Fig. 20.1
Schematic drawing of a cochlea cross-cut. A: apical part, responsible for low-frequency hearing; B: basal part, responsible for high-frequency hearing; 1: scala tympani; 2: scala media; 3: scala vestibule; red circle: Organ of Corti without hair cells, cochlear dead region; yellow circle: organ of corti with hair cells, residual hearing possible; black arrow: cochlea implant, short array to allow acoustic low-frequency hearing in the upper cochlear regions
Nearly 188,000 individuals worldwide are fitted with a cochlear implant (NIH 2014) which allows patients to understand speech in quiet environments and, more importantly, nowadays more and more recipients have speech understanding even in noise (Lenarz et al. 2013a). Nevertheless, CI users still have difficulty in identifying pitch, melody, and timbre efficiently (Kim et al. 2012) and novel technologies have to be developed to achieve satisfactory perception of sound, leading to appreciation and enjoyment of music.
Based on the excellent results achieved in profoundly deaf patients due to advances in implant technology and improved surgical techniques, also patients with severe hearing loss predominantly in the high frequencies and substantial residual hearing in the low frequencies are implanted with the goal of hearing preservation. Hearing aids do not provide the desired benefit in speech recognition in those patients (von Ilberg et al. 2011).
20.1.1 Electrical-Acoustical Stimulation
Continuing developments in implant electrode design and improved surgical techniques have resulted in increasing incidence of preservation of residual acoustic hearing in the implanted ear following cochlear implantation (Incerti et al. 2013; Lenarz et al. 2009), leading to expanded selection criteria in adult cochlear implant patients (Arnoldner and Lin 2013).
The apical portion of the basilar membrane within the cochlea is responsible for hearing in the low frequencies (Fig. 20.1A), while the basal turn is involved with audition in the high frequencies (Fig. 20.1B). Most patients suffer from hearing loss across all frequencies or from high-frequency hearing loss with residual hearing in low frequencies. If hearing in the apical region of the cochlea has been preserved in cochlear implant patients, then the electrical stimulation of the implant is combined with a hearing aid in the same ear (electrical-acoustical stimulation, EAS) and patients can benefit from both: electrical stimulation from the CI and amplification of residual low-frequency hearing. This particular subset of patients who have residual low-frequency hearing can utilize their natural hearing to distinguish fine pitch differences that current cochlear implants cannot provide due to the limited number of separated channels (Bas et al. 2012a).
Recent data prove that EAS is a safe and effective method to provide patients with both benefits, the electrical stimulation in the deaf inner ear regions and acoustic hearing in the healthy parts (Gantz et al. 2009, 2010; Lenarz et al. 2013a; Mahmoud et al. 2014). Jurawitz et al. followed a large number of patients with different amounts of residual hearing using short and long atraumatic electrodes over a period of up to 2 years. Hearing thresholds were stable over time for the majority of patients with initial hearing preservation rates between 87 (longer electrode) and 93 % (shorter electrode) (Jurawitz et al. 2014). A study conducted by Mahmoud and colleagues followed up five EAS patients provided with the Med-El EAS system consisting of the PULSARCI 100 or SONATATI 100 implant, a FLEXEAS electrode array, and a DUET™ Speech Processor to support electrical cochlear stimulation and external hearing aid simulation. All participants showed preserved hearing in the surgical ear after implantation as measured by pure-tone audiograms and speech reception thresholds. Significant improvement in speech recognition testing over hearing aid was observed at 3 months with EAS vs. 6 months with CI-only stimulation. There were no significant complications in this cohort of patients. Adequate hearing preservation was achieved. EAS- and CI-aided conditions both showed significant improvement over hearing aid alone with better results in the EAS condition (Mahmoud et al. 2014). When comparing the percentage of correct answers to the HSM-speech test of patients provided with the FLEX system, it is obvious, that patients using the EAS instead of electrical stimulation only, perform better as shown by our own data (Fig. 20.2).
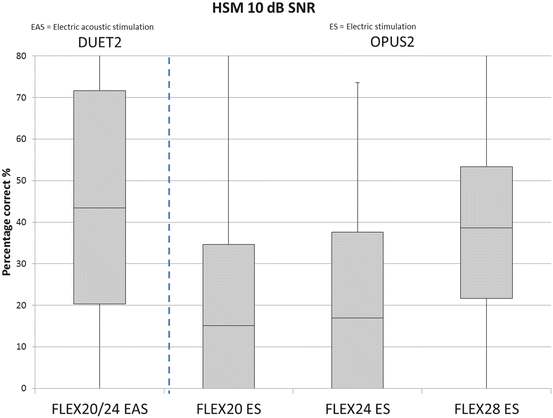
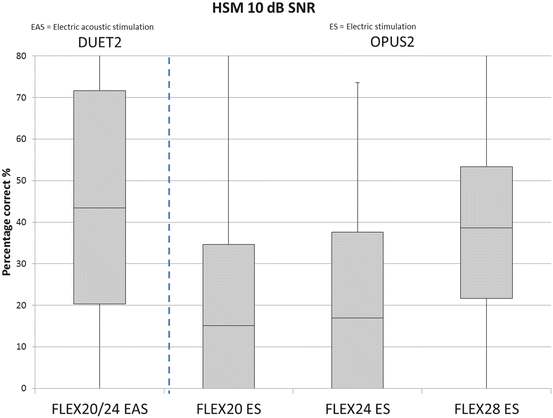
Fig. 20.2
Box-plot of the percentage of correct answers to HSM-speech test in patients using electric-acoustic-stimulation (EAS; left box) or electric stimulation only (ES; the three right boxes). Subjects were implanted with three different electrode length: 20 mm (FLEX20, n = 15), 24 mm (FLEX24) or 28 mm (FLEX28). In EAS patients (n = 15) a DUET2 speech processor was used whereas the ES patients were fitted with a OPUS2 processor (FLEX 20 ES: n = 22; FLEX24 ES: n = 14; FLEX28 ES: n = 27)
A multicenter study investigated the preservation of residual hearing in 66 subjects who received the Nucleus Hybrid L24 cochlear implant. The loss in mean air-conduction thresholds in the implanted ear for test frequencies 125–1,000 Hz was <15 dB across the population; both immediately and 1 year postoperatively. Sixty-five percent of subjects had significant gain in speech recognition in quiet, and 73 % in noise and combining residual hearing with CI gave a 22–26 % improvement in mean speech recognition scores over CI alone (p ≤ 0.01). Useful residual hearing was conserved in 88 % of subjects and speech perception was significantly improved over preoperative hearing aids, as was sound quality and quality of life (Lenarz et al. 2013a). The EAS approach has already been proven to be beneficial in infants as well. A feasibility study was conducted to evaluate whether the use of a shorter-length cochlear implant (10 mm) on one ear and a standard electrode (24 mm) on the contralateral ear is a viable bilateral option for children (n = 8; age 12–24 month) with profound bilateral sensorineural hearing loss. A secondary purpose of the study was to determine whether the ear with the shorter-length electrode performs similarly to the standard-length electrode. Preliminary results for eight children have been collected before and after the operation using the Infant-Toddler Meaningful Auditory Integration Scale (IT-MAIS). Three children showed incremental improvements in their IT-MAIS scores overtime. Early Speech Perception, Glendonald Auditory Screening Procedure word test, and Children’s Vowel word perception results indicated no difference between the individual ears for the two children tested. Performance compared with age-matched children implanted with standard bilateral cochlear implants showed similar results to the children implanted with Nucleus Hybrid S12 10-mm electrode and a Nucleus Freedom implant in contralateral ears. The use of a shorter-length cochlear implant on one ear and a standard-length electrode on the contralateral ear might provide a viable option for bilateral cochlear implantation in children with bilateral profound sensorineural hearing loss, the authors concluded (Gantz et al. 2010).
To allow the comparison of results of different clinical trials investigating residual hearing preservation in CI patients a group of experts introduced a Hearing Preservation Classification System, fulfilling the following necessary criteria: (1) classification is independent from users’ initial hearing; (2) it is appropriate for all cochlear implant users with measurable preoperative residual hearing; (3) it covers the whole range of pure-tone average from 0 to 120 dB; (4) it is easy to use and easy to understand (Skarzynski et al. 2013).
Unfortunately, post-implantation hearing loss occurs in a certain percentage of patients. Besides insertion depth, length, and mechanical characteristics of the electrode in use and the surgical technique other factors might contribute. The insertion of the electrode array into the inner ear during cochlear implantation can lead to foreign body and trauma reaction and a deterioration of the residual hearing. The postoperative result is influenced by five main factors besides multiple other biological mechanisms:
(a)
Electrode characteristics and surgical technique
(b)
Insertion trauma
(c)
Foreign body reaction
(d)
Electrode–neuron-interfacing
(e)
Long-term stability of electrode position and function
20.1.2 Electrode Characteristics and Surgical Technique
The design of the electrode array and the choice of the surgical technique are two highly relevant factors influencing the residual hearing in cochlear implant patients. Since electrode characteristics like stiffness are well known to have a high impact on preservation of residual hearing (Tamir et al. 2012), the insertion depth of the electrode array is still controversially discussed. In some studies implantation with a standard electrode array resulted in a significant risk of destroying residual hearing in the low frequencies (Ching et al. 1998; Vickers et al. 2001). And in a histological study using fresh human temporal bone specimens, smooth electrode insertions through a cochleostomy resulted in shallower insertion depths but associated significantly less intracochlear trauma compared with more forceful and deep insertions (Adunka and Kiefer 2006). Next to this, other studies did not report an effect of electrode insertion depth on residual hearing if relatively long electrode arrays of low stiffness are used (Tamir et al. 2012). While previous papers show hearing preservation rates that range from 50 to 81 % (Baumgartner et al. 2007; Skarzynski et al. 2002), the latest papers demonstrate hearing preservation rates ranging from 77 to 100 % in a large number of child and adult subjects (for review see Bas et al. 2012a). Jurawitz et al. could demonstrate on a large patient population that electrode length and insertion depth are relevant for hearing preservation with a higher percentage of preservation for a short electrode (16 mm) compared to a longer electrode (20 mm insertion depth) (Jurawitz et al. 2014).
This enormous increase of residual low-frequency hearing preservation is due to a novel generation of cochlear implants with atraumatic, often shorter electrode arrays, avoiding too deep insertion in the relevant patient population. The available cochlear implants with shortened electrode array are the “Hybrid-system” from Cochlear LTD (Sydney, Australia) with an electrode length of 10 mm (“Hybrid-S”), 15 mm (“Hybrid-M”) and 24 mm (“Hybrid-L”) and the “Flex-EAS-System” from MEDEL (Innsbruck, Austria) with an electrode length of 20 mm (“Flex 20”), 24 mm (“Flex 24”) and 28 mm (“Flex 28”). The indications for hearing aid and cochlear implant therapy are visualized in Fig. 20.3.
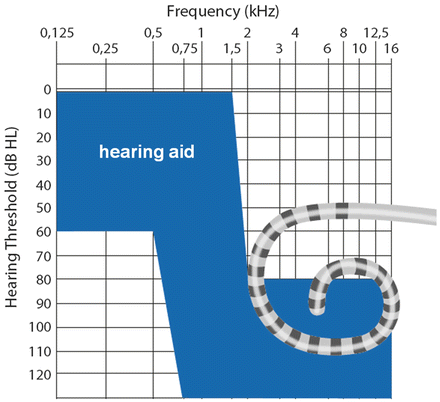
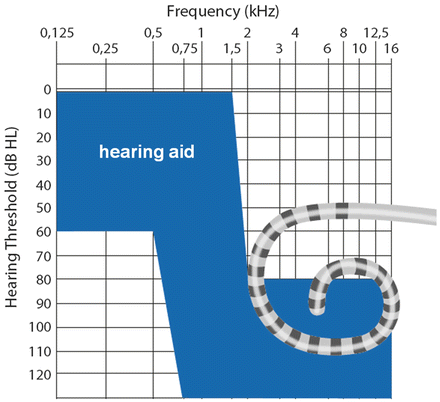
Fig. 20.3
This figure illustrates the indication range for short electrode arrays: the target ear needs to fall within the shaded region
There are different methods the surgeon has on hands to choose the right electrode length in each individual case of residual low-frequency hearing. On the one hand one should base the decision on the tone audiograms. As better the hearing in lower frequencies as shorter the electrode array should be. The second, and more precise, method to use as basis for the decision of electrode array length is imaging of the cochlea before surgery since the individual cochlear anatomy dictates the maximum electrode array insertion depth. Digital volume tomography (DVT) or high-resolution computer tomography (HRCT) are methods routinely used preoperatively in cochlear implant patients for diagnosis of e.g., hydrops or ossifications relevant for planning the surgery. Those datasets can be used to measure the length of the cochlea, resulting in an exactly individualized prediction of which electrode array length may fit into a specific cochlea (Würfel et al. 2014). Basic measurements of the cochlear dimensions, including cochlear axis height, width of the cochlear, base, and length of the cochlear base, show variability of cochlear parameters ranging from 12 to 19 % of the maximum value, proving a tremendous variability in cochlea anatomy (Avci et al. 2014). A significant positive correlation between length of the cochlear base and width of the cochlear base as well as a variability of the number of cochlear turns was variable, the mean being 2.64 ± 0.17 (949 ± 62°), ranging from 2.39 to 2.84 (859–1,024°) (Avci et al. 2014). Pelliccia and colleagues were able to prove that there is no age-relation in cochlea size, since the cochlea has already reached adult size at birth. They evaluated the cochlea length and height, basal turn lumen diameter and volume of the cochlea and concluded that the degree of deafness does not affect the length or volume of the cochlea, while it can affect height and basal turn lumen diameter (Pelliccia et al. 2014). In order to preserve residual hearing they propose a straight electrode with three landmarks on the array (the first at 16.635 mm from the tip, the second at 17.987 mm, the third at 19.34 mm) to assist the surgeon in obtaining the ideal insertion depth angle of 270° when a preoperative measurement of cochlear length by high-resolution computer tomography has been performed (Pelliccia et al. 2014).
The electrode array length and stiffness are not the only cochlear implant characteristics influencing the outcome of the implantation on residual hearing. Next to this, the positioning of the array in the scala tympani, due to precurving of the array, and the arrays diameter are relevant factors.
Conventional straight electrodes and contoured electrodes are preserving residual hearing using a cochleostomy approach (Berrettini et al. 2008; Fraysse et al. 2006; Garcia-Ibanez et al. 2009) and there are published results on the hearing preservation outcome demonstrating that there is no significant difference in hearing preservation between these non atraumatic straight and perimodiolar electrodes. Soda-Merhy and colleagues compared straight Nucleus 24 K (Cochlear Corp, Australia), MEDEL Combi 40+ (MEDEL, Innsbruck, Austria) and HiRes 90 Focus 1j (Advanced Bionics, Valencia, CA) and the perimodiolar Nucleus 24 Contour and Nucleus 24 Contour Advance (both Cochlear Corp, Australia) in a clinical trial with 48 patients using a cochleostomy approach. They reported no statistically significant difference in the preservation of residual hearing between straight or contour arrays with similar rates for both electrode designs across frequencies (Soda-Merhy et al. 2008).
When inserting the Contour Advance electrode through the round window in human temporal bones (n = 16) successful full insertions of the electrode with minimal resistance and good perimodiolar placement was infrequently achieved (Souter et al. 2011). A common finding was obstruction of electrode entry at the anteroinferior margin of the round window, which prevented optimal scala tympani positioning and often resulted in intracochlear trauma. The anteroinferior region of the round window bony margin influences the trajectory of insertion of the relatively large Contour Advance electrode as well as physically obstructing and distorting the array. A pure round window insertion is not predictable and reliable enough to be a recommended approach for the used electrode (Souter et al. 2011). This leads to the conclusion that the surgical technique is also influencing the outcome of cochlear implantation. While the surgical approach—round window vs. cochleostomy—seems to be highly important for the preservation of residual hearing using contoured arrays, the choice of the array insertion-point seems less important when using atraumatic straight electrode arrays. Straight electrodes have been used with round window and cochleostomy insertions with good results for protecting residual auditory function in the low frequencies (Havenith et al. 2013; Lenarz et al. 2013a; Skarzynski et al. 2011; Soda-Merhy et al. 2008). Studies comparing cochleostomy and round window insertion show that hearing preservation cochlear implantation can be performed via a cochleostomy or via the round window membrane itself with similar outcomes in terms of both hearing preservation rates as well as speech perception measures (Adunka et al. 2014; Nguyen et al. 2013). However, when comparing the results of two multicenter studies using the Hybrid-L electrode, better results were documented with the round-window approach (Lenarz et al. 2013a vs. US trial reported by Zhou et al. 2014). The round-window approach avoids intensive drilling on the cochlea, the formation and incorporation of bone dust, and accidental suctioning while removing bone dust.
The tip diameter is an essential criterion for the array design to improve hearing preservation results. When comparing pure-tone audiograms of patients implanted with various surgical techniques and three array designs, 3 months postoperatively, hearing preservation within 30 dB was achieved in 50 %, 50 %, and 84 % cases of patients implanted with a Contour Advance, Flex-EAS, and Hybrid-L, respectively. Best results were achieved using arrays with small-tip diameters (Nguyen et al. 2013).
To reduce the surgically induced trauma the so-called “soft surgery techniques” were developed. Lehnhardt was the first to describe a procedure for CI insertion to preserve residual low-frequency hearing (Lehnhardt 1993). Over the years improvements of Lehnhardts surgery method were developed to increase the protection of residual hearing ability (Backous 2014; Cohen 1997; Kiefer et al. 2004; Lenarz et al. 2009; Postelmans et al. 2011; Roberson 2005; Roland et al. 2005).
Parameters of the soft surgery-technique are a slow electrode insertion with the least pressure and coverage of the insertion site of the electrode array using muscle or fascia.
The insertion speed has a significant impact on various insertion characteristics as well as hearing preservation and vestibular function. Force measurements performed while inserting human electrodes in an artificial scala tympani model at progressive increase in insertion speed from 10 to 200 mm/min resulted in significant, proportional increase in the average insertion forces from 0.09 to 0.185 N and in the maximum forces from 0.18 to 0.42 N (Kontorinis et al. 2011). Additionally, the insertion speed was measured through videos of 116 human implantations showing an average insertion speed used in the surgical theaters during human cochlear implantations of 96.5 mm/min (range, 42–165.2 mm/min) and depended on the electrode type and the surgeon (Kontorinis et al. 2011). Other clinical data obtained during hearing-preserving cochlear implantation using systemic and topical steroids in conjunction with a round-window approach, a complete cochlear coverage electrode and two different electrode insertion speeds [60 mm/min (n = 18) vs. 15 mm/min (n = 22)] proved that a slow electrode insertion speed appears to facilitate full electrode insertion, reduce the occurrence of insertion resistance as well as promote preservation of residual hearing and vestibular function after cochlear implantation (Rajan et al. 2013).
Several different sealing techniques are used in human or animal studies to seal the insertion site to prevent leakage of perilymph or ingress of air and tissue formation, including no seal (Skarzynski et al. 2007) as well as an autologous muscle or fascia graft (Braun et al. 2011; Lenarz et al. 2013a; Prentiss et al. 2010). A recently published study using an animal model compared the effect of different sealing techniques—no seal, muscle graft or carboxylate cement—in relation to the surgical approach—round window and cochleostomy—on residual hearing performance after cochlea implantation. Almost no difference with regard to hearing thresholds was observed between the no seal and muscle seal groups. In all animals using the cement an almost total loss of residual hearing was observed. Therefore the use of carboxylate cement as a sealing material in cochlear implantation should be avoided even in animal studies, whereas sealing the insertion site with a muscle graft does not induce an additional tissue growth compared to omitting a seal (Burghard et al. 2014).
20.1.3 Insertion Trauma
Both, electrode characteristics and surgical technique, may lead to mechanical injury of the basilar membrane or osseous spiral lamina, which can affect the endocochlear potential, create oxidative stress, and initiate proapoptotic pathways associated with direct injury to and loss of HCs (Bas et al. 2012a). At the molecular level, insertion of a cochlear implant electrode array causes direct tissue trauma and cell losses via necrosis, but it also generates molecular events that will contribute further to a loss of any residual hearing: for example, oxidative stresses and release of proinflammatory cytokines that can lead to the initiation of programmed cell death (Eshraghi et al. 2013). Even though to our knowledge the exact mechanisms involved during cochlear implant surgery that results in loss of residual hearing is not completely understood recent findings on caspase activation, JNK activation, oxidative stress with ROS, and lipid peroxidation of cellular membranes (Eshraghi et al. 2013) give a first hint on possible targets for drug-based therapies of insertion trauma-related hearing loss. (See Chap. 19 by Bas et al. for a detailed discussion of cell death pathways associated with cochlear implantation.)
Studies on local drug delivery to the inner ear to prevent loss of residual hearing are mainly focussed on corticosteroids, JNK inhibitors, and antioxidants. It is believed that corticosteroids such as dexamethasone (DEX) can protect against death of HCs through inhibition of proinflammatory and proapoptotic triggers that initiate intrinsic and extrinsic cell death and prevents recruitment of additional macrophages to the site of injury and adjacent sites (Bas et al. 2012b; Tizard 2004). Using an animal model it was possible to attenuate progressive hearing loss associated with electrode implantation trauma when dexamethasone was applied locally into the inner ear via a miniosmotic pump (Vivero et al. 2008). Additionally in vivo studies indicate a decrease in the early elevated hearing thresholds observed following cochlear implant surgery to preoperative levels 1 month following initiation of DEX treatment (Eshraghi et al. 2011) and recovery of the CAP or ABR threshold following corticosteroid treatment in implanted inner ears (Braun et al. 2011; Eastwood et al. 2010a; Ye et al. 2007).
C–Jun N–terminal kinases (JNKs), a class of mitogen-activated protein kinases, are suggested to be involved in insertion-trauma-induced hearing loss since inhibitors of JNK-signaling have been demonstrated to protect HCs from degeneration and residual low-frequency hearing from decline after electrode insertion trauma. DJNK inhibitor-1 (DJNKI-1, also known as AM-111) treatment substantially attenuated ABR threshold shifts and changes in distortion product otoacoustic emissions (DPOAE) associated with immediate and progressive auditory dysfunction in an animal model of electrode insertion trauma (Eshraghi et al. 2007).
Lastly, there is an increasing role for antioxidants in the protection of residual hearing loss as there is increasing evidence linking high levels of oxidative stress to programmed cell death of remaining HCs. Knowledge of the mechanisms underlying noise- and drug-induced hearing loss (NIHL, DIHL) has led to the hypothesis that cochlear-implantation-related hearing loss is based on the same pathophysiology (see also Chap. 19 by Bas et al.). Noise, ototoxic drugs, and other stress agents induce free radical formation in the inner ear, which upregulate apoptotic cell death genes. If free radical formation is sufficient, cell membranes are attacked, resulting in necrotic cell death. Aerobic organisms require molecular oxygen (O2) for vital cellular processes. As the consequence of respiration and enzymatic activities, cells can generate partially reduced forms of O2 collectively referred to as “reactive oxygen species” (ROS). The gaseous molecule nitric oxide (NO) and its derivatives, also produced intracellularly, define a subclass of ROS termed reactive nitrogen species (RNS) (Ryter et al. 2007). The production of ROS/RNS in excess of an endogenous cellular capacity for their detoxification and/or utilization results in nonhomeostatic states referred to as “oxidative” or “nitrosative” stress, respectively. In addition to metabolic production, which is governed in part by O2-tension, a multiplicity of xenobiotics, drugs, cytokines, and environmental factors (i.e., solar ultraviolet radiation, ionizing radiation, and cigarette smoke) can elevate intracellular ROS production (Ryter et al. 2007). ROS can cause the progressive modification or degradation of cellular biochemicals, including DNA, protein, lipids, and carbohydrates, when produced at elevated nonphysiological concentrations. Such cumulative damage induced by ROS can lead to loss of cell function or cell death. Consequently, ROS have been implicated in the aging process, in tumorigenesis/carcinogenesis, and in the progression of various pathologies (Essick and Sam 2010), such as cardiovascular diseases, neurodegenerative disorders, and rheumatoid arthritis. In addition to their roles in subcellular damage, an emerging hypothesis argues that ROS exert physiological effects or functions in the signaling pathways that regulate cellular processes, including gene expression, growth, and regulated forms of cell death (e.g., apoptosis) (Herrera et al. 2001).
Exogenous antioxidants such as sodium thiosulfate (STS, Neuwelt et al. 1998); d-methionine (d-met, Samson et al. 2008); ascorbic acid (vitamin C, Derekoy et al. 2004); tocopherol (vitamin E, Hou et al. 2003); and N-acetylcysteine (NAC, Eastwood et al. 2010b; Lu et al. 2014) (Kannan and Jain 2000) have shown some degree of benefit against cisplatin, carboplatin, aminoglycoside, and noise-induced trauma to the inner ear in various studies (Dinh and Van De Water 2009). However, none of these studies address hearing loss associated with electrode insertion trauma and cochlear implantation except for Eastwood et al. who were able to demonstrate benefit with NAC treatment (Eastwood et al. 2010b). This benefit only pertained to the high frequencies of the basal turn and did not extend significantly to low-frequency hearing located in the apical section of the cochlea. Unfortunately, delivery of NAC to the round window prior to implantation caused a slight increase in hearing thresholds and greater amounts of osteoneogenesis, which may preclude its use locally in the protection of residual hearing (Eastwood et al. 2010b). No study was performed on evaluation of antioxidant effects on cochlear implant electrode insertion-related hearing loss.
In 2013 a single-side, randomized clinical trial started in the Department of Otolaryngology at Hannover Medical School (MHH), Germany. In this double-blind, placebo-controlled phase II study for the first time the potential hearing preservation in cochlear implant patients with residual hearing mediated by a chewable tablet with antioxidative and vasodilative effects is investigated (www.pro-hearing.eu). The tablet components, the vitamins A, C, E, and magnesium (ACEMg) belong to the pharmacological class of vitamins and micronutrients. ACEMg is a formulation composed of a micronutrient combination of 500 mg vitamin C (magnesium ascorbate), 315 mg magnesium (magnesium citrate, magnesium ascorbate, magnesium stearate), 267 mg vitamin E (α-tocopherol acetate), and 18 mg beta carotene.
The strategy has already been evaluated in a series of international human clinical trials treating temporary (military gunfire, audio player use) and permanent (stamping factory, military airbase) hearing threshold shift models (NCT00808470) to investigate the effect of ACEMg during noise exposure.
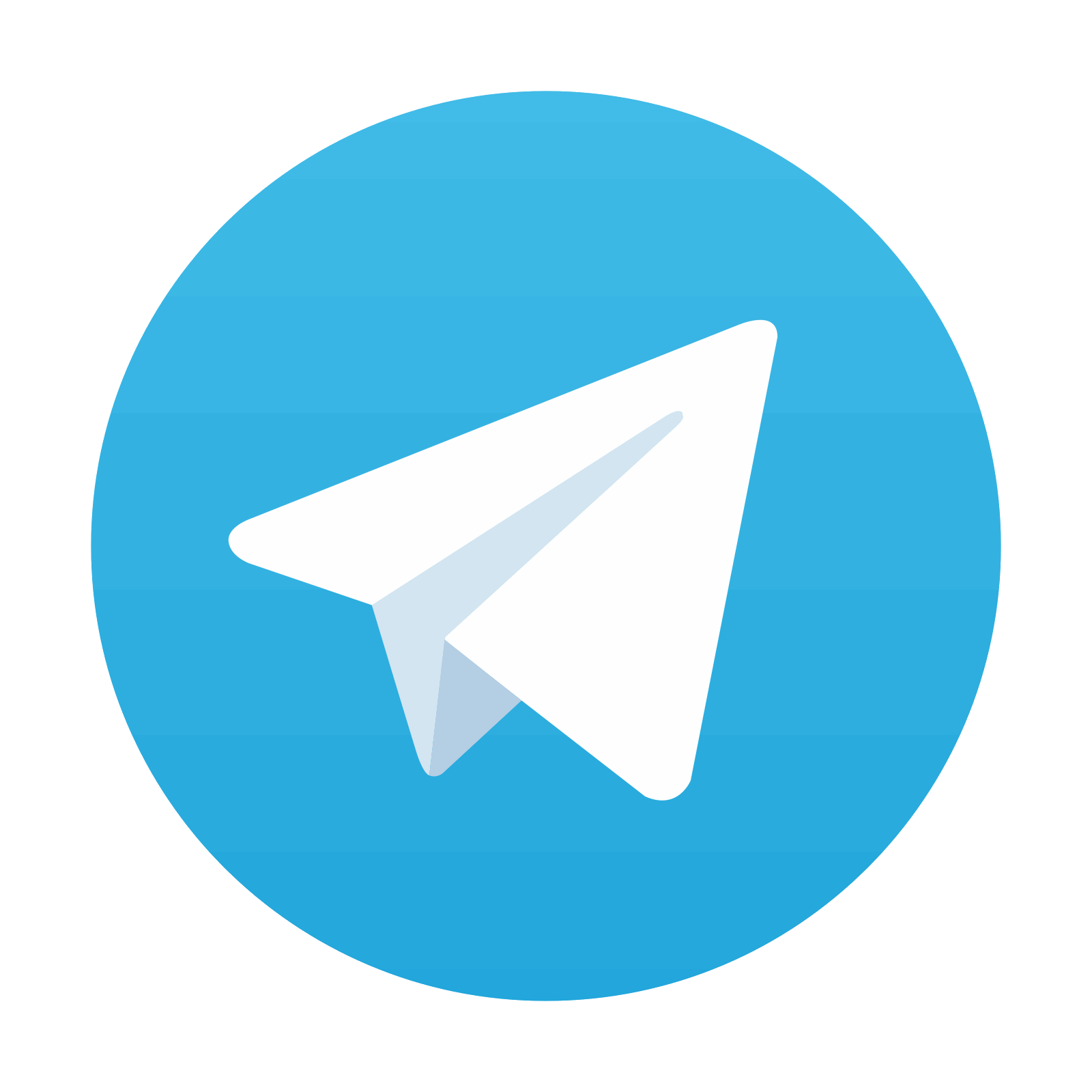
Stay updated, free articles. Join our Telegram channel

Full access? Get Clinical Tree
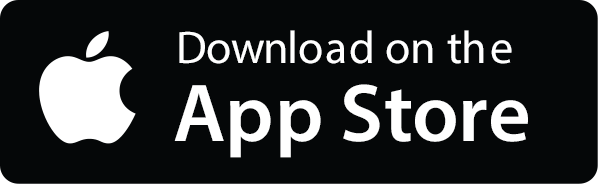
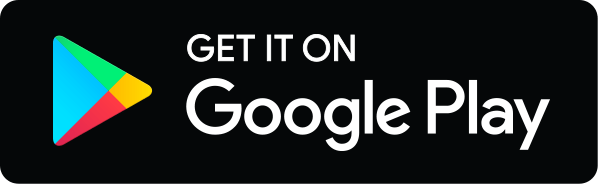