Originally, the term high-performance thin-layer chromatography referred essentially to the use of special HPTLC plates. Soon it became clear that the potential of the new stationary phase could fully be realized only if the chromatogram was miniaturized and all steps of TLC were precisely executed with the help of special instruments. Even though all modern TLC equipment can also be used with conventional plates, it should be understood that the maximum performance and all advantages of the technique are only achieved with HPTLC plates. HPTLC is often used as a synonym for instrumental TLC. Although many advantages of TLC are accessible even if very simple or no instruments are employed, it is the availability of modern, usually computer-controlled equipment that has unlocked the full power of the method and opened new fields for qualitative and quantitative applications. Work in a regulated environment and demanding issues of quality control for routine analyses have changed TLC from “quick and dirty” to a dependable, powerful, and GMP-compliant analytical technique, which has its established place in the modern laboratory. Today’s instruments, such as automatic application devices, sophisticated developing chambers, scanning densitometers, and electronic documentation systems have complemented the inherent advantages of TLC with increased reliability, better sensitivity, and improved precision and accuracy of the analytical result. The serious analyst can select instruments from different levels of automation without sacrificing quality of analysis and without losing the immense flexibility of the method. This chapter is organized according to the individual steps of the TLC process. Each section starts with a brief discussion of general aspects followed by a presentation of practical consequences. There are many parameters that can affect the result in TLC. These parameters are investigated in detail, and recommendations for appropriate choices are made. Although the focus is on the proper use of modern instrumentation, we will also show what can be achieved with simple equipment or even without. Examples are chosen with special emphasis on herbal analysis to provide a good starting point and to illustrate common problems. The suggested solutions are intended to help the practitioner also become a successful troubleshooter. The last section deals with standardization. Recommendations are given for setting parameters to obtain comparable and reproducible results. Sample Preparation General Aspects Suitable sample preparation is a prerequisite for any successful analysis. For TLC, a solid or liquid sample is either dissolved in or extracted with a suitable solvent. The key is making the analyte (target compounds of a sample) available for the analytical procedure without interferences from the sample matrix. TLC is a method that can usually tolerate a larger matrix amount than any other chromatographic technique, because the system (TLC plate) is used only once and irreversible contamination is no problem. However, the “cleaner” the sample, the better the result. This is particularly true for quantitative determinations. Generally speaking, sample preparation should be kept to a minimum and be made as simple as possible, but the analytical goal usually dictates the necessary efforts. As part of the analytical method, sample preparation has to be validated. For qualitative analyses such as identification, the only requirement is that the target compound actually be applied to the TLC plate. For quantitative determinations, the accuracy and precision of the sample preparation step need attention. In that respect, the completeness of extractions has to be ensured. Details are discussed in Chapter 6. Because of the general nature of sample preparation, we will not go further into this topic but rather refer the interested reader to the literature. The following three sections focus on some aspects that are particularly important for TLC. We begin with a discussion of dissolution and extraction procedures. Once the sample is in solution, it can be applied (see the section “Sample Application”). At that point, TLC offers some unique possibilities of further “preparing” the sample on the plate. These include chromatographic steps prior to the “actual” chromatographic separation and in situ pre-chromatographic derivatization. Dissolution and Extraction Dissolution In cases where the analyte is a single substance (e.g., a chemical reference substance) or when all components of a sample are considered the analyte (e.g., a dry plant extract), sample preparation is limited to preparing a solution of a given concentration in a suitable solvent. The term suitable primarily means that the sample is completely dissolved. When looking a bit closer into the matter, several other aspects come to mind, even though the solvent of the sample is no longer present after sample application. The solvent should: aQuestions regarding proper sampling and mechanical operations to break down the sample into particles of suitable size are beyond the scope of this text. Figure 3–1 HPTLC fingerprint of Lichen islandicus. MP: dichloro-methane, formic acid (19:1). D: UV 366 nm. Tracks 1 to 6, samples prepared with acetone; track 7, sample prepared with water. See Box 3–1 for discussion. As a rule of thumb, solutions of single substances for qualitative investigations should be prepared at a concentration of approximately 0.1 mg/mL. Toluene is a good solvent for nonpolar samples. Medium polar samples may be dissolved in mixtures of dichloromethane-methanol, and polar samples are usually dissolved in methanol with some percentage of water if necessary. Polar solvents like water, dimethylsulfoxide, acids, and bases should be avoided. It is difficult and time consuming to remove them completely from the plate after sample application, and chromatograms may be affected as illustrated in Fig. 3–1. Although it is of suitable volatility, acetone as a solvent should be avoided as well. On active layers it has the tendency to form condensation products (such as acetyl acetone), which may show up after chromatography as distinct zones (Fig. 3–2). Box 3–1 Problems That May Be Caused by the Sample’s Solvent Figure 3–1 illustrates a typical problem that can be caused by polar sample solvents such as water. A volatile solvent (acetone) was used for preparing the samples on tracks 1 to 6. The sample on track 7 is aqueous. After application of all samples (left to right) using the spray-on technique, the plate was developed immediately with a low-polarity mobile phase. Whereas acetone completely evaporates during sample application, the water of sample 7 left the application zone somewhat wet. The consequence is broadening of the applied zone; the zone assumes a doughnut shape. During separation, the individual substance zones are not straight but bent (red arrow). During the application of the aqueous sample, not only the application position but also the area nearby is deactivated. The RF values of zones are higher the less active (“wetter”) the layer is, thus causing a disturbance of the chromatogram in the area marked in red. To avoid such problems, the plate must be properly dried prior to chromatogram development. A different problem is seen in Fig. 3–2. All samples in acetone as well as the blank (neat acetone) show a distinct yellow-brown zone at RF 0.35 not seen in the samples dissolved in methanol. It might be suspected that the zone indicates an impurity of this particular batch of acetone, but independent experiments with other batches gave the same result. A possible explanation could be that during sample application, acetone forms a condensation product on the active silica gel. After chromatography, the artifact can be detected by derivatization with anisaldehyde. Ideally, all samples and reference compounds selected for analysis on the same plate should be dissolved in the same solvent to optimize the application step for achieving thin starting zones. If samples have a low solubility in a given solvent, ultrasound can be used to aid the dissolution process. Heat should only be applied if the stability of the analyte at elevated temperature has been properly assessed and the sample does not precipitate when cooled back to room temperature. Figure 3–2 HPTLC of usnic acid and lichen samples (Cetraria islandica). MP: dichloromethane, formic acid (19:1). D: anisaldehyde reagent, white light. tracks 1 and 2, lichen extracted with acetone; track 3, usnic acid (in methanol); track 4, usnic acid (in acetone); track 5, acetone blank; track 6, methanol blank. See Box 3–1 for discussion. Figure 3–3 Influence of different extraction procedures on the fingerprint of Dang gui (Angelica sinensis). MP: toluene, ethyl acetate, acetic acid (90:10:1). D: anisaldehyde reagent, white light. S: 1 hour Soxhlet extraction with hexane. U: Five-minute extraction with hexane in the ultrasonic bath. See Box 3–2 for discussion. Extraction Whenever parts of the sample are either insoluble in a given (or any) solvent or not desired to be present for separation, extraction is performed to selectively separate the analyte from the matrix. Before extraction, the sample is usually broken, cut, ground, or milled to decrease its particle size and at the same time increase its surface area. The sample is then sieved to a defined particle size. For the extraction processes, several technical solutions are available: Liquid samples or solid samples that have already been dissolved can be extracted with an immiscible liquid (liquid-liquid extraction) or with a solid (solid phase extraction; SPE). Both techniques can either be used to selectively remove matrix from the sample (cleanup) or to enrich the analyte in the extraction solvent. The extraction step can easily be automated with techniques such as accelerated solvent extraction (ASE). A special technique, solid-phase microextraction (SPME), which is widely used for trace analysis in HPLC and GC, does not yet have a place in TLC because of the technical difficulties of eluting the analyte from the SPME fiber in the off-line mode. Extractions are an essential part of the analysis of herbal drugs. For the purpose of identification by fingerprint analysis, the extraction step should be as simple as possible. Usually, a 10-minute extraction with a suitable solvent in the ultrasonic bath is sufficient (Fig. 3–3). During method development, alternative extractions (e.g., digestion, Soxhlet extraction) should be evaluated. For quantitative work, multiple extractions and spiking experiments can be performed to check completeness of the procedure. The difficult part of setting up a suitable extraction, aside from deciding on the technical details, is the proper choice of the extraction solvent. An example is given in Fig. 3–4. Each solvent has a selectivity: a “complete” extraction in the sense of all-inclusive is nearly impossible. It would be equivalent to a complete dissolution. However, complete extraction in the sense of exhaustiveness is usually desired and can be validated. Each extraction is completed with a separation of the extract from the insoluble matter. This can be done by decantation, filtration, or centrifugation. Concentration Zones and Multiple Development (Predevelopment) The discussion in this and the following section addresses techniques that can affect the sample after application to the TLC plate. The purpose of concentration zones and multiple developments usually is to focus the application zone into the shape of a thin band to improve the resolution of the separation. For concentration zones (Fig. 3–5), the effect is based on the very low retentive power of their material. Cellulose or kieselguhr is located in a strip directly below the stationary phase and afford rapid migration of most substances with the solvent front. Such substances are “concentrated” when they reach the actual stationary phase and chromatography begins. Plates with concentration zones can also be used for sample cleanup. Multiple development can serve two purposes: the separation of the analyte from the matrix and the focusing of the application zone. Figure 3–6 illustrates these approaches schematically: Box 3–2 Extraction of Sample and Chromatographic Result Sample preparation should always be as simple as possible. In many cases, particularly for qualitative analyses, a short extraction in an ultrasonic bath gives results comparable with those involving lengthy Soxhlet extractions. The chromatogram in Fig. 3–3 shows three volumes (1, 2, and 5µL) of extracts obtained by 1-hour continuous extraction (Soxhlet) and 5-minute sonication with the same solvent. Although the amount of matter extracted in the ultrasonic bath is always somewhat lower, no significant difference is seen between the qualitative results of the two methods except for a faint zone in the lower RF region. Note: There are cases, particularly if sample components are only sparingly soluble in the selected extraction solvent, in which exhaustive continuous extraction provides better results. Depending on the spectrum of extractable compounds contained in the sample, solvents of different polarity and selectivity will give different results. Let us look at the extractions compared in Fig. 3–4. The red zones close to the solvent front (upper image) belong to chlorophylls. These compounds are especially well extracted with dichloromethane and with 80% methanol but not at all with water. Caffeoyl malic acid (yellow arrow, upper image) requires at least the polarity of methanol for extraction, while the compound marked with the blue arrow on the lower image is only present in extracts with nonprotic solvents. The effect of selectivity versus polarity is seen for the compound marked with the orange arrow (upper image). This compound is extracted with dichloromethane as well as with alcohols with or without water. Straight water and ethyl acetate don’t extract it at all. Figure 3–4 Influence of different extraction solvents on the fingerprint of stinging nettle (Urtica sp.). MP: ethyl acetate, methanol, water, formic acid (50:4:4:2.5) (A) D: Natural Products reagent, UV 366 nm polar compounds. (B) D: sulfuric acid reagent, white light, nonpolar compounds. Tracks 1 and 2, standards; tracks 3 to 14, extracts of nettle obtained by sonication with 3, hexane; track 4, toluene; track 5, TBME; track 6, DCM; track 7, ethyl acetate; track 8, isopropanol; track 9, ethanol; track 10, methanol; track 11, methanol-water (8:2); track 12, ethanol-water (7:3); track 13, ethanol-water (1:1); track 14, water; track 15, extract of nettle obtained by reflux with methanol. See Box 3–2 for discussion. Pre-chromatographic Derivatization Pre-chromatographic derivatization is an easy way of changing the properties of the entire sample or those of some portions of it. In TLC, derivatization can be performed on the plate immediately after the samples have been applied. The plate can be dipped into a reagent or more elegantly the reagent can be spayed only onto the samples. Examples include the introduction of chromophoric or fluorophoric groups into the molecule.1 Very polar compounds separa-(such as acids) can be converted into less polar substances (such as esters) to improve their chromato graphic behavior. Another example is the separation of oleanolic and ursolic acid.2 The two acids are extremely difficult to separate due to their similar structure. Bromination of the double bonds during pre-chromatographic derivatization provides a convenient way of changing the molecular structure. The HPTLC plate containing the applied samples is first dipped into the reagent solution then slightly warmed to complete the bromination. The heating step also removes excess reagent from the plate. Figure 3–5 Effects of a concentration zone: separation of polar matrix and formation of bands from spotted samples (Schisandra chinensis). MP: toluene, ethyl acetate, acetic acid (70:33:3). D: sulfuric acid reagent, white light. See Box 3–3 for discussion. Figure 3–6 Multiple developments (predevelopment) for sample preparation on the plate (schematic). (A) Removal of nonpolar matrix. (B) Removal of polar matrix. (C) Predevelopment of rectangular application zones. See Box 3–3 for details and discussion Selecting the Stationary Phase General Aspects Modern TLC offers a great selection of stationary phases, which have been discussed in detail in Chapter 2. The first choice that has to be made is that of the type of layer, giving the best separation of the analytes in question. For the analysis of botanicals, silica gel is predominantly used. The high selectivity for differences in functionality of the analyte is one of the reasons. Particularly for fingerprint analysis, it is desirable to separate/analyze as many compounds of different classes as possible to obtain a comprehensive profile of the plant. Adsorption chromatography is well suited for this task. Another strong point of silica gel is its comparatively simple manufacturing process, which leads to lower costs while maintaining good batch-to-batch consistency. The use of modified silica gel layers and other stationary phases is discussed further in the section “Other Stationary Phases,” page 86. Box 3–3 Changes to the Sample After Application onto the Plate Sample preparation in the sense of changing its behavior during chromatography can be easily performed after the sample has been applied to the plate. Concentration zones are a practical tool for removing matrix and focusing the sample to a thin zone as seen in Fig. 3–5. During plate development, the sample matrix is retained at the application position of the concentration zone. It can be seen as an additional clean-up step. The image also shows how the sample was applied. On the left side of the plate, the sample was spotted with a capillary; on the right side, the sample was “streaked” with a syringe. Although applied over a large area of the concentration zone, the sample was focused into a thin zone when entering the actual chromatographic layer. Except for the band length, no qualitative difference is seen in the obtained chromatograms. Note: Unless the sample is sprayed on as a band, quantitative evaluation of plates with concentration zone is not reliable, because the sample concentration across the generated band is not defined. The examples in Fig. 3–6 illustrate in a schematic way how to use multiple developments to improve the chromatographic result. On the left plate of Fig. 3–6A, chromatography with toluene or hexane as mobile phase was used to remove the nonpolar sample matrix (paraffin, fat, or wax) and push it to the top of the plate. The cleaned sample can be chromatographed using a more polar mobile phase. Figure 3–6B also features sample clean-up. This time on the left plate, the sample components were separated from polar matrix (like sugars) by predevelopment with methanol to a distance of 1.5 cm from the lower edge of the plate. Then the plate was developed with a less polar mobile phase. Without predevelopment, the zones of the chromatogram may be disturbed, because the mobile phase cannot properly “extract” the sample from the polar matrix Figure 3–6c gives an idea about the effect of predevelopment. A large volume of a much diluted sample was applied as an 8×5-mm rectangle to avoid an additional concentration step (left plate). Predevelopment was performed with methanol, which focused the sample components of interest into a thin band (middle plate). The band can then be developed using an appropriate mobile phase. Without this step and the sample being sprayed on as band (not rectangle), the chromatogram zones are less resolved due to possible erosion of the layer or overload with matrix. Note: Details on parameters for sample application are discussed in the section “Standardization”. If we have selected silica gel as stationary phase, we still must think of several other things before chromatography can begin. In the following sections, we will address some aspects (hoping to provoke thoughts) that may never come up in an average laboratory where most procedures are based on traditional habits. Out of several possible options, we present the one that will be most useful in the majority of cases. However, there will always be exceptions, and a decision must be made from case to case. Even today there are publications of chromatographic results obtained on self-coated plates. In many Asian countries, these plates are widely used. In our opinion, it is quite possible to obtain satisfactory results on such plates if they are skillfully prepared. All of the following discussion will be based on commercially available brand-named precoated plates. Types of Stationary Phases HPTLC or TLC A principal question concerns the use of TLC or HPTLC plates, or both. Except for tradition, there is no reason why HPTLC plates should not be preferred over the conventional TLC plates. Yet a method that was optimized using TLC plates might not give exactly the same results without adaptation when HPTLC plates are employed. This fact alone is the dominant reason why analysts often hesitate to switch. We will see in the next sections some examples substantiating the assumption that it is usually not the transition to the finer particles that causes the difference in the results. Other plate properties and differences in the chromatographic chamber have greater effects. In general, HPTLC plates give better separations and reproducibility. They also allow more sensitive detection. When evaluated visually, a separation on HPTLC plates generally looks “better” and “sharper” (Fig. 3–7). Although slightly higher in price, HPTLC plates will pay off by significant savings in cost for solvents and analysis time. A typical pharmacopoeial method requires 45 minutes to 1 hour for development on TLC plates. The same or better separation can often be achieved on HPTLC plates in 8 to 20 minutes without changes to the mobile phase. The separation on the HPTLC plate as seen in Fig. 3–8 requires only one development but still gives sharper zones and an equally suitable (yet not identical) finger print to the one obtained on TLC plates with double development. As a rule of thumb, TLC methods can be adapted for use on HPTLC plates by following the conversions of Table 3–1. Aside from that, the general parameters for HPTLC as specified in the section “Standardization” should be followed. Figure 3–7 Separation of flavonoids of St. John’s wort on TLC (A)and HPTLC (B) plates. MP: ethyl acetate, dichloromethane, formic acid, acetic acid, water (100:25:10:10:11). D: Natural Products reagent, UV 366 nm. Track 1, Rutin, chlorogenic acid, hyperoside; track 2, isoquercitrin, hypericin; tracks 3 to 5, St. John’s wort. See Box 3–4 for discussion. Figure 3–8 Separation of lavender oil (Lavendula officinalis) on TLC and HPTLC plates. MP: toluene, ethyl acetate (95:5). D: anisaldehyde reagent, white light.(A) TLC plate first development. (B) TLC plate second development. (C) HPTLC plate. (D) Comparison of track 2 from B and C by video densitometry. Track 1, linalool; tracks 2 and 3, lavender oil; track 4, linalyl acetate. See Box 3–4 for discussion. Different Silica Gel Layers Normal HPTLC silica gel layers are made of irregular particles having an average size of 5 to 7 µm. Most layers are about 200 m thick. It may be advantageous to evaluate the behavior of thinner layers for a given separation problem because thickness can affect the chromatographic resolution as seen in Fig. 3–10. See Box 3–5 for further discussion. Instead of irregular silica gel, a special type of layer is made from spherical particles. According to the manufacturer, it offers faster chromatography and better separation power. When compared with Fig. 3–11A (reference plate), the selectivity of the separation on such a plate in the given case is different (Fig. 3–11B). The same is true for another specialty plate based on silica gel: a purified and “water-resistant” layer (Fig. 3–11C). It should be noted that these examples illustrate the differences that certain plate parameters can make to the chromatographic result. We neither want to generalize nor rate the performance of the mentioned plates, but there are differences. In one case, they may be used to solve a given separation problem; in the other, they may unexpectedly alter or ruin the analytical result if ignored.
3
Practical Aspects of Modern TLC
Parameter | TLS | HPTLC |
---|---|---|
Application volume | 10–20 (max 50) µ | 1–5µ(10–20%) |
Band length | 10–20 mm | 8 mm |
Developing distance | 10–15 (max 18) cm | 6 cm |
Box 3–4 TLC or HPTLC Plates?
The first impression of an HPTLC separation in comparison with that on a TLC plate is usually: wow, how sharp! This effect is due to the higher separation power but also to the shorter separation distance and smaller size of HPTLC plate. Figure 3–7 is a typical example. When looking closer at the chromatograms, only small but generally insignificant differences in separation can be found. Especially if the employed method was developed on HPTLC plates, there is no reason for using conventional plate material.
The higher separation power is also illustrated in Fig. 3–8. During first development, the TLC plate (A) is not able to separate the compound marked with a green arrow (B, C, D) from the violet zone just above it. A single development on the HPTLC plate (C) accomplished it in a much shorter time, and a separation similar to that on a TLC plate after double development (B) is obtained. When looking at the direct comparison of the track profiles (D), the similarity is even more obvious.
In cases like that of Fig. 3–9, the analytical question can only be answered if high-performance plates are employed. Usnic acid, a marker for Lichen islandicus (red arrow), produces very diffuse zones on the TLC plate, and the presence of the compound in the samples can’t be established with certainty. On HPTLC plates, this detection is significantly improved. The amount of usnic acid in the samples varies. The substance is contained in all but those on tracks 3, 10, and 14.
Support, Binder, and Indicator
HPTLC/TLC layers are available on glass plates, aluminum sheets, and polyester sheets. Although glass plates should be preferred because of their durability, there are some advantages that make sheets an attractive choice. These advantages include a much lower weight and the possibility of being easily cut into any desired size. One would expect that the support does not affect the chromatographic result, but small differences might still occur as illustrated in Fig. 3–10B and C. If exactly the same silica gel is used, these effects are predominantly due to the different binders. They may also be related to the layer’s thickness. The binder principally will affect the separation. It can also influence detection based on color reactions. Common binders found in self-coated TLC plates are gypsum, carboxymethyl cellulose, and starch. The resulting layers are soft and not very durable. The last two are not suitable for detections based on charring reactions. Starch as binder also prohibits the use of iodine as general detection reagent. Because of adduct formation, the plate background will darken. Most modern precoated HPTLC plates feature a polymeric organic binder such as poly-methacrylate. The layers are much harder and do not suffer during packaging, shipping, and storage. Silica gel G (for gypsum as binder, nowadays often falsely used, even in many pharmacopoeias, as a synonym for TLC silica gel) is hardly available as high-quality pre-coated layer. Figure 3–11E compares the performance of a plate with gypsum binder to that of plates with polymeric binder.
Most commercially available plates feature a so-called fluorescence indicator F254. It emits green light when excited at UV 254 nm. Another indicator (F254s, acid stable) emits pale blue light. Images are shown in the section “Visualization and Documentation of the Chromatogram.” Usually, it is assumed that the indicator, which is present only at very low amounts (around 2%), does not affect chromatography. The example in Fig. 3–10A and B shows that there might still be unexpected differences between plates with and without indicator. This effect must not be confused with that of different material used to make the layer (Fig. 3–11).
In conclusion, we want to emphasize that one has to be very careful when comparing the performance of two HPTLC silica gel phases and to take into account all parameters of the layer.
Figure 3–9 Detection of usnic acid in Lichen samples on TLC (A) and HPTLC (B) plates. MP: acetone, methanol, formic acid, toluene (5:5:10:80). D: anisaldehyde reagent, UV 366 nm. Tracks 1 to 6, Lichen islandicus; track 7, caffeic acid; track 8, anethole; track 9, usnic acid. See Box 3–4 for discussion.
Different Manufacturers
Even though the label on each box may describe a given HPTLC plate as made of silica gel 60 with fluorescence indicator in a given thickness, plates of different manufacturers might still give quite different results as seen in Fig. 3–12. Production parameters for the silica gel, particle size and pore distribution, as well as amount and type of the binder can vary considerably. When used for the predominantly qualitative purpose of identification by fingerprint analysis, it is usually not a question of “good or bad.” This can affect the difference in number, sequence, and color of the separated zones of the fingerprint. What is demonstrated for one chromatographic system may be different for another.
Figure 3–10 Separation of St. John’s wort extract (Hypericum perforatum) on HPTLC Si 60 F254 (Merck): effects of indicator and layer thickness (binder). MP: Ethyl acetate, dichloromethane, acetic acid, formic acid, water (100:25:10:10:11). D: Natural Products reagent, UV 366 nm. (A) Glass plate without fluorescence indicator. (B) Glass plate 0.2 mm (reference plate). (C) Aluminum foil 0.15 mm. D Glass plate extra thin 0.1 mm.
Figure 3–11 Separation of St. John’s wort extract on HPTLC Si 60: effects of specialty plates. (A) HPTLC Si 60 F254 (Merck, reference plate).(B) LiChrospher F254s (Merck). (C) Silica gel WRF254s (Merck). (D) AMD sil extra thin 0.05 mm (Macherey @ Nagel). (E) Uniplate G (Analtech).
For stability tests and quantitative measurements, two other aspects are also of importance: signal to noise ratio (a function of surface smoothness and homogeneity) and reproducibility across and from one plate to another over long periods of time (a function of quality control during the manufacturing process). Only plates from a few reputable manufacturers are able to meet these requirements.
In any case, it is advisable to develop a method for use with one type of plate from one manufacturer. If so desired, the suitability of other plate material can be shown during the validation of the method. For further discussion, see Chapter 5 and Chapter 6.
Label Symbols
There are a huge number of abbreviations describing plate properties. Different manufacturers have created their own terminology, and it is easy to get confused. Table 3–2 gives an overview of the symbols used by Merck.
Prewashing, Activation, and Handling
With storage and handling, silica gel (and also other adsorbents) will interact with the environment, adsorbing water vapor as well as fumes and dust. This has two consequences:
- The activity of the plate depends on the relative humidity of the surrounding atmosphere.
- When developed with polar solvents, “dirt zones” can be seen at the position of the solvent front and sometimes also at the position of secondary fronts.
The activity of the plate is inversely proportional to the relative humidity, and it affects the RF value of the analyte. Generally speaking, the higher the activity, the lower is the RF. As seen in Fig. 3–13, it is possible that humidity/activity changes affect different compounds to different degrees. Whereas the position of -sitosterol remains constant, the zones above and below (alkylamide) have lower RF values when humidity decreases.
Box 3–5 Plate Effects: What Is Different?
The chromatograms on the plates shown in Figs. 3–10 to 3–12 are obtained by applying the same amounts of the same samples (two sets of standards and two samples of St. John’s wort extract) onto each plate and developing these plates under exactly identical conditions. We will consider the HPTLC Si 60 F254 plate by Merck as “reference” to which all other plates will be compared.
Silica gel as stationary phase is most widely used. Let us assume we have decided to work with HPTLC material and for reasons of simplicity want to stick with one manufacturer so that we can be sure that the actual silica gel is a constant material.
Looking at Fig. 3–10, we first want to find out whether there is any effect caused by the fluorescence indicator F254. When we compare Fig. 3–10A and B, we see that chlorogenic acid (white arrow) shows significantly more tailing on the plate without indicator. The zones at the same RF in the samples are less separated.
What about the influence of the layer thickness on the chromatographic result? On three different plates (200µm, 150µm, and 100µm), we obtain the results shown in Fig. 3–10B, C, and D. Two trends can be noticed: The resolution of the two zones marked with a red arrow improves on the thinner layers and so does the intensity of zones. Otherwise, the sequence of separated zones remains constant. The difference in separation between Fig. 3–10A and C may also be due (at least in part) to the fact that a different support (and binder) is used: glass versus aluminum. However, when looking at the even thinner plate of Fig. 3–10D, the trend is seen clearly.
In Fig. 3–11, results obtained on “specialty” plates are compared with those on the reference plate (Fig. 3–11A). Figure 3–11B shows results on a LiChrospher plate and Fig. 3–11C those on a specially purified and “water-resistant” layer. The surface pH of both plates is significantly more acidic than that of “normal” HPTLC material. As a consequence, the separation of polar compounds, which are sensitive to acid-base interaction, might turn out quite differently. See the behavior of chlorogenic acid (blue-white zone on track 1, white arrow). The ultrathin automated multiple development (AMD) plate (Fig. 3–11D) fits well in this series, and we can suspect that it has an even lower surface pH. The last plate in the series (Fig. 3–11E) shows a plate with gypsum binder. The sequence of zones is similar to that on the reference plate (Fig. 3–11A), but the zones are considerably more diffuse. When comparing this plate to the one of the same manufacturer (same silica gel but with an organic binder) shown later in Fig. 3–12, it can be concluded that the binder is probably causing the decrease in separation efficiency.
Finally, let us look at “equivalent” products from different manufacturers (Fig. 3–12). At first glance, results are quite similar. When looking closer at the sharpness of zones (e.g., chlorogenic acid, white arrow) and the separation of hypericin and pseudohypericin (red zones, marked with green arrow), the differences are considerable. Note: The separation was optimized for HPTLC Si 60 F254 Merck plates. It is not the intention to rate the superiority of one plate over the others. We just want to make it clear that, like HPLC columns, plates of various kind and manufacturer will be different. Consequently, methods may need adaptation when switching the plate.
Heating a silica gel plate to 120C in an oven can maximize activity. At that temperature, adsorbed water is completely removed from the surface. High activity is not necessarily desirable, because it can cause tailing and even chemical reactions. Technically, it is rather difficult to maintain a specific activity of the silica gel for chromatography. During transport and sample application, the stationary phase is again in contact with the relative humidity of the environment. A common approach, covering most of the plate with a counter plate during sample application, is not a reproducible solution. It is more useful to equilibrate the active plate with the humidity of the surroundings by cooling it down to room temperature in a dust- and fume-free environment such as an empty desiccator. In any case, it is a good practice to record the actual relative humidity and the temperature during chromatogram development. To adjust the activity of the plate conditioning prior to development over sulfuric acid or a saturated salt solution for an extended period of time is possible (Table 3–3).
Figure 3–12 Separation of St. John’s wort extract on HPTLC Si 60: plates of different manufacturers. (A) HPTLC Si 60 F254 (Merck, reference plate). (B) Uniplate (Analtech). (C) Adsorbosil (Alltech). (D)HPKF (Whatman). (E) Nano-DURASIL (Macherey @ Nagel).
Abbreviation | Meaning |
---|---|
Si | Silica gel |
60 | Pore size 60Å(6 nm) |
F | Fluorescence indicator |
254/366 | Excitation wavelength of indicator |
s | Acid-stable indicator |
G | Gypsum binder |
H | Specially clean |
WR | Water resistant and purified |
RP | Reversed phase |
2, 8, 18 | Carbon chain length |
W | Wettable |
PSC | Preparative layer |
Alox | Aluminum oxide |
Diol | Spacer bonded propanediol mod. Si |
CN | Cyanopropylsiloxane mod. Si |
NH2 | Aminopropylsiloxane mod. Si |
Figure 3–13 Effects of relative humidity (RH) on the fingerprint (alkylamides) of Echinacea purpurea. (A) Thirty percent RH. (B) 45% RH. MP: toluene, ethyl acetate, cyclohexane, formic acid (24:6:3:0.9). D: anisaldehyde reagent, white light. Tracks 1 and 2, samples; track 3, dodecatetraenic acid isobutyl-amide; track 4, β–sitosterol.
A TLC system (layer and developing solvent) is more sensitive to changes in relative humidity the less polar the developing solvent is. Therefore, it is an important aspect of method development to find systems that are not sensitive to small changes in humidity.
Sulfuric acid | %RH | Mass (%H2SO4 in H2O) |
---|---|---|
96 | 10 | |
88 | 20 | |
75 | 30 | |
56 | 40 | |
35 | 50 | |
16 | 60 | |
3 | 70 | |
Saturated salt solution | %RH | Solubility (g/100g H2o) |
NaCl | 75 | 36.6 |
NaBr | 60 | 90.6 |
Mg(NO2)3 | 53 | 71.1 |
KSCN | 47 | 222.6 |
K2CO3 | 44 | 110.6 |
MgCl2 | 33 | 54.4 |
CH3COOK | 23 | 257.6 |
Data adapted from Geiss F. Fundamentals of Thin Layer Chromatography. Heidelberg: Müthig; 1987:207–208; and from www.d-r-h.de/hilfstabellen/klima_def_luftfeuchte.html.
Impurities on the plate accumulate not only from the laboratory atmosphere but also from packing material such as shrink-wrapping foil. When plates are stored in the original Styrofoam box, the plate on the top of a stack should be stored face down. Although for most qualitative analyses plates can be used “out of the box” without any pretreatment, it is important to consider a standardized cleaning procedure if the analytical method has to be validated (stability test, quantitation) and reproducible results are required. To remove impurities from the layer, plate prewashing or predevelopment is recommended. For prewashing, the plate is immersed into a large volume of isopropanol and soaked for several hours. During this time, impurities diffuse into the washing liquid. After drying the plate in a clean bench, the procedure is repeated with fresh solvent. Much easier to perform is predevelopment. The plate is simply developed with a strong solvent to the upper edge. Methanol is often recommended for use but may not be the most sufficient cleaning agent if the contaminants are rather nonpolar. Figure 3–14 shows the effect of prewashing of plates, which are contaminated with impurities from the packaging material. It is not a good practice to predevelop with a portion of the actual mobile phase. This way secondary fronts may be established, where impurities are collected. Normally, these zones cannot be removed during the actual chromatography.
When predevelopment is finished, the upper edge of the plate can be marked with pencil to avoid confusing the proper position for sample application (i.e., the plate should always be developed in the same direction).
Figure 3–14 Effects of prewashing on background of derivatized plate. MP: toluene, ethyl acetate (95:5). D: anisaldehyde reagent, white light. (A) Untreated plate. (B) Plate pre-washed with methanol. Samples on both plates left to right: chamomile, thyme, lavender, bitter orange, anise, spruce needle oils.
The cleaned plate is dried (and activated) in an oven at 120°C for 20 to 30 minutes. The last step is necessary to completely remove all traces of the washing solvent. In a dust- and fume-free environment, the active plate is cooled down to room temperature and equilibrated with the relative humidity of the laboratory atmosphere.
For temporary storage, a prewashed plate can be wrapped in aluminum foil. When unwrapped or taken from its original box, the TLC plate must be handled with special care to avoid contamination. It should be held by the edges when moved around, and the layer must never be touched.
Other Stationary Phases
Silica gel is most widely used as stationary phase for various tasks in herbal analysis, but there are also other stationary phases that might offer advantages for solving special analytical problems. This section gives examples illustrating the different chromatographic behavior of some special phases in comparison with silica gel. You are encouraged to experiment with the enormous flexibility accessible through those phases. Information concerning the selection of proper mobile phases as part of method development will be given in Chapter 5.
Let us first look at aluminum oxide, which also uses adsorption as the separation principle. Unfortunately, it is not available as high-performance material, and the separation power is lower than that of silica gel, but aluminum oxide offers special selectivity with respect to Lewis acid–base interaction. This can be advantageous for the separation of unsaturated compounds, differing in the type and degree of substitution as well as the number of double bonds. The separation of kavalactones is an example of aluminum oxide giving significantly better separation than silica gel (Fig. 3–15). In that case, neither the use of HPTLC material nor the change of mobile phase can compensate for the lack of selectivity of silica gel. If the silica gel is impregnated with caffeine, improved separation is possible. Further information about impregnated layers is given in the next section.
In the following examples, the separation principle is predominantly partitioning. The literature on TLC separation and isolation of natural products such as phenolics and flavonoids often refers to cellulose and polyamide. Both stationary phases have been used traditionally for several decades and do have their merits with regard to selectivity. From the viewpoint of modern TLC, most of these separations are less than optimal. HPTLC on silica gel will give superior results in most cases. Again, there are also exceptions. Figure 3–16 shows an excellent separation of procyanidines from Arrabidaea fruits on cellulose.
Chemically bonded phases based on silica gel also utilize the partition principle. A general statement comparing each phase to silica gel is very difficult, given that separation always depends on the solvent system in addition to the stationary phase. Figure 3–17 compares the fingerprint of St. John’s wort (Hypericum perforatum) on silica gel to that on a diol and an amino phase. All separations were obtained with the same mobile phase, which contained water and acid. The mobile phase was optimized for use on silica gel.
All three layers behave quite similarly but offer different selectivity with respect to compounds like hypericin/pseudohypericin (red arrow) and chlorogenic acid (blue arrow). Hypericin and pseudohypericin are only slightly different in their structure.
On the diol phase, they are separated best. When compared with the fingerprint obtained on silica gel WRF (Fig. 3–11B), there is a strong resemblance. The retention is lower on the diol phase resulting in higher RF values.
Figure 3–15 Separation of kavalactones on different stationary phases. (A) TLC aluminum oxide F254. (B) HPTLC Si 60 F254. (C) HPTLC Si 60 F254 impregnated with caffeine. All Merck. MP: (A, B) hexane, TBME (1:1); (C) hexane, TBME (3:7). D: anisaldehyde reagent, UV 366 nm. Track 1, D/L-kavain; 2, dihydrokavain; 3, methysticin; 4, dihydromethysticin; 5, yangonin; 6, desmethoxyyangonin; 7, kava root.
Figure 3–16 Separation of procyanidines from Arrabidaea. HPTLC cellulose F254 (Merck). MP: hydrochloric acid, formic acid, water (25:24:52); white light. Tracks 1 to 4, reference substances; tracks 5 to 10, samples of Arrabidaea.
Figure 3–17 Fingerprint analysis of St. John’s wort extract. (A) HPTLC Si 60 F254. (B) Diol F254s. (C) NH2 F254s. All Merck. MP: Ethyl acetate, dichloromethane, acetic acid, formic acid, water (100:25:10: 10:11). D: Natural Products reagent, UV 366 nm. See text for discussion. Structures of hypericin (D) and pseudohypericin (E).
Separation of hypericin and pseudohypericin is impossible on the amino phase.The phase overall gives the worst separation but sharpest zones and lowest RF values. This could be explained by the stronger interaction of the amino function with the OH-groups of the flavonoids and the hypericins.
What can be seen in Fig. 3–18 is different. Here, in a water-free environment, the amino phase exhibits a distinct selectivity. Paclitaxel, deacetylbaccatin, and dihydroacetylbaccatin are reference substances for the analysis of tar from Taxus species. Silica gel cannot provide sufficient selectivity to discriminate between paclitaxel and dihydroacetylbaccatin. With the same mobile phase, the amino phase gives excellent separation.
Reversed (bonded) phases not only use a different separation principle but compared with silica gel often reverse the elution order of a set of compounds. In combination with different inherent selectivity, these phases may be especially powerful for solving a given problem. Figure 3–19 and Box 3–6 feature more details. Unfortunately, bonded phases are rather expensive, and batch-to-batch conformity is not always achieved.
Figure 3–18 HPTLC of paclitaxel derivatives. (A) HPTLC Si 60 F254. (B)NH2 F254s. Both Merck. MP: chloroform, acetonitrile (4:1). D: sulfuric acid reagent, white light. Track 1, paclitaxel; track 2, deacetylbaccatin; track 3, dihydroacetylbaccatin. See text for discussion.
Modifications
Let us finally discuss the use of modified plates. Such plates are typically prepared from commercially available HPTLC plates with silica gel 60. The plate is immersed into a solution containing the modifier in an alcoholic or hydro-alcoholic solution. After drying in the oven, the plate is ready for use. Typical modifiers include acids, bases, buffers (boric acid, sodium carbonate, sodium acetate), or complex forming substances (caffeine, silver ions). Figure 3–20 illustrates the separation of ginkgolides on silica gel, which was impregnated to different extents with sodium acetate. Without impregnation, the separation is not successful. The effect of increasing the concentration of the modifier is also seen. The retention of the ginkgolides as well as the background fluorescence is increased. Best results were obtained with 4% sodium acetate.
The separation of kavalactones on caffeine-impregnated layers was already discussed in the previous section. Table 3–4 gives a summary of commonly used impregnation reagents on silicagel. Even though only a few separations on impregnated layers were reported in the field of plant analysis, we think that there is great potential to be explored.
Box 3–6 Switching the Separation Mode on a CN Phase
Let us look at some options for detecting chrysine (green arrow) in passion flower as pictured in Fig. 3–19A and B. On straight silica gel and cyano modified silica gel, the substance migrates close to the solvent front. In the samples, it doesn’t separate from co-eluting compounds, which makes positive detection difficult. What about switching the separation mode? This is taking advantage of a possible change in the elution order of the compounds. Theoretically—and also in practice—this works very well (Fig. 3–19C and D).
The cyano phase, when used in normal phase mode (the mobile phase is less polar than the stationary phase), is of similar selectivity compared with silica gel. When operated in reversed-phase mode, a very polar mobile phase is required, for instance, methanol, formic acid, water (5.5:1:4.5). Now the flavonoids of passion flower migrate to high RF values, and chrysin remains well separated in the lower half. The compound is clearly not found in any of the samples. A similar result can be obtained on a “true” reversed-phase system (RP-18W). When compared with RP-18W, the cyano phase because of its functionality offers additional specific interaction with chlorophylls (red zones).
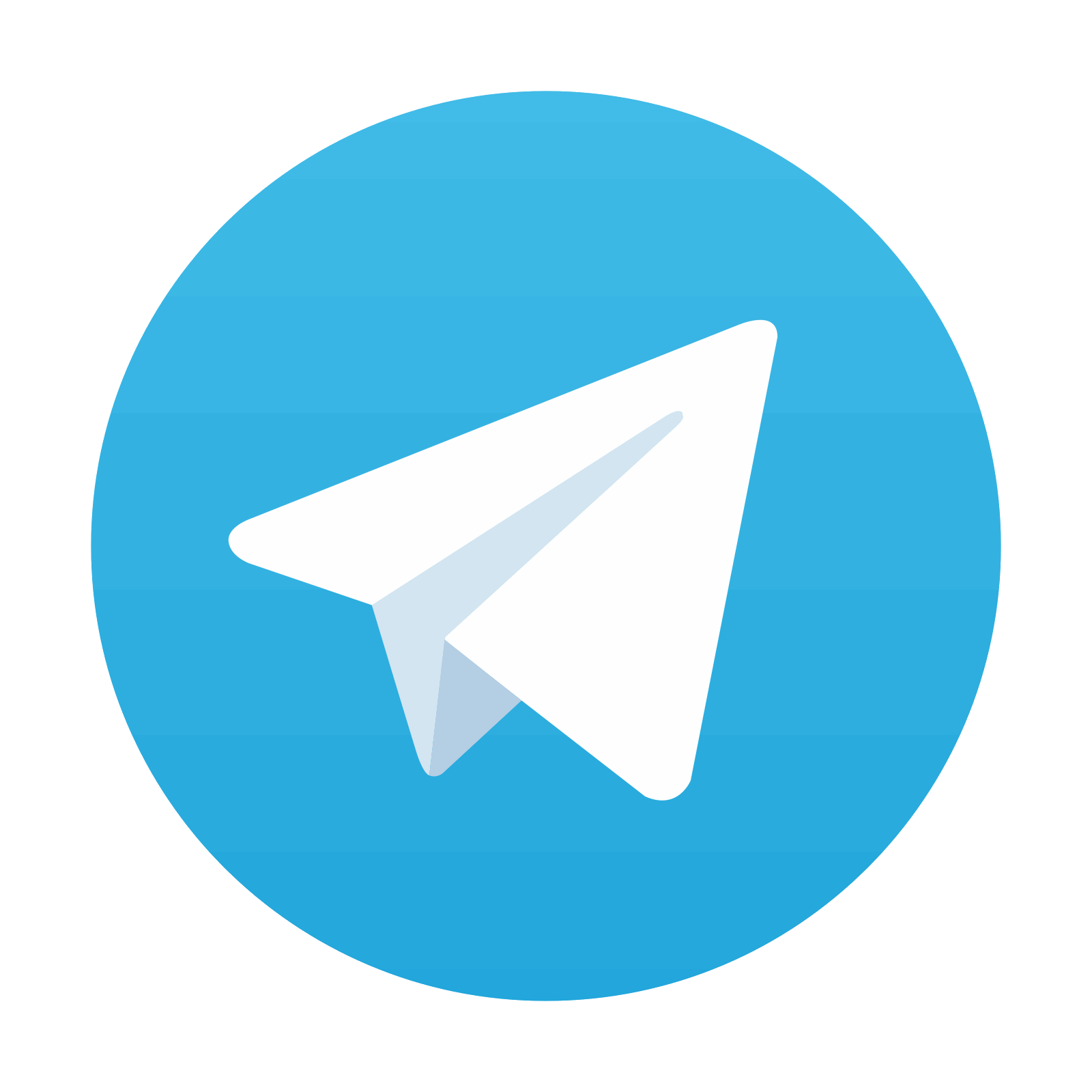
Stay updated, free articles. Join our Telegram channel

Full access? Get Clinical Tree
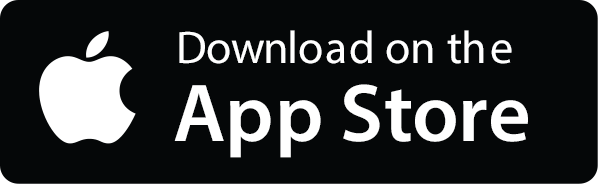
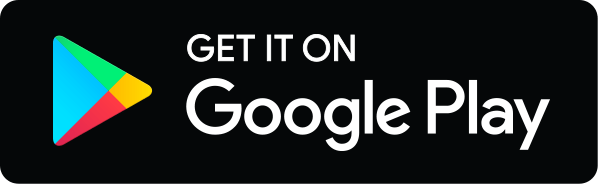