Fig. 6.1
Eyeball anatomy
The fibrous layer is composed of a rear opaque part, the sclera, and anterior and transparent, the cornea. The cornea is the transparent frontal window of the eye, is formed by several layers: an outer hydrophobic epithelium of about 50 μm thick delimited by the Bowman’s layer, followed by the hydrophilic stromal layer and finally, the thin Descemet’s membrane and the endothelium. The intact corneal epithelium permeability is very low, due to its polylaminated non-keratinized structure with zonulaocludens joints (tight junctions) between the cells of the outer layer. This structure has a protective function and exclude any movement of solutes (resulting consequently in exclusion of hydrophilic drugs with low lipophilicity or large molecular sizes) except those that occur through the apical and basal plasma membranes of surface epithelial cells [5]. The vascular tunic is formed, from back to front, by the choroid, ciliar body and iris, which form a continuous structure. The ciliary body, has several important functions, including the active secretion of aqueous humor. Much of the volume secreted goes through the trabecular meshwork to Schlemm’s canal, leaving the eye through the episcleral veins [6]. Here there is a blood-aqueous barrier formed by tight junctions between epithelial cells of the non-pigmented ciliary processes along with not fenestrated vessels of the iris, limiting the systemic all access of drugs. Finally, the retinal pigment epithelium also forms a blood-retinal barrier to accessing components that may come from systemic circulation.
Whereas the dosage form most widely used in topical ocular treatment are the eye drops (conjunctivitis, dry eye syndrome, glaucoma, iritis (anterior uveitis), keratitis); there are other parameters of ocular surface that affect pharmacotherapy, including: lower conjunctival sac capacity, blinking, tear secretion and tear drainage.
When the lower eyelid is carried forward gently with your fingers the lower conjunctival sac forms a funnel-shaped reservoir that can accommodate the instilled formulation, but the conjunctival surface cannot accommodate a larger volume than 25 μL if added quickly. When the eyelid returns to its normal position conjunctival sac capacity is reduced to less than 10 μL.
Blinking is one me the most important defense mechanisms of the eye. The blink reflex is usually fast enough to anticipate a strange body approaching at high speed to the eye. Flicker is also essential in the reformation of the tear film and activates the pump mechanism by which the tears drain. Blink rate in humans is 15–20 per minute.
Under normal baseline, the total volume required to cover the eye surface is approximately 6–8 μL, the tear secretion rate is about 1.2 μL/min and the rate of lacrimal turnover per minute is 16 % of total tear volume. However in stimulus conditions, by irritation of the conjunctiva or cornea reflects, tearing occurs. The volume of the tear film grows to about 16 ml, with a range between 5 and 6 μL [7]. Thus reflex tearing stimulated for any reason, including many parameters of eye drop formulation to enhance solubility and stability of the dosage form, cause an accelerated drop instilled washing.
The tear leaves the surface of the eye and eyelids before going to the lacrimal sac before draining to the nasolacrimal duct. In addition much of the tear film is removed by evaporation or absorption at lacrimal sac level. When blinking is prevented, tear accumulation occur, leading to spill to the skin of the eyelids and cheeks [8]. Some studies have shown that the drainage of the instilled solution is the main cause of the loss of drug in the precorneal area [9].
In response to this problem a growing interest in research related to pharmaceutical technology has been generated. A lot of work has been done on solving inherent problems of drug release, administered dose and site of action in order to design new drug delivery platforms. The design of this new group of pharmaceutical forms has focused primarily on the use of polymers as base material.
Systems for Ocular Controlled Drug Delivery Currently Investigated
Hydrogels
Hydrogels are water-swollen, cross-linked polymeric structures produced by the simple polymerization reaction of one or more monomers or by association of bonds such as hydrogen bonds and strong van der Waals interactions between chains. These systems exist in a state between rigid solids and liquid and this feature sets them apart from other forms of matter. Presently, a huge number of synthetic hydrogels is known. Hydrogels and viscous solutions, based upon the addition of hydrocolloids to simpler aqueous solutions, are the most common formulations. There is no clear cut frontier between very viscous solutions and gels in terms of biopharmaceutical results. According to Plazonnet et al. [10], aqueous gels are at the upper limit of viscous preparations, and they are formed when high molecular weight polymers or high polymer concentrations are incorporated in the formulations.
Currently, two groups of hydrogels are distinguished, namely preformed and in situ forming gels. The preformed gels can be defined mainly as hydrogels which do not undergo further modification after administration, whereas in situ gelling systems can be described as viscous liquids or suspensions that, upon exposure to physiological eye conditions (ionic strength, temperature or pH), will shift to a gel phase. Preformed gels are administered in the same way as an ointment, which is less convenient for the patient than the instillation of a viscous drop. The most common polymers used in viscous solutions are cellulose derivatives, carbomers, polysaccharides, and, recently, hyaluronic acid. The advantage offered by this last product depends upon the active ingredient and the formulation environment [11]. Polyvinyl alcohol and polyvinyl pyrolidone are also used in ophthalmic drugs. Gels permit longer residence time in the precorneal area than viscous solutions. This has encouraged researchers to work on formulations that would be (viscous) solutions in the drug vials but would gel in the conjunctival cul-de-sac. The polymers chosen to prepare ophthalmic hydrogels should meet some specific rheological characteristics. It is generally well accepted that the instillation of a formulation should influence tear behavior as little as possible. Because tears have a pseudoplastic behavior, pseudoplastic vehicles would be more suitable than Newtonian formulations, which have a constant viscosity independent of the shear rate. Pseudoplastic solutions exhibit decreased viscosity with increasing shear rate, thereby offering lowered viscosity during blinking and stability of the tear film during fixation.
A large amount of today’s research is focused on the so-called ‘smart’ or ‘intelligent’ hydrogels. A representative of this interesting class of hydrogels is a polymer system with a defined phase transition capable of abruptly swelling to many times its original size or collapsing into a compact mass when stimulated externally [12]. Smart hydrogels react in response to an external stimulus in a manner similar to many living organisms rather than to non-living organic matter [13].
The improvement in residence time of ophthalmic semisolid hydrogels is primarily based on an increase in ocular residence time as a result of a reduction in drainage rate through enhanced viscosity and mucoadhesive properties.
Preformed Hydrogels
Preformed hydrogels for topical administration in the eye can be based on natural, synthetic, or semisynthetic polymers.
Cellulose Derivatives. The pioneering group of polymers used as components of ophthalmic preformed hydrogels is the family of cellulosic derivatives. Because pure cellulose is not water soluble due to its relatively high crystallinity, cellulosic derivatives have been used for a long time as viscosifiers in collyria. Methylcellulose (MC) (Fig. 6.2) was first introduced in ophthalmic formulations in the 1940s as a mean of decreasing their fluidity [14]. Since then, cellulosic polymers have been extensively studied in human, [15–17] as well as in veterinary medicine [16, 18–20], for ocular administration. The cellulosic derivatives most commonly used in ophthalmology are: (1) Methylcellulose, (2) Hydroxyethylcellulose (HEC), (3) Hydroxypropycellulose (HPC), (4) Hydroxypropylmethylcellulose (HPMC) and (5) Sodium carboxymethylcellulose (CMC Na).
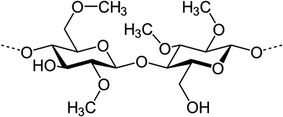
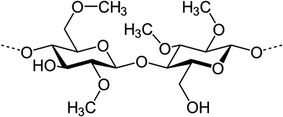
Fig. 6.2
Methylcellulose monomer
The boundary between viscous solutions and gels for cellulosic derivatives is particularly difficult to define, because data regarding the hydrocolloid concentration or the viscosity of the final formulation are not always available. These cellulosic polymers appear in several currently available commercial preparations such as Adsorbotear® (Alcon, Fort Worth, Texas), Lacril® (AUergan, Irvine, California) and Celluvisc® (AUergan, Irvine, California).
Subsequent advances in the polymers field with respect to ocular drug delivery have led to the use of poly(vinyl alcohol) (PVA); sodium hyaluronate, and carbomer, which often give better results [21–24] than celluloses. On the other hand cellulose-based hydrogels are still in focus for ophthalmical applications as ocular bandage [25].
Poly(vinyl alcohol). Scientific interest has been directed toward using other viscosifying agents. Poly(vinyl alcohol) (PVA) is a synthetic polymer commercially obtained by polymerization of vinylacetate to poly(vinyl acetate) (Fig. 6.3) and subsequent hydrolysis to PVA [26]. Polyvinyl alcohol was introduced in the early 1960’s as a mean to increase solution viscosity and, hence, prolong precorneal residence time. The presence of PVA in ophthalmic preparations has been shown to significantly delay precorneal drainage of topically applied formulations and to increase drug bioavailability as well as pharmacological effects such as miotic response to pilocarpine exposure when compared with conventional saline [27]. Some commercial products, particularly for the treatment of dry eye, are based on PVA, including HypoTearse® (IOLAB Corp., Claremont, California) and Liquifilm® (Allergan, Irvine, California).
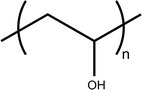
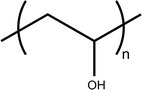
Fig. 6.3
Poly(vinyl alcohol) monomer
Sodium Hyaluronate. The actual trend in ocular delivery is to use sodium salt of hyaluronic acid (SH). The SH is a high molecular weight biological polymer composed of repeating disaccharide units of glucuronic acid and n-acetylglucosamine (Fig. 6.4), a specific ultrapure fraction being patented as Healon (Kabi Pharmacia, Sweden) by Balazs [28] in 1979. The HS is a natural polysaccharide found in skin, connective tissues, umbilical cord, vitreous body and aqueous humor. The main advantages of SH are its excellent biocompatibility, mucoadhesiveness as well as its pseudoplastic and viscoelastic behavior. Its use as a vehicle in ocular drug delivery has been extensively reviewed by Bernatchez et al. [29]. This polysaccharide is frequently proposed as a vehicle of choice in tear substitutes since it has been reported to possess a desirable protective effect against damage caused by benzalkonium chloride, a compound commonly added as a preservative in multiple dosage forms [30]. An extended residence time is one of the factors used to select artificial tears for the therapy of KCS (Keratoconjunctivitissicca), being effective in reducing common symptoms such as blurring vision, pain and photophobia. A further advantage of SH in this application is its pseudoplastic behavior. The ability of SH to prolong drug release by increasing precorneal drug residence time has been studied (mostly in animals) for several ophthalmic compounds such as pilocarpine [31–33] or, more recently, gentamicin [34]. Some commercial products containing SH are currently available being mostly indicated (for example Healon® and Viscoat®) for use as surgical aids in anterior segment procedures such as cataract extraction or intraocular lens implantation rather than for topical administration.
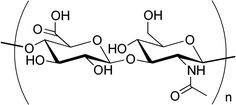
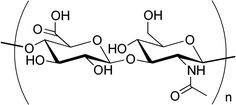
Fig. 6.4
Hyaluronic acid monomer
New efforts were directed to use this material in novel ophthalmic drug delivery platforms; recent reports in scientific literature propose the SH as solid bioadhesivedrug delivery system. Crosslinked SH films loaded with timolol maleate were successfully used to reduce intra ocular pressure in normotensive rabbits, prolonging the hypotensive effect for longer than commercial timolol maleate eye drops [35].
Carbomer. Cross-linked poly (acrylic acid) (Fig. 6.5) of high molecular weight, commercially available as Carbopol® (B.F. Goodrich Chemical Company, Cleveland, Ohio), is widely used in ophthalmology to enhance precorneal retention to the eye. The superiority of Carbopol over simple saline and suspensions in enhancing precorneal residence time [36] and drug bioavailability [37, 38] has been demonstrated by several authors. Preparation of Carbopol hydrogels is simply based on the dispersion of the polymer in water at room temperature, followed by a neutralization process with agents such as sodium hydroxide, triethanolamine, or directly with active basic compounds. The maximal viscosity is obtained at neutral pH. Carbopol offers the advantage of exhibiting excellent mucoadhesive properties when compared with others polymers (e.g., cellulose derivatives, PVA and SH). The efficacy of Carbopol in enhancing precorneal residence time has been extensively studied by incorporating tracers such as sodium fluorescein [39] or active compounds such as pilocarpine or prednisolone [24, 38, 40]. A large number of commercial ophthalmic preparations contain Carbopol, including tear substitutes such as Lacrigel® (Europhta, Monaco), Lacrinorm® (Chauvin, Montpellier, France) or formulations containing active compounds such as Iduviran® (Chauvin, Montpellier, France) and Pilopine® (Alcon, Fort Worth, Texas).
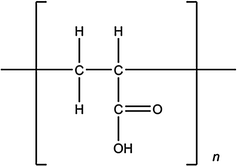
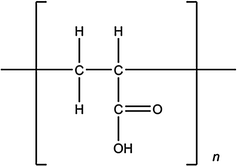
Fig. 6.5
Poly(acrylic acid) monomer
Other polymers. Other natural or synthetic polymers have also been evaluated as potential vehicles to prolong the residence time of drugs at the surface of the eye but are currently being further investigated, such as xanthan gum or chitosan are currently under investigation for topical administration. An important difference between the two polymers is the anionic character of xanthan gum, whereas chitosan exhibits positive charges. Xanthan gum has been proposed as a material for artificial tears preparations [41] as well as vehicle for drug delivery [42, 43]. Evaluating transcorneal delivery of pilocarpine from several ophthalmic formulations, Saettone et al. [44] demonstrated that the presence of 1.5 % of xanthan gum induced a significant improvement of the pharmacokinetic parameters of the drug such as area under the curve (AUC), half-life time of elimination and the mean residence time in aqueous humor. Chitosan is emerging as a polymer of interest for ophthalmic use [45–47]. Formulations based on the concept of mucoadhesion (Fig. 6.6) have been investigated to overcome the rapid elimination of instilled ophthalmic solutions. They appear less viscous than those based on traditional viscolizers. The possible advantage of chitosan over xanthan gum lies in the presence of positive charges at physiological pH on the sugar backbone of chitosan, which are supposed to interact with the negative charges of the mucus, thereby conferring a bioadhesive property to this polysaccharide. Therefore, chitosan has attracted attention for topical ophthalmic applications, for example, promising results have been obtained demonstrating that chitosan formulations remained significantly longer on the corneal surface when compared with a conventional commercial solution [48].
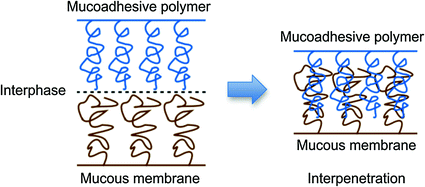
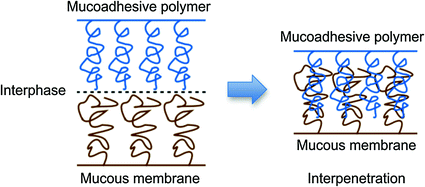
Fig. 6.6
Polymer-mucous membrane chains interpenetration in mucoadhesion phenomena
In Situ Forming Gels
The use of preformed hydrogels still has drawbacks that can limit their interest for ophthalmic drug delivery or as tear substitutes. They do not allow accurate and reproducible administration of quantities of drugs and, after administration, they often produce blurred vision, crusting of eyelids, and lachrymation. A new approach is to try to combine advantages of both, solutions and gels, such as accuracy and facility of administration of the former and prolonged residence time of the latter. Thus, in situ hydrogels can be instilled as eye drops and undergo an immediate gelation when in contact with the eye.
“Smart” hydrogels or stimuli-sensitive hydrogels or in situ forming (Fig. 6.7), are very different from inert hydrogels in that they can “sense” changes in environmental properties such as pH and temperature and respond by increasing or decreasing their degree of swelling. These sensing capabilities are attractive in many biomedical applications. The volume-changing behavior of “smart” hydrogels is particularly useful in drug-delivery applications as drug release can be desirably triggered upon environmental changes [49–51]. Temperature responsiveness is particularly useful for in situ formation of drug-delivery devices since it allows handling of the formulation in the sol-phase at room temperature and solidification of the carrier upon injection [52]. Stimuli-responsive hydrogels, especially those sensitive to temperature and pH, are attractive because these factors are variables that change in typical physiological, biological and chemical systems. Product(s) using the gellan gum technology [53], and with polymer associations like those published by the University of Nebraska researchers [54, 55], and Smart Gel® technology [56] are examples of technologies that use this approach. This field of intricately entangled polymers seems promising since new “patentable” entities might be obtained through in-depth studies of associations of well-established products.
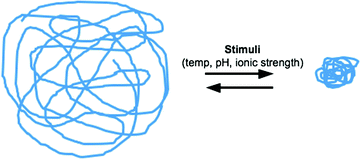
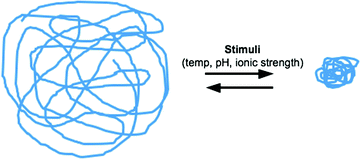
Fig. 6.7
Stimuli-sensitive hydrogels
In situ forming gels influenced by ionic strength. Ionic-strength-responsive polymers undergo their phase transitions, resulting from the different concentration of salts (e.g., ionic strength). Gellan gum is an anionic polysaccharide produced by the bacterium Pseudomonas elodea [57] which, when dispersed in aqueous solutions, undergoes a liquid-gel transition under the influence of an increase in ionic strength [58]. The acetylated form is commercially available as Gelrite® (Kelco Division of Merck and Co, USA). The sol-gel transition process is induced by the presence of monovalent or divalent ions such as Na+ and Ca2+. Some other parameters influence the phase transition, e.g., the concentration of polysaccharide, the temperature of the preparation, and the nature and the concentration of cations. Exceptional rheological properties of gellan gum such as thixotropy, pseudoplasticity, and thermoplasticity [59] are further advantages for its use in ophthalmology: the fluidity of the solution can be increased simply by shaking or slightly warming the preparation. The gellation increases proportionally to the amount of either monovalent or divalent cations present in the lacrimal fluid and in vitro experiments have demonstrated that divalent cations are more efficient in promoting sol-gel transition than monovalent ions. However, the in vivo conditions (i.e. the concentration of sodium in tears) is sufficient to induce the gellation process. Recently two other natural polymers believed to be able to form in situ gels by interacting with the lachrymal fluid have been evaluated as potential adjuvants in ophthalmic formulations [60, 61]. Carrageenans, a group of water soluble sulphated galactans extracted from red seaweed, showed similar features to gellan gum regarding their rheological behavior, gelling properties [62], and tolerance. This suggested that they could be interesting polymers for prolonging the residence time of topical ocular formulations [60]. Furthermore, the authors suggested that since these compounds are strong polyelectrolytes, they will have an identical gelling mechanism to gellan gum. Some alginates, rich in guluronic acid residues, have been demonstrated to exhibit reversible liquid-gel transition after administration and to be efficient in reducing intraocular pressure when carrying pilocarpine [61]. Also, alginate-pectine combinations and thiolated pectines were studied. Thiolation of pectin was observed to result in an increase in the gelling behavior, viscosity, and bioadhesive strength; combination of pectin and sodium alginate demonstrated good in vitro release characteristics [63].
In situ forming gels influenced by temperature. The volume-changing behavior of “smart” hydrogels is particularly useful in drug-delivery applications as drug release can be desirably triggered upon environmental changes [49–51]. Temperature responsiveness is particularly useful for in situ formation of drug-delivery devices since it allows handling of the formulation in the sol-phase at room temperature and solidification of the carrier upon injection [52]. These hydrogels are liquid at room temperature (20–25 °C) and undergo gelation when in contact with body fluids (35–37 °C), due to an increase in temperature. Different thermal setting gels have been described in the literature, including for example acrylic acid copolymers [43, 64] and N-isopropylacrylamide derivatives [65]. However, specific requirements inherent to ophthalmic administration such as tolerance have limited the choice of such polymers. Poloxamers, commercially available as Pluronic® (BASF-Wyandotte, USA), are the most commonly used thermal setting polymers in ophthalmology owing to their low toxicity, mucomimetic properties and optical clarity. They are formed by a central hydrophobic part (polyoxypropylene) surrounded by hydrophilic part (ethylene oxide). Their concentration is chosen in accordance with the desired liquid-gel transition [66]. At concentrations above 20 % w/w, poloxamers exhibit the phenomenon of reverse thermal gellation, that is, gelling upon warming up from ambient to body temperature [67]. Interestingly, the temperature of transition of poloxamers can be modulated by adding solutes or polymers such as poly(ethylene glycols) [68] or cellulosic derivatives such as MC or HPMC to the formulation. The mucomimetic property of poloxamers is supposed to be due to their hydrophobic and hydrophilic sequences simulating mucin action by adsorption of the aqueous layer of tears on the hydrophobic epithelium. However, the disadvantage of poloxamers as compared to Gelrite® lies in their mechanism of gellation. In fact, since sol-gel transition takes place as the temperature increases, accidental gellation during conservation may occur. A new attractive thermal sensitive hydrogel, Smart Hydrogel® composed of a polymeric network of poly (acrylic acid) and poloxamer, has been described by Gilchrist et al. [69]. Owing to their protective and mucomimetic action, poloxamers have also been evaluated for the treatment of dry eye [70]. Poloxamers have also been widely investigated as ocular drug delivery systems. Miller and Donovan [71] reported enhanced activity of pilocarpine in poloxamers 407 gels when compared with a simple solution, whereas Dumortier et al. [72] have shown that a thermoreversible gel does not improve the kinetic profile of morphine over a reference solution. Despite all the promising results obtained with thermoreversible gels, it remains an important drawback associated with the use of these hydrogels; the risk of gelling before administration by an increase in the ambient temperature during packaging or storage.
In situ forming gels influenced by pH. All the pH-sensitive polymers contain pendant acidic or basic groups either accept or release protons in response to changes in environmental pH. The polymers with a large number of ionisable groups are known as polyelectrolytes. Swelling of the hydrogel increases as the external pH increases in the case of weakly acidic (anionic) groups, but decreases if the polymer contains weakly basic (cationic) groups [73].
pH-sensitive hydrogels are composed of polymer chain networks crosslinked to each other and surrounded by a salt solution. A change in the pH of the solution surrounding the gel will initiate a physical process of either gel swelling or deswelling. The physical process, in general, is not instantaneous, and modelling the gel swelling/deswelling rate helps us to have a thorough understanding of the gel dynamics. This is particularly important when hydrogels are used in controlled drug-delivery devices, where the drug is released during the swelling process.
Pseudo-latexes have been defined by El-Aasser [74] as artificial latices obtained by the dispersion of a pre-existing polymer in an aqueous medium. Such systems correspond to low viscosity aqueous dispersions, which can undergo spontaneous coagulation in the conjunctival cul-de-sac owing to an increase of the local pH. The massive swelling of the particles is due to the neutralization of the acid groups contained in the polymer chain. The increase in viscosity is by several orders of magnitude [75]. In situ gelling pseudo-latexes can be prepared by two manufacturing processes; the solvent evaporation process [76] and the salting out process [77].
The uses of new technologies were recently combined to developnovel pH triggered polymeric nanoparticulate in situ gel for ophthalmic delivery of acetazolamide to enhance conjunctival permeation and precorneal residence time of the formulation. Nanoparticles were developed by nanoprecipitation method and exhibited significantly higher ex vivo transcorneal ACZ permeation than eye drops and ACZ suspension [78]. Similar findings were also described for a fluconazole pH triggered nanoemulsified in situ ophthalmic gel [79].
Inserts
This section is devoted to solid devices delivering drugs to the anterior segment of the eye that are denoted by the general name insert, originating from the Latin inserere, to introduce. Ophthalmic inserts are defined as preparations with a solid or semisolid consistency, whose size and shape are especially designed for ophthalmic application (i.e. rods or shields) [80]. These inserts are placed in the lower fornix and, less frequently, in the upper fornix, or on the cornea. They are usually composed of a polymeric vehicle containing the drug and are mainly used for topical therapy.
Ophthalmic inserts have been, and continue to be, in fashion in research and development laboratories, which is testified by abundant literature [81, 82]. The insert is probably the oldest ophthalmic formulation. Historically, the first solid medication precursors of the present insoluble inserts, were described in the 19th century. They consisted of squares of dry filter paper, previously impregnated with drug solutions (e.g., atropine sulfate, pilocarpine hydrochloride) [83], small sections were cut and applied under the eyelid. However, although the British Pharmacopoeia 1948 described an atropine in gelatin “wafer”, and notwithstanding all the formulation possibilities as well as the modulation of biopharmaceutical properties that inserts permit, the insert market never took off. This was apparently caused by incompatibility between the product-insert and the user-patient, particularly in the elderly; difficulty of insertion by the patient and foreign-body sensation. Besides the initial discomfort upon administration, other potential disadvantages arising from their solid state are, possible movement around the eye, occasional inadvertent loss during sleep or while rubbing the eyes, interference with vision and difficult placement (and removal for insoluble devices) [84]. Most of the ongoing research is therefore dedicated to improving ocular retention and to ensure an easy placement, while reducing the foreign body sensation in the eye. Two products, AlzaOcusert® [85] and Merck Lacrisert® [86], have been marketed, although Ocusert is no longer sold. Ocusert was an insoluble delicate sandwich technology filled with sufficient pilocarpine for 1 week’s use, whereas Lacrisert is a soluble minirod of hydroxypropyl cellulose, nonmedicated and dissolving within 24 h to treat dry-eye syndromes [86]. Other inserts are more like implants to be placed in the eye tissues by surgery and are not within the present scope of this Chapter.
Ophthalmic inserts are generally classified according to their solubility behavior and their possible biodegradability.
Soluble Inserts
Soluble inserts are the most frequently investigated class of ophthalmic inserts. Their main advantage relies on their complete solubility compared with their insoluble counterparts, so that they do not need to be removed from the eye after deposition. The major problems of these soluble inserts are the rapid penetration of the lacrimal fluid into the device, the blurred vision caused by the solubilization of insert components and the glassy constitution of the insert increasing the risk of expulsion. They are usually divided into two categories according to their polymer composition. The first type is based on natural polymers whereas the second is derived from synthetic or semisynthetic polymers.
Natural polymers. Natural polymers include collagen, which was the first ophthalmic insert excipient described in the literature. Inserts containing collagen were first developed by Fyodorov [87, 88] as corneal bandages following surgical operations and eye disease. Later, collagen shields as drug carriers were suggested by Bloomfield et al. [89]. As described for contact lenses, the therapeutic agents are generally absorbed by soaking the collagen shield in a solution containing the drug and, once placed in the eye, the drug is gradually released from the interstices between the collagen molecules, as the collagen dissolves. Accordingly, the residence time of drugs [90] such as antibacterials, [91, 92] anti-inflammatory agents [93, 94] antivirals [95] or combination drugs [96] was increased when compared to traditional eye drops. However, as observed for contact lenses, most drugs are released quite rapidly by a diffusion process, whereas dissolution requires a much longer time.
Solid Precorneal Inserts (Collagen Shields). Collagen shields were first introduced by Fyodorov in 1984 for use as a bandage contact lens following radial keratotomy and photorefractive surgery [97]. Collagen shields are manufactured from porcine scleral tissue and commercially available (Bio-Cor, Bausch & Lomb) with three dissolution times of 12, 24, and 72 h, depending on the level of collagen cross-linking induced during the manufacture process. Bloomfield et al. [89] were the first to suggest that collagen might provide a suitable carrier for sustained ocular drug delivery. They showed that wafer shaped collagen inserts impregnated with gentamicin produced higher levels of drug in the tear film and tissue in the rabbit eye compared to drops, ointment, or subconjunctival injection. They appeared useful as a delivery system for anti-infective agents and might possibly be of interest for some other drugs. Hydrophilic drugs are entrapped within the collagen matrix when the dry shield is soaked in aqueous solution of the drug whereas water-insoluble drugs are incorporated into the shield during the manufacturing process. When compared with intensive topical treatment, collagen shields have been found superior with regard to the delivery of different antibiotics and antifungal agents in the rabbit model [91, 98, 99]. In experimental bacterial keratitis in animal models, the enhanced drug delivery ability of collagen shield was translated to enhanced bacterial eradication [92, 100–102]. Improved results were reported also for the delivery of anti-inflammatory agents by collagen shields [93, 94, 103].
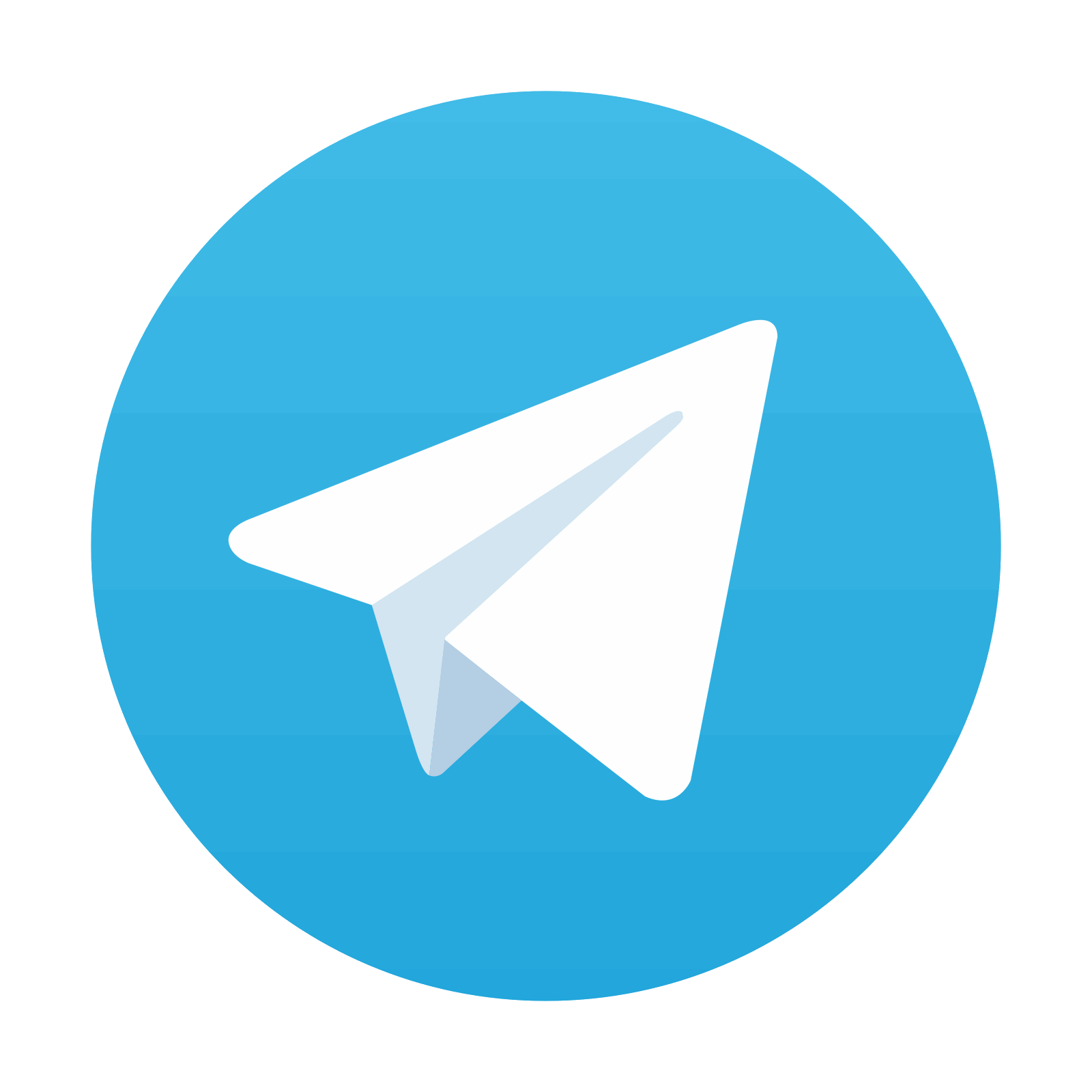
Stay updated, free articles. Join our Telegram channel

Full access? Get Clinical Tree
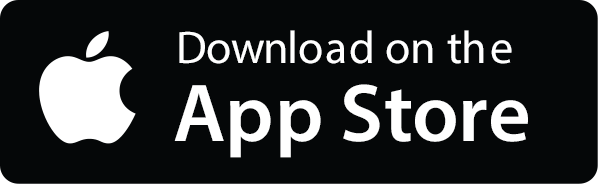
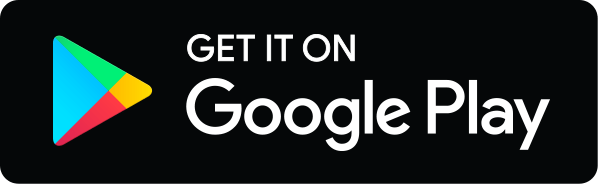