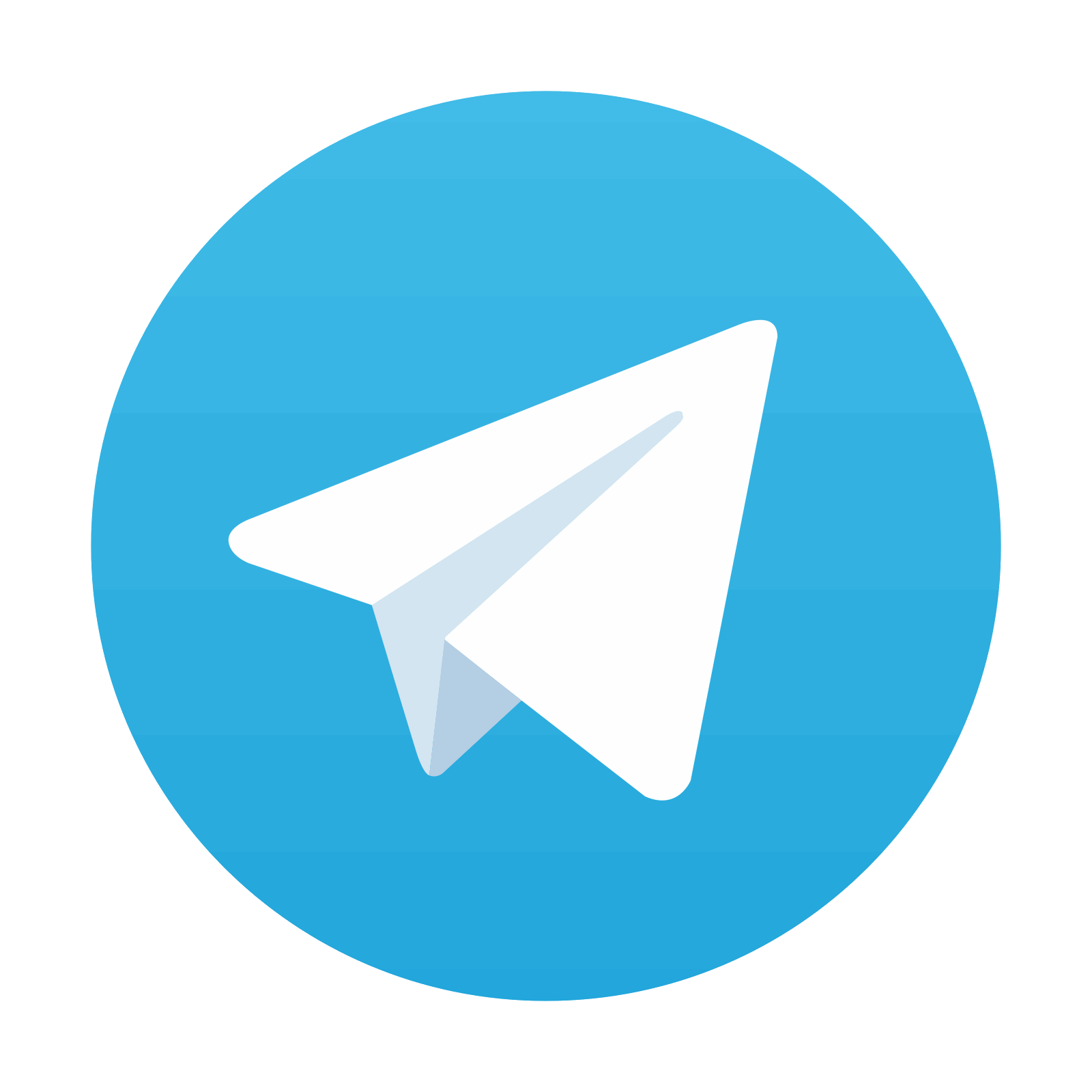
Stay updated, free articles. Join our Telegram channel

Full access? Get Clinical Tree
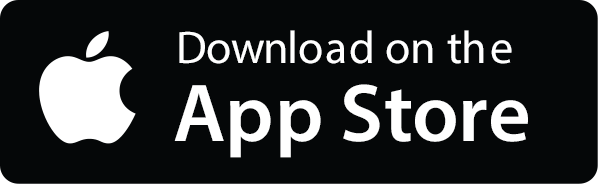
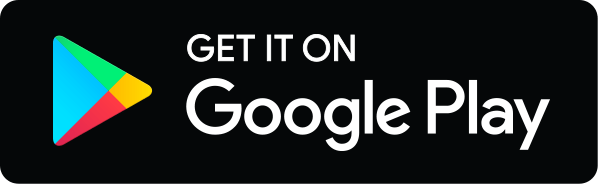