Designation
Title
F451-08
Standard specification for Acrylic Bone Cement
F639-09
Standard specification for Polyethylene Plastics for medical applications
F648-14
Standard specification for Ultra-High-Molecular-Weight Polyethylene Powder and Fabricated Form for surgical implants
F755-99(2011)
Standard specification for selection of Porous Polyethylene for use in surgical implants
F1925-09
Standard specification for Semi-Crystalline Poly(lactide) Polymer and Copolymer Resins for surgical implants
F2026-14
Standard specification for Polyetheretherketone (PEEK) Polymers for surgical implant applications
F2033-12
Standard specification for Total Hip Joint Prosthesis and Hip Endoprosthesis Bearing Materials Made of Metallic, Ceramic, and Polymeric Materials
F2313-10
Standard specification for Poly(glycolide) and Poly(glycolide-co-lactide) Resins for surgical implants with mole fractions greater than or equal to 70 % Glycolide
F2565-13
Standard guide for Extensively Irradiation-Crosslinked Ultra-High Molecular Weight Polyethylene Fabricated Forms for surgical implant applications
F2579-10
Standard specification for Amorphous Poly(lactide) and Poly(lactide-co-glycolide) Resins for surgical implants
F2695-12
Standard specification for ultra-high molecular weight Polyethylene Powder Blended with Alpha-Tocopherol (Vitamin E) and Fabricated Forms for surgical implant applications
F2759-11
Standard guide for assessment of the Ultra High Molecular Weight Polyethylene (UHMWPE) used in Orthopedic and Spinal Devices
F2820-12
Standard specification for Polyetherketoneketone (PEKK) Polymers for surgical implant applications
F2902-12
Standard guide for assessment of Absorbable Polymeric Implants
Nonabsorbable Polymers
Polymethylmethacrylate
Otto Rohm is credited with the development of perhaps the most widely used polymer in orthopaedic surgery—poly(methylmethacrylate) (PMMA)—in 1901 [1] but the material did not come into widespread use in orthopaedics until Sir John Charnley described its use for bonding prostheses to bone in the early 1960s [2]. A good review of the early work in characterizing PMMA including the evolution of the use in orthopaedics can be found in the article by Dennis Smith where he describes his collaboration with Charnley [3].
PMMA is composed of a powder polymer and a liquid monomer, which is usually mixed in a 2:1 ratio just prior to implantation. The liquid monomer is mainly (approximately 97 %) methylmethacrylate, but also contains an accelerator and a stabilizer [4]. The composition of the powder component varies by brand but contains mainly microspheres of ground PMMA or copolymer with small amounts of radiopaque substances and an initiator. Some formulations also contain a dye or antibiotics. Mixing of the polymer and the monomer creates an exothermic reaction, which can have a necrotic effect on surrounding musculoskeletal tissues. The resulting polymer will progress through several stages during curing, usually reaching a workable, dough-like consistency within a few minutes followed by the hardening phase where peak temperatures are reached. There is a published standard that describes the required characteristics of all acrylic bone cements including setting time, material properties, and maximum temperature of polymerization (Table 5.1).
PMMA is strongest in compression and weakest in tension and under shear stresses with the ultimate compressive strength lying between trabecular and cortical bone [5]. The addition of antibiotics to the cement, commonly done to prevent or treat infections, can significantly impair the strength of the cement [5, 6]. There are numerous other factors that can affect the mechanical properties of PMMA including molecular weight, mixing method, and sterilization [7–11].
Despite its widespread and successful use, relatively rare complications have been documented with the use of PMMA in orthopaedic surgery. Tissue necrosis can be caused by the high heat of polymerization or by the chemicals themselves [12, 13]. Bone cement implantation syndrome (BCIS) is usually associated with total hip arthroplasty and can be fatal for the patient. Clinical manifestations include hypoxia, hypotension, cardiac arrhythmias and in some cases cardiovascular collapse [14]. Extravasation of the cement from the site of implantation can lead to a so-called “cement emboli”, which although rare is a potentially fatal complication. [15–18] The cement can fail mechanically, leading to loosening of the implant it is meant to stabilize [5, 19, 20] or osteolysis due to the accumulation of wear particulates [21].
Polyethylene
After PMMA, the most commonly used polymer in orthopaedic surgery is polyethylene (PE). For several decades, this material has been the gold standard for bearing surfaces in total joint replacement devices. In general, the type of PE used in total joint replacements is ultra-high molecular weight polyethylene (UHMWPE) or highly cross-linked polyethylene (HXPE) [22]. Implant bearing surfaces are usually machined from ram-extruded bar stock, and then packaged and sterilized prior to shipment. Table 5.2 includes a list of some of the types of stock PE used by orthopaedic implant manufacturers.
Table 5.2
Sample of commercially available polymer products used in orthopaedic surgery
Company | Product type/trade name | Polymer type | Polymer detail |
---|---|---|---|
Arthrex | Interference screws | Bioabsorbable | PLLA |
Arthrex | Interference screws | Bioabsorbable | 70 % PLDLA 30 % Biphasic Calcium Phosphate |
Arthrex | Interference screws | Nonabsorbable | PEEK |
Arthrex | Tenodesis screws | Bioabsorbable | PLLA |
Arthrex | Tenodesis screws | Bioabsorbable | 85 % PLLA 15 % β-Tricalcium Phosphate |
Arthrex | Tenodesis screws | Nonabsorbable | PEEK |
Arthrex | Transfix | Bioabsorbable | PLLA |
Arthrex | Transfix | Bioabsorbable | 70 % PLDLA 30 % Biphasic Calcium Phosphate |
Arthrex | Graftbolt | Bioabsorbable | 70 % PLDLA 30 % Biphasic Calcium Phosphate |
Arthrex | Graftbolt | Nonabsorbable | PEEK |
Arthrex | Compression screw | Bioabsorbable | PLLA |
Arthrex | Corkscrew | Bioabsorbable | PLDLA |
Arthrex | Corkscrew FT | Bioabsorbable | PLLA |
Arthrex | Corkscrew FT | Bioabsorbable | 85 % PLLA 15 % β-Tricalcium Phosphate |
Arthrex | PushLock | Bioabsorbable | PLLA |
Arthrex | PushLock | Bioabsorbable | 85 % PLLA 15 % β-Tricalcium Phosphate |
Arthrex | PushLock | Nonabsorbable | PEEK |
Arthrex | SwiveLock | Bioabsorbable | PLLA |
Arthrex | SwiveLock | Bioabsorbable | 85 % PLLA 15 % β-Tricalcium Phosphate |
Arthrex | SwiveLock | Nonabsorbable | PEEK |
Arthrex | FASTak | Bioabsorbable | PLDLA |
Arthrex | SutureTak | Bioabsorbable | PLDLA |
Arthrex | SutureTak | Bioabsorbable | PLLA |
Arthrex | SutureTak | Bioabsorbable | 85 % PLLA 15 % β-Tricalcium Phosphate |
Arthrex | SutureTak | Nonabsorbable | PEEK |
Arthrex | SwiveLock tenodesis | Bioabsorbable | 85 % PLLA 15 % β-Tricalcium Phosphate |
Arthrex | SwiveLock tenodesis | Nonabsorbable | PEEK |
BioMet | Active articulation™ Dual mobility hip | Nonabsorbable | E1® Antiosidant infused PE |
DePuy Synthes | Plivios revolution cage for posterior lumbar interbody dusion (PLIF) | Nonabsorbable | PEEK |
Medtronic | Prevail cervical interbody device | Nonabsorbable | PEEK |
Medtronic | Bryan cervical disc | Nonabsorbable | Polyurethane |
Small Bones Innovations | STAR™ ankle | Nonabsorbable | UHMWPE |
Small Bones Innovations | rHead™ recon | Nonabsorbable | UHMWPE |
Small Bones Innovations | RE-MOTION™ total Wrist | Nonabsorbable | UHMWPE |
Small Bones Innovations | Avanta CMC | Nonabsorbable | UHMWPE |
Smith & Nephew | Healicoil regenesorb | Bioabsorbable | PLGA, β-TCP, Calcium Sulfate |
Stryker | Mobile bearing hip™ system | Nonabsorbable | Polyethylene |
Stryker | X3 bearing | Nonabsorbable | Polyethylene |
Stryker | Crossfire polyethylene | Nonabsorbable | Polyethylene |
Stryker | Solar shoulder | Nonabsorbable | UHMWPE |
Stryker | Biosteon® Wedge interference screw | Bioabsorbable | 25 % Hydroxyapatite (HA), 75 % amorphous PLLA |
Stryker | Bioabsorbable interference screw | Bioabsorbable | PLLA |
Stryker | BioZip absorbable anchor | Bioabsorbable | PLLA |
Stryker | Intraline, Zip, Twinloop, ReelXAnchors | Nonabsorbable | PEEK |
Stryker | AVS AL spacer system | Nonabsorbable | PEEK |
Synthes | ProDisc | Nonabsorbable | Polyethylene |
Zimmer | Conventional polyethylene | Nonabsorbable | GUR 1050 polyethylene resin or bar stock |
Zimmer | Sulene® | Nonabsorbable | GUR 1020 polyethylene bar stock |
Zimmer | Prolong® Highly crosslinked polyethylene | Nonabsorbable | GUR 1050 polyethylene bar stock |
Zimmer | Longevity® Highly crosslinked polyethylene | Nonabsorbable | GUR 1050 polyethylene bar stock |
Zimmer | Durasul® Highly crosslinked polyethylene | Nonabsorbable | GUR 1050 polyethylene bar stock/pre-forms |
Zimmer | Vivacit-E® Vitamin E highly crosslinked polyethylene | Nonabsorbable | GUR 1020 polyethylene resin, VitE |
The method of sterilization has critical effects on the mechanical properties of PE. Sterilization using gamma radiation produces free-radicals. If oxygen is present, the free-radicals produce chain scission and significant degradation of the mechanical strength of the implant. On the other hand, gamma irradiation without the presence of oxygen results in cross-linking of the polymer chains and can produce implants with improved wear properties [22, 23]. The addition of vitamin E, a natural antioxidant, is also thought to counteract the oxidative degradation of PE, and currently there are commercially-available PE implants containing vitamin E [24].
One of the greatest concerns about the use of PE in joint replacement bearing surfaces is the wear of the material and the effect the wear debris has on the surrounding tissues. For many years, it has been demonstrated that this wear debris leads to osteolysis and loosening of the prosthetic components [21, 25]. Wear of the polyethylene or loosening of the implants, which may be related to wear debris, remains a common reason for revision surgery after total hip [26, 27] or total knee arthroplasty [28].
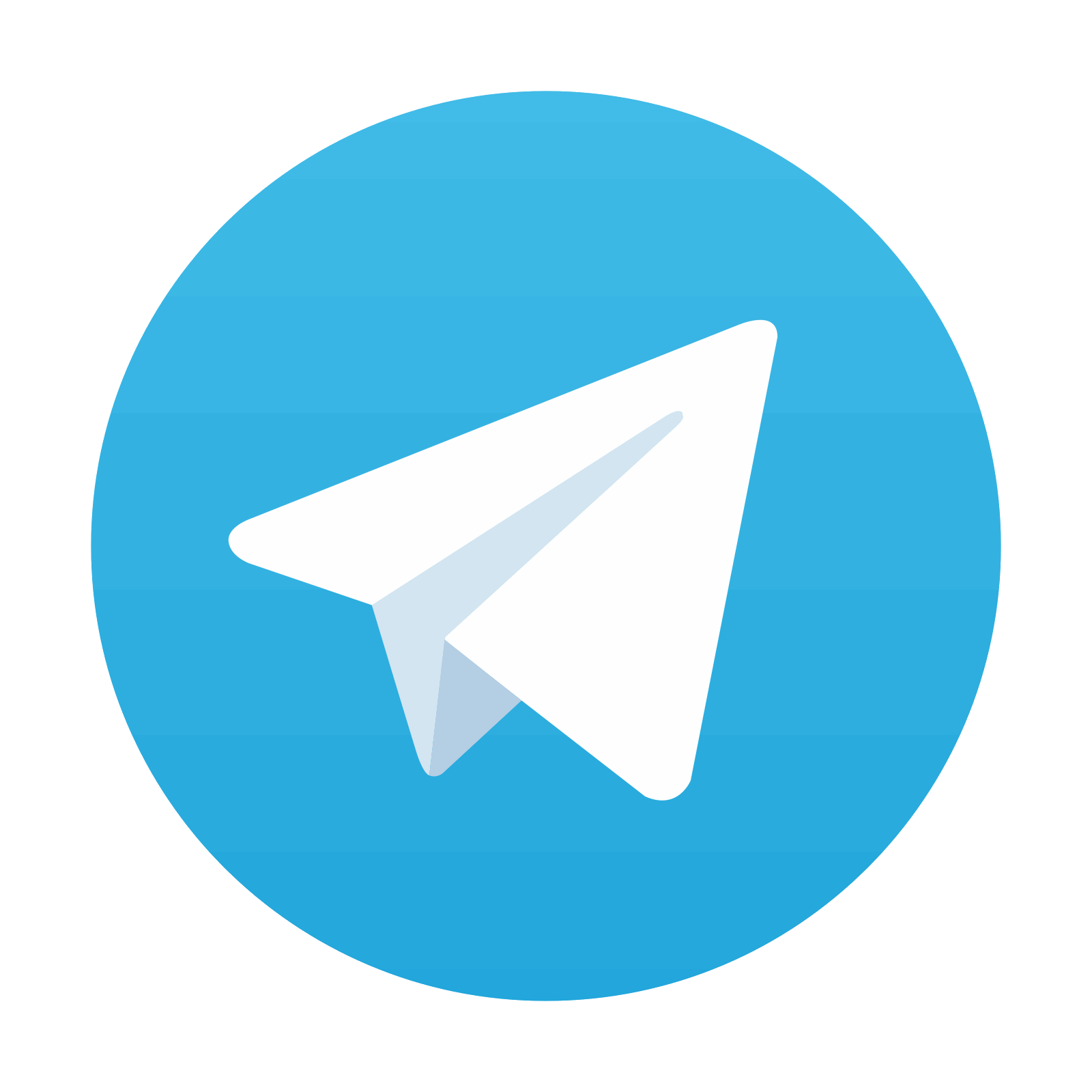
Stay updated, free articles. Join our Telegram channel

Full access? Get Clinical Tree
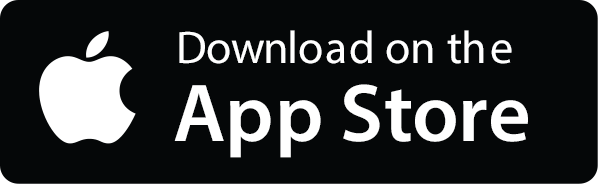
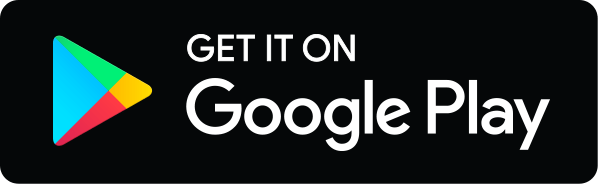