Preventing Toxic Drug Interactions and Exposures
More than 3200 prescription drugs, 300 dietary supplements, and 600 herbal products occupy pharmacy shelves in the United States,1 and more than one half of our patients cannot recite an accurate list of their medications.2 This creates a regrettable scenario when we write prescriptions for patients with whom we have little history, we see in our partners’ absence, or we rush to treat in a busy emergency department with the goal of easing disease while assuring safety in our treatment methods.
We do our best to avoid adverse drug interactions. However, for patients taking two medications, the risk of a drug interaction is 15%. This risk rises to 40% for those taking five medications and to an alarming 80% for patients taking seven or more.3 The risk of a toxic medication interaction is real considering that more than one half of noninstitutionalized adults older than 65 years take five or more different medications, and 12% use 10 or more.4 In hospitalized patients, adverse drug interactions are estimated to be as high as the fourth leading cause of death.5
EPIDEMIOLOGY
The most dangerous drug combinations in the nursing home population involve warfarin interactions with nonsteroidal anti-inflammatory drugs (NSAIDs), sulfonamides, macrolides, or quinolones; angiotensin-converting enzyme (ACE) inhibitor interactions with potassium supplements or spironolactone; digoxin interactions with amiodarone; and theophylline interactions with quinolones.6 Toxic drug interactions can occur any time two or more medications compete is such a way that their pharmacologic interaction causes a detrimental physiologic response, when a medication is prescribed in excessive amounts, or when one medication produces untoward consequences although it is prescribed according to established guidelines. This last effect is often the most difficult to predict because drug absorption and metabolism can vary with age, concomitant illness, gastric motility, pH of the gastrointestinal milieu, genetic variation, smoking, or some other obscure physiologic parameter.
THE CYTOCHROME P-450 MONOOXYGENASE SYSTEM
The system responsible for most drug metabolism in the human body is the cytochrome P-450 monooxygenase system (CYP-450). Composed of more than 30 heme-containing isoenzymes, the CYP-450 is embedded in the lipid bilayer of the endoplasmic reticulum of hepatocytes, enterocytes of the small intestines, and, in lesser quantities, in other tissues including the kidneys, lungs, and brain.2 The major isoenzymes responsible for drug metabolism in humans are CYP3A4, CYP2D6, CYP1A2, and CYP2C; CYP3A4 and CYP2D6 account for the bulk of drug metabolism. CYP3A4 is the most abundant CYP-450 isoenzyme in the small intestine (responsible for much first-pass drug metabolism) and in the liver7 and accounts for more than 50% of drug metabolism.2
Some drugs are metabolized by more than one CYP isoenzyme, so that inhibition in its metabolism by one pathway can lead to a compensatory increase in its metabolism by another. Moreover, a drug that is metabolized by one CYP pathway can, at the same time, inhibit another CYP isoenzyme such that the inhibition of the second pathway leads to the toxic accumulation of a drug that is normally metabolized by the second pathway.2
Genetic polymorphism affects drug metabolism such that some persons are extensive metabolizers and others are poor metabolizers of medications. From 5% to 10% of whites and 1% to 3% of Asians and African Americans are poor metabolizers by the CYP2D6 isoenzyme, and poor metabolizers by this pathway are at risk for tricyclic antidepressant (TCA)-induced cardiotoxicity and neuroleptic-induced side effects.8 Prodrugs that are normally converted to their active metabolites (e.g., codeine requires biotransformation to morphine) may be ineffective in poor metabolizers. CYP2C9, CYP2C10, and CYP2C19 make up the metabolically active CYP2C isoenzyme subfamily, and the CYP2C10 isoenzyme exhibits genetic polymorphism, and 20% of African Americans and Asians and up to 5% of whites are poor metabolizers. Of course, whether or not your patient is a poor metabolizer is not apparent, nor may it be clinically relevant. Measuring the O-demethylated metabolite of dextromethorphan in the urine will help determine who is an extensive metabolizer and who is a poor metabolizer.7
Cytochrome P-450 Isoenzyme Induction and Inhibition
Drug metabolism by the CYP-450 system is important to prevent accumulation of medications and substances toxic to the body, but the ability to induce the CYP-450 system can decrease with age or with organ dysfunction (e.g., cirrhosis, hepatitis).7 Isoenzyme inhibition by compounds that compete with the primary drug for the isoenzyme binding site can last several days, and the rate-limiting step in reversal of the inhibition can depend on isoenzyme turnover itself. The CYP-450 isoenzyme half-life ranges from 1 to 6 days7 and may be inhibited or induced by secondary medications.
CYP-450 enzyme synthesis may be stimulated by increases in hepatic blood flow (such as with hepatic enlargement by phenytoin therapy), and isoenzyme function may be enhanced for more rapid drug metabolism by induction from other substances (such as CYP1A2 induction by cigarette smoke or charcoal-broiled foods).7 Notwithstanding isoenzyme inhibition or induction, many commonly prescribed medications pose challenges for the physician to avoid causing toxicity when given in combination with other medications.
WARFARIN
Warfarin combined with other medications accounts for the most common dangerous drug interactions.6 Warfarin is prescribed with increasing frequency in patients with vascular stagnation states (e.g., atrial fibrillation, ventricular aneurysm), peripheral vascular disease leading to limb ischemia and stroke, and hypercoagulable conditions causing venous thromboembolic events (e.g., deep venous thrombosis, pulmonary embolism). Warfarin toxicity can lead to life-threatening intracranial and gastrointestinal hemorrhages, and the physician should pause to think about medication interactions when prescribing any drug, especially antibiotics and anti-inflammatories, to a patient taking warfarin.
Among the list of medications that inhibit warfarin metabolism and enhance its anticoagulation effects are cimetidine, selective serotonin reuptake inhibitors (SSRIs), antifungals (fluconazole, itraconazole, ketoconazole), erythromycin, (possibly clarithromycin), omeprazole, ciprofloxacin, norfloxacin, trimethoprim-sulfamethoxazole (TMP-SMX), and amiodarone.2,7 Cimetidine is a potent CYP-450 inhibitor, confounding the metabolism of many medications including warfarin by CYP1A2 inhibition of R-warfarin metabolism. Omeprazole inhibits R-warfarin metabolism and can increase anticoagulation, but lansoprazole does not and may be considered an alternative treatment in the appropriate patient needing a proton pump inhibitor.7
Of the antibiotics that enhance warfarin’s anticoagulation effect, erythromycin, ciprofloxacin, and TMP-SMX appear to have the most predictable interaction. Concomitant erythromycin use can cause a twofold increase in the international normalized ratio (INR) after 7 days of treatment. Azithromycin does not appear to increase warfarin’s anticoagulant effect, but clarithromycin should be prescribed with caution because it can increase the risk of bleeding.7 Quinolones can interact with warfarin after 2 to 16 days,7 and ciprofloxacin can cause life-threatening hemorrhage in patients formerly stable on warfarin therapy.9 Ofloxacin is less likely than ciprofloxacin to cause a dangerous interaction with warfarin, and levofloxacin and lomefloxacin are alternative quinolones with little or no effect on warfarin metabolism.7 TMP-SMX enhances anticoagulation by inhibiting CYP2C9, which metabolizes S-warfarin and can cause serious bleeding in combination with warfarin.2 Nonetheless, consider checking the INR more frequently, perhaps every other day, in patients for whom these antibiotic combinations with warfarin cannot be avoided.
Prescribing warfarin with other medications that inhibit coagulation or platelet function is risky and should only proceed when a well-defined end point supersedes the chance of causing hemorrhage, such as when low-molecular-weight heparin is given concurrently as a bridge to full anticoagulation therapy with warfarin. Synergy from the antiplatelet effect of NSAIDs and the anticoagulant effect of warfarin increases the risk of bleeding and usually occurs from the accidental combination of the two classes of medications, and although the risk of bleeding is less with cyclooxygenase (COX)-2 inhibitors than with traditional NSAIDs, the risk remains significant. Celecoxib competitively inhibits CYP2C9, the isoenzyme that metabolizes S-warfarin, and significantly increases INR and bleeding risk.10,11
Amiodarone, which is metabolized by CYP2C9 and decreases the total body clearance of both R- and S-warfarin, can increase bleeding. The increased anticoagulation effect begins 1 to 2 weeks after starting amiodarone and can last up to 3 weeks after discontinuing the antiarrhythmic. Consider reducing the warfarin dose by 25% when giving it in combination with amiodarone.7
Some medications can induce warfarin metabolism, thereby reducing the risk of bleeding. Failed anticoagulation has been observed when rifampin, carbamazepine, phenobarbital, or phenytoin is given in combination with warfarin. These medications induce S-warfarin metabolism.7
DIGOXIN
Digoxin serum concentration has a narrow therapeutic range. Its absorption is affected by gastrointestinal pH, intestinal contents, motility, and blood flow. The kidneys excrete digoxin. Any medication with anticholinergic properties that slows gastrointestinal motility or any condition that impairs digoxin excretion can increase digoxin’s concentration and the risk of digoxin toxicity. Patients at highest risk for digoxin toxicity are those who have renal insufficiency, congestive heart failure, and dehydration. Antibiotics such as clarithromycin, erythromycin, and tetracycline can alter the gut flora and increase digoxin levels, as can other medications that reduce renal clearance, such as quinidine, amiodarone, and verapamil.2 Consider reducing digoxin dosing by 50% when giving it with amiodarone, and monitor levels.
PHENYTOIN
Medications that inhibit phenytoin metabolism (CYP2C) and can favor phenytoin toxicity include cimetidine, omeprazole (impairs phenytoin elimination after 8 days), fluconazole, isoniazid, topiramate, and fluvoxamine.7 In a similar fashion of isoenzyme induction with warfarin, rifampin induces phenytoin metabolism by the CYP2C isoenzyme and can cause therapeutic failure. It sometimes seems, however, that the most likely cause for phenytoin failure is not its interactions with other medications but our patients’ failure to take the anticonvulsant in the first place.
THEOPHYLLINE
Theophylline is metabolized by CYP1A2. Cimetidine decreases theophylline clearance by 30%, and it takes approximately 2 days for theophylline to reach its new steady state when given in combination with cimetidine.12 Other medications that interfere with theophylline metabolism and increase its serum concentration are erythromycin and clarithromycin (which decrease theophylline clearance by approximately 25% after 7 days), the quinolones ciprofloxacin and norfloxacin, isoniazid (after at least 6 days of coadministration), fluvoxamine (increases theophylline concentrations two- to threefold), and oral contraceptives (which decrease theophylline clearance by 30%). As an alternative to reducing theophylline dosing and diligently measuring its serum concentration, consider prescribing other antibiotics (azithromycin, dirithromycin, oflaxacin, levofloxacin, lomefloxacin, other tuberculosis therapy), other psychiatric medications, and alternative means of contraception.7 Rifampin, carbamazepine, phenobarbital, phenytoin, and cigarette smoke induce theophylline metabolism, thereby decreasing theophylline’s serum concentration. Cigarette smokers might need twice the usual theophylline dose to achieve a therapeutic concentration; the enzyme induction effect can last for several months after smoking cessation.7
ANTIDEPRESSANTS, ANTIPSYCHOTICS, BENZODIAZEPINES, AND NARCOTICS
Although little is known about the true mechanisms by which antidepressant and antipsychotic medications work, many interactions among them and other drugs are characterized, and toxicity of one or another substance occurs by affecting the CYP-450 system. Fluoxetine, paroxetine, and sertraline, to a lesser extent, inhibit the CYP2D6 isoenzyme. Coadministration of any of these can increase the plasma concentrations of each, perhaps to toxic levels.7 Fluvoxamine inhibits CYP1A2 and increases the plasma concentrations (with accompanying clinical symptoms) of amitriptyline, clomipramine, clozapine, desipramine, imipramine, and haloperidol.7 Fluoxetine can cause delirium when given in combination with clarithromycin.7 Avoid giving SSRIs to patients taking class Ic antiarrhythmics.7
Interactions with benzodiazepines are often unexpected but may be predictable, because benzodiazepines have a significant inhibitory effect on CYP-450 isoenzymes, and other substances that induce the isoenzymes can diminish the benzodiazepine’s intended effect. Smokers experience less drowsiness than nonsmokers when taking chlordiazepoxide or diazepam due to CYP1A2 isoenzyme induction from cigarette smoke. Rifampin induces benzodiazepine metabolism (CYP3A4) and can decrease its effect. Omeprazole inhibits diazepam metabolism (CYP2C) and increases its elimination half-life by 130%.7 Fluoxetine and fluvoxamine increase the serum concentration of alprazolam, midazolam, and triazolam (CYP3A4 inhibition) and can potentiate psychomotor effects.7 Grapefruit juice increases midazolam peak serum concentrations by 50%,13 whereas nefazodone increases alprazolam and triazolam serum concentrations.7 Consider decreasing dosages by as much as 75% of the usual dose when giving benzodiazepines in these combinations.
Similar to concurrent benzodiazepine use, rifampin increases the rate of opioid metabolism (CYP2D6) and may induce narcotic withdrawal symptoms.7 Codeine is a prodrug that requires demethylation to its active form. Demethylation by the CYP-450 system is impaired in poor metabolizers and may be inhibited in extensive metabolizers who are taking drugs that compete for the same metabolic pathway, thereby reducing codeine’s analgesic effect.7 However, inhibition of the CYP3A4 metabolic pathway can enhance the effects of some narcotics. For example, surgical patients taking erythromycin who are also given alfentanil might experience prolonged respiratory depression.14 Cimetidine doubles fentanyl’s elimination half-life (CYP3A4 inhibition) and decreases meperidine clearance (CYP2D6 inhibition).7 Meperidine’s metabolite, normeperidine, is renally excreted and lowers the seizure threshold in patients with seizures. This can prove troublesome for some patients with renal insufficiency who are given meperidine for analgesia.
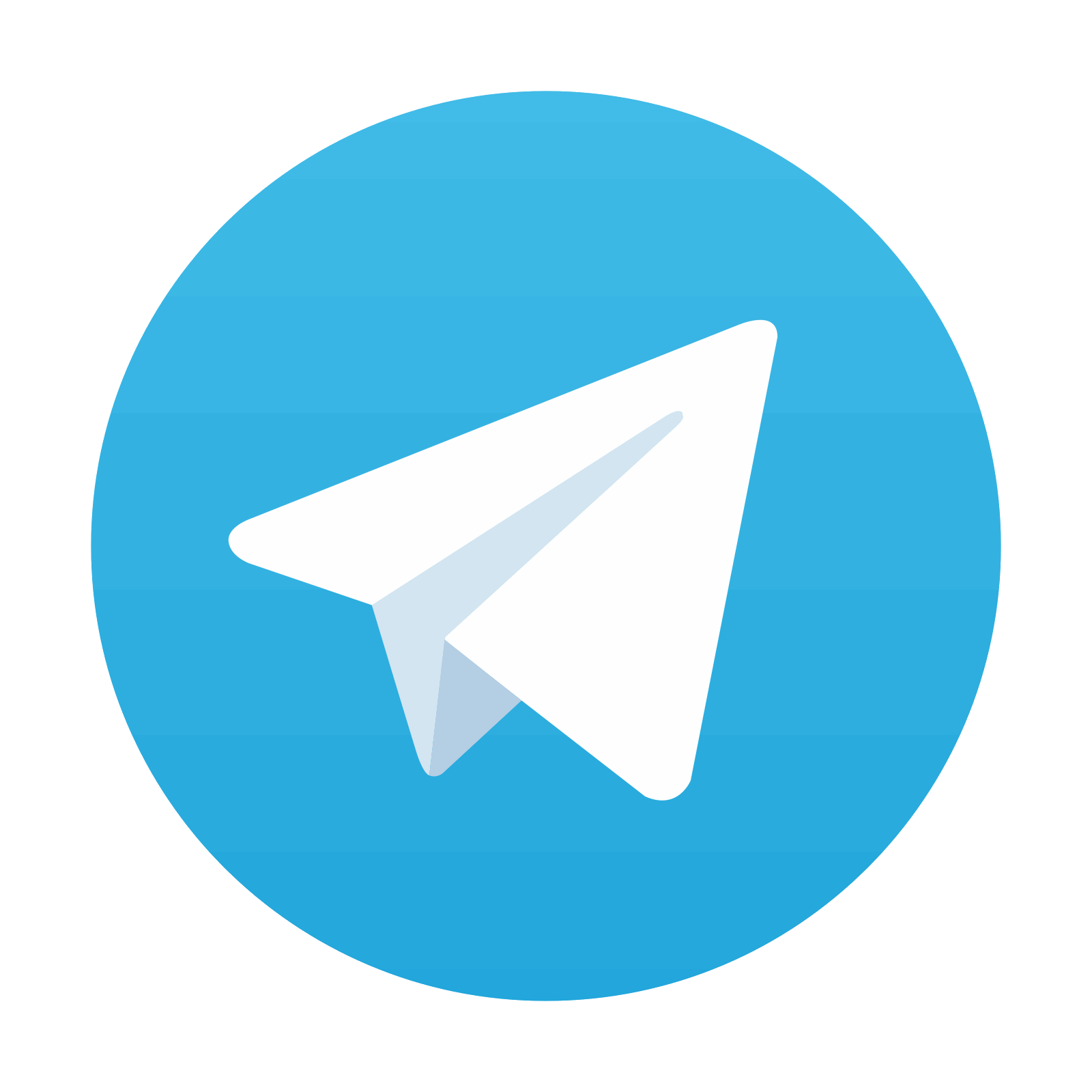
Stay updated, free articles. Join our Telegram channel

Full access? Get Clinical Tree
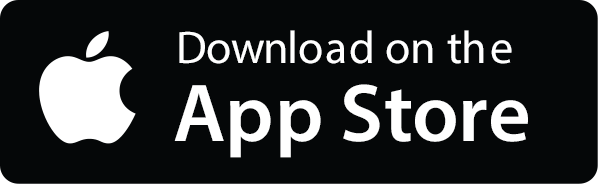
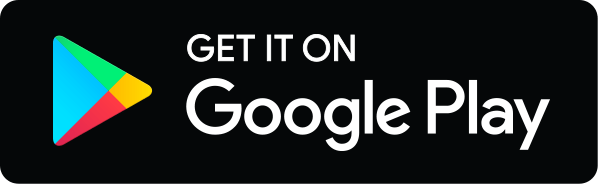