Function
Purpose
Examples
Binding
Fixation
Fractured bone fixation
Adhesion
Adhesion of tissue pieces
Closure
Suturing
Tissue approximation and vascular and intestinal anastomosis
Covering
Wound cover
Sealing
Topical hemostasis and air leakage
Occlusion
Vascular embolization
Separation
Isolation
Organ protection
Contact inhibition
Adhesion prevention
Scaffold
Cellular proliferation
Tissue and organ regeneration
Tissue guide
Nerve reunion
Capsulation
Controlled drug delivery system
Sustained drug release
Both synthetic and natural biodegradable polymers have been used as surgical materials, and these polymers degrade in the body through hydrolysis or enzymes, respectively. Synthetic polymers are mainly homopolymers or copolymers of poly(α-hydroxyacid)s. Natural polymers include proteins, such as collagen, gelatin, albumin and fibrin, and polysaccharides, such as hyaluronic acid, chitosan and chitin. Alternatively, water soluble polymers, such as carboxymethyl cellulose (i.e. natural polymer) and poly(ethylene glycol) (PEG) (i.e. synthetic polymer), have also been used that are not degradable but are absorbable in the body.
This chapter covers polymeric medical devices that are essential for surgical operation focusing in particular on bioabsorbable devices. Since some of these devices such as bone fixatives and drug delivery systems are covered in details in other chapters, bioabsorbable polymers used as sealants/adhesives/hemostatic agents, anti-adhesive barriers, and sutures are discussed. It is beyond the scope of this chapter to comprehensively cover all the research in this rapidly expanding area. Instead, the focus is on commercially available products and some recent research.
Sealants/Adhesives/Hemostatic Agents Against Bleeding and Other Types of Leakage
Surgical adhesives/sealants have been widely used in surgery to prevent air leaks (from holes in diseased soft tissues such as lung), liquid leaks (including hemostasis, as for oozing), and as adhesives (to bond two separate tissues, but very rarely). They have many advantages over traditional techniques (i.e. sutures and staples) such as reducing both operative time and physical load on patients. Sealants/adhesives are often liquids and cure when applied to form a firm gel. Ideally, they should be rapidly curable even in the presence of water, as pliable as soft tissues when cured, and bioabsorbable in the body. Three main types are available for these materials; naturally-derived (such as fibrin glue), semi-synthetic (such as naturally-derived polymer—aldehyde based), and synthetic (such as cyanoacrylates and PEG).
Requirements for these surgical sealants/adhesives are listed in Table 8.2 [1]. As they are applied to the human body, they must be safe, nontoxic, and free from risk of infectious transmission. They should be bioabsorbable and not persist long as a foreign body. The sealants (i.e. solutions that are applied and form a gel) as well as their degradation products must be safe. In addition, they must not hinder the natural healing process. Commercial products of these materials and recent research in surgical sealants/adhesives/hemostatic agents are discussed in this section. This is a fast growing area as can be seen from various recent reviews [2–5], and we will not cover all the products and research studies. Several commercially available products are listed in Table 8.3.
Table 8.2
Requirements for these surgical sealants/adhesives
1. In situ curable from the liquid state through polymerization, chemical cross-linking, or solvent evaporation |
---|
2. Rapidly curable under wet physiological conditions |
3. Strongly bondable to tissue |
4. Nontoxic agents and degradation products |
5. As tough and pliable as natural tissues |
6. Bioabsorbable |
Table 8.3
Commercially-available sealants, adhesives, and hemostatic agents
Main composition | Manufacturer | Trade name | Introduced/Approved year | Degradation/Absorption time | |
---|---|---|---|---|---|
Sealants/Adhesives | Fibrinogen and thrombin from human pooled plasma | Baxter | Tisseel, Artiss | 1998 | 2 weeks |
Johnson & Johnson | Evicel | 2006 | 2 weeks | ||
Aventis Behring | Beriplast | 1980s | 2 weeks | ||
PEG-NHS and trilysine | Confluent surgical | DuraSeal, DuraSeal Xact | 2003 | 4–8 weeks | |
PEG-NHS and PEG-SH | Baxter | CoSeal | 2001 | 4 weeks | |
PEGa, triethanolamine and eosin Y | Ethicon | AdvaSeal | 1998 | 1–2 months | |
Human serum albumin and PEG-NHS | BARD | Progel | 2010 | <1 month | |
Bovine serum albumin and polyaldehyde | Tenaxis Medical | ArterX vascular sealant | 2013 | >12–24 monthsb | |
Gelatin, resorcinol, formaldehyde and glutaraldehyde | Cardial | GRF glue | 1979 | >24 months | |
Bovine albumin and glutaraldehyde | CryoLife | BioGlue | 1999 | <24 months | |
Octyl-2-cyanoacrylate | Colure Medical | Dermabondc | 1998 | – | |
n-Butyl-2-cyanoacrylate | B.Braun | Histoacrylc | 1968 | – | |
COVIDIEN | Indermilc | 1996 | – | ||
Blend of n-butyl and 2-octyl cyanoacrylates | Advanced Medical Solutions | LiquiBandc | 1999 | – | |
(PVA)-(AAc)-(AAc-NHS) and PGLA | Tissuemed | TissuePatch3, TissuePatch | 2007 | <12 weeksd | |
Hemostatic Agents (Stand-alone) | Gelatin | Pfizer | Gelfoam | 1945 | 4 weeks |
Oxidized cellulose | Ethicon | Surgicel | 1942 | 7–14 days | |
Collagen | Bard | Abiten | 1970 | 8 weeks | |
Chitosan | HemCon Medical | HemCone, ChitoFlexe | 2002, 2007 | – | |
(Combination) | Fibrinogen, thrombin and collagen fleece | Nycomed Arzneimittel | TachoComb | 1990 | 16–20 weeks |
Thrombin and gelatin particles | Baxter | FloSeal | 1999 | 6–8 weeks | |
PEG, trilysine and oxidized cellulose backing | COVIDIEN | Veriset | N/Af | N/Af |
Naturally-Derived Sealants/Adhesives/Hemostatic Agents
Fibrin Glue
Fibrin glue is based on the natural clotting process, as shown in Fig. 8.1. It consists of two component systems; one contains human fibrinogen, Factor XIII, fibronectin, and fibrinolysis inhibitor, while the other contains human thrombin and calcium chloride (Table 8.4). When two solutions are mixed, thrombin promotes the conversion of fibrinogen to soluble fibrin molecules, as well as activates factor XIII. Fibrin molecules subsequently assemble into a fibrin clot and are stabilised by the activated factor XIII. Aprotinin is a basic pancreatic trypsin inhibitor which is added to commercial fibrin glues to yield delayed fibrinolysis of clots. The fibrin clot is degraded by physiologic fibrinolysis over a two-week period [6]. The concentration of fibrinogen determines the strength of the fibrin clot, and the amount of thrombin controls the rate of clot network formation. For application, two solutions are set in a dural-chamber delivery system with or without a spraying device. A fibrin clot is formed within 30 s when the components are applied at the site of injury. The process of preparation requires constant warming and stirring, which takes around 20–30 min.
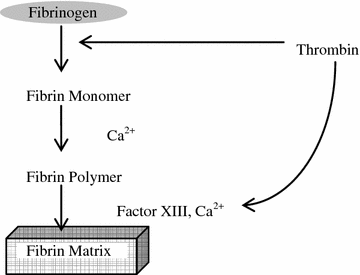
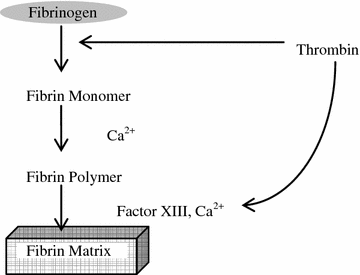
Fig. 8.1
Fibrin clot formation from fibrinogen
Table 8.4
Compositions of fibrin glues
Components | Concentration | Origin |
---|---|---|
Fibrinogen (clottable) | 80–120 g/l | Human plasma |
Factor XIII (activity) | 10–30 IU/ml | Human plasma or human placenta |
Fibronectin (antigen) | 5–20 g/l | Human plasma |
Thrombin (activity) | 300–600 NIH-U/ml | Human plasma or bovine plasma |
Aprotinin | 3,000 KIU/ml | Bovine lung |
Calcium chloride | 40–60 mM | Inorganic |
Fibrin glue has many advantages such as acceptable biocompatibility, biodegradability, injectability, and in situ-producible scaffolds for tissue regeneration. Successful clinical application of fibrin glue has been demonstrated in many surgical fields (Table 8.5) [7–9]. Cardiovascular surgery is the most common among them, and its major purpose is hemostasis and suture hole sealing. Fibrin glue is also highly effective as a tissue sealant in cases of diffuse, low-pressure bleeding such as coronary vein bleeding, right-ventricle/pulmonary-artery conduits, and right-ventricular patches.
Table 8.5
Clinical applications of fibrin glue
Cardiovascular surgery | Hemostasis of bleeding from mediastinum and small blood vessels, reinforcement of suture line, sealing of vascular grafts and anastomoses |
Thoracic surgery | Sealing of air leaks from raw lung surface, and pulmonary and bronchial staple lines |
Gynecologic surgery | Anastomosis of fallopian tube, adhesion of peritoneum |
Plastic surgery | Closure of skin wound, attachment of skin grafts, fixation of tissue flaps |
Orthopaedic surgery | Fixation of bone fragments, hemostasis during total knee arthroplasty |
Urologic surgery | Anastomosis of urinary tube and adhesion of kidney, pelvis renalis, and ureter |
On the contrary, some clinical applications have shown no effects of fibrin glue such as for reducing drain output or in facilitating early functional recovery in total knee arthroplasty [10] and seroma formation after breast surgery [11]. There are several disadvantages, such as the risk of viral infection due to human- and animal blood-derived products, low strength, and rapid loss of strength [12]. It also requires long preparation time and operator skill, and is quite expensive, at approximately $100–150/ml of fibrinogen.
Many technical efforts have been made to improve its low adhesive strength, especially in situations like sealing of needle holes. Methods of application include a separate dripping method using two separate syringes, a simultaneous dripping method using a syringe applicator, and a spray method using a spray applicator. Although the spray method exhibits the strongest sealing effect due to homogeneous application resulting in complete mixing of the two components [13–15], adhesive strength is still not adequate for control of local bleeding in the clinical setting. The rub-and-spray method consists of rubbing the fibrinogen solution onto the needle holes with a finger and then spraying the two solutions using an application nozzle. This technique has been experimentally and clinically found to be effective in cardiovascular surgery [16, 17].
For application in pulmonary surgeries, surgeons have often found that fibrin sealant does not effectively seal air leakages. To overcome this problem, the application of fibrin glue in combination with a polyglycolide (PGA) sheet (Neoveil, Gunze Corp, Kyoto, Japan) has been developed and shown to have clinical effectiveness [18–21]. The PGA sheet is a soft non-woven fabric, which is flexible, easily shaped to reconstruct complex shapes, and highly compatible with fibrin glue. It is degraded by hydrolysis and bioabsorbed in about 15 weeks. The PGA sheet retains fibrin glue that otherwise would run down from the rough site of application. The combination of PGA fabric and fibrin glue has been shown to improve adhesive strength and resistance to air/water leakage. This technique has been expanded to other applications including neurosurgery and gastroenterological surgery [22–27].
Fibrin Glue Sheet
TachoComb® (CSL Behring, PA, USA) is a ready-to-use hemostatic sheet, consisting of equine collagen fleece with surface coating of fibrin agents (human fibrinogen, bovine thrombin, and bovine aprotinin). Further development of TachoComb® is TachoSil®, and their compositions are shown in Table 8.6. The products do not require any preparation or conditioning, and can be immediately applied directly to traumatized tissues, in contrast to the liquid application of fibrin glue. When the coating of collagen fleece comes in contact with fluids (i.e. normal saline, body fluid, or a bleeding surface), the components dissolve and diffuse into the cavities and begin to react. The collagen fleece helps to tamponade the wound and keeps the coagulation components in the region of bleeding. It requires 3–5 min of pressing till the area is sealed. It is mechanically stable and can be used for the treatment of diffuse bleeding of the thoracic wall. The adhesive strength is much higher than that of fibrin glue, since dried fibrinogen and thrombin will form a highly concentrated gel when a small amount of surface water is used from the site of application.
Table 8.6
Active compositions of TachoComb® and TachoSil® (/cm2 sheet)
TachoComb | TachoSil |
---|---|
Fibrinogen (human, 5.5 mg) | Fibrinogen (human, 5.5 mg) |
Thrombin (bovine, 1.38 IU) | Thrombin (human, 2.0 IU) |
Aprotinin (bovine, 128 KIU) | |
Collagen fleece (equine, 1.65 mg) | Collagen fleece (equine, 2.1 mg) |
The application of fibrin glue sheet was reviewed for 408 patients with hemorrhagic risk factors or operations associated with an expected increase in bleeding [28]. The operations were performed on various organs, such as the liver, vascular system, heart, spleen, thorax, and kidney, and the results supported the efficacy and safety of TachoSil® as a hemostatic agent. Other recent clinical studies have demonstrated efficacies of Tachosil® in hepatobiliary and pancreatic surgeries [29–31] and lymph node dissection [32]. Tachosil® Fibrin glue sheet has also been used as a dural substitute, and a clinical study of 288 patients revealed no superficial or deep wound infections or aseptic meningitis [33].
Gelatin—Thrombin Hemostasis
FloSeal® (Baxter Healthcare Corporation, Fremont, CA, USA) consists of cross-linked gelatin granules and human thrombin (Thrombin-JMI®, a sterile freeze-dried powder, Jones Pharma, Inc., Bristol, VA, USA). Bovine-derived gelatin is cross-linked with glutaraldehyde and ground to 500–600 µm sized particle. Prior to use, the thrombin is reconstituted with saline and added to the gelatin matrix component in the operating suite.
When applied to a bleeding site, the gelatin granules swell by 10–20 % within 10 min, reducing blood flow and providing tamponade. It can conform to irregular wound shapes. The high concentration of thrombin reacts with fibrinogen and forms a reinforced clot around the gelatin matrix. Unreacted granules can be removed with gentle irrigation or suction. The granules in the clot are bioabsorbed by the body in 6–8 weeks. Unlike fibrin glue, this agent only works in the presence of blood [34, 35]. FloSeal® has been widely used in various surgical operations [36], and its application in neurosurgery has also been reviewed [37].
Other Types of Natural Physical Hemostatic Agents
Various types of biodegradable hemostatic agents are currently available. These include gelatin foams, collagen microfibrillar, fibrillar/knitted oxidized cellulose, and chitosan sponges (Table 8.3). These hemostatic agents have often been applied to aid to cease hemorrhage mechanically when surgeons compress the bleeding points. They can absorb fluid up to several times of their own weight and are useful in the situation of heavier bleeding. Despite their clinical successes as hemostatic agents, there are some disadvantages, such as weak adherence to wet tissues, complications from the expanded materials pressing nerves in surrounding tissues against hard tissue, confusions of the presence of passive hemostatic agents in subsequent diagnostic images with a tumor or abscess, and prolonged residual products causing foreign body reaction that lead to granuloma formation [38].
Semi-synthetic Sealants/Adhesives/Hemostatic Agents
Gelatin-Based Glue
Gelatin-resorcinol-formaldehyde (GRF glue, Cardial, Techinopole, Sainte-Etienne, France) is composed of two types of solutions, GR solution (gelatin and resorcinol) and F-activator (formaldehyde and glutaraldehyde). Formaldehyde and glutaraldehyde react not only with gelatin and resorcinol, but may also react with amino groups of tissues, creating bonding between the resulting gel and the tissue. The proposed chemical structures of these components and their reaction scheme are shown in Fig. 8.2.
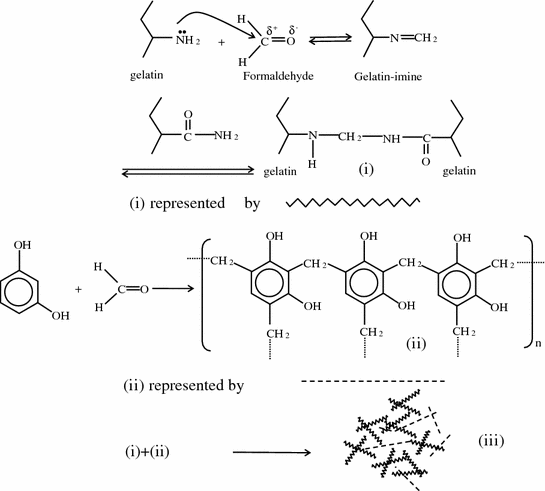
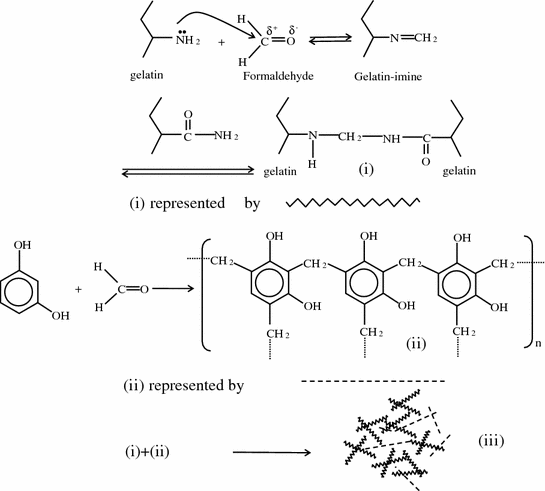
Fig. 8.2
Reaction scheme for gelatin-resorcinol-formaldehyde (GRF) glue
Since the introduction of GRF in 1979 for acute aortic dissection [39], it has been widely used except in the United States. In acute type A aortic dissection, the survival of patients depends on immediate diagnosis and emergent surgical intervention. Without surgery, the mortality rate is 38 % in the first day and up to 90 % after 2 weeks. The clinical use of GRF is problematic due to continued controversy concerning the possible carcinogenicity and mutagenicity of formaldehyde. It has been reported that long-term exposure of formaldehyde in embalming practice and in the funeral industry is significantly associated with increased risk for mortality from myeloid leukemia [40].
Efforts have been made to eliminate formaldehyde from the composition of GRF and to replace it with glutaraldehyde alone or glyoxal. However, these attempts were unsuccessful, as its bonding was reduced and affected the efficacy of the product, though greater durability was observed in vivo. By reducing the formaldehyde concentration and mixing with glutaraldehyde, high initial strength and increased durability were achieved [41]. Conflicting data are found in the literature on the effect of GRF glue in aortic surgery. Long-term stability of the dissected, GRF-treated aorta has been reported, whereas other studies found late adverse events such as distal embolization [42–44].
Albumin-Based Glue
In BioGlue® (CryoLife, Inc., Kennesaw, GA, USA), stoichiometrically equivalent doses of bovine serum albumin (45 % solution) and glutaraldehyde (10 % solution) are mixed in a custom cartridge delivery system [45]. It has been used in cardiac, vascular, pulmonary, and soft tissue repairs. The major applications include sealing around suture lines and staples in large blood vessels such as the aorta and femoral arteries. In comparison to fibrin glue, BioGlue® has high adhesive strength to tissues and is much stiffer [46], as well as has longer degradation time (Table 8.3).
In 2007, Zehr reported detailed techniques of application for the use of BioGlue® in a variety of cardiovascular surgical cases and found that BioGlue® was safe and effective when used properly [47]. In a clinical study involving 151 patients undergoing cardiac and vascular repair procedures, anastomotic bleeding was significantly reduced in the Bioglue® group (18.8 %, n = 76) compared with the control group (42.9 %, p < 0.001, n = 75). The use of pledgets was reduced in the BioGlue® group (26.2 %) compared with the control group (35.9 %, p = 0.047) [48]. In application in thoracic surgery, a randomized, controlled trial of the effectiveness of BioGlue® was carried out in 52 patients being treated for alveolar air leaks [49]. Surgical treatment with BioGlue® exhibited statistically significant effectiveness in all categories compared to the control group (surgical treatment only). Despite its success in clinical studies, the toxicity of unreacted and leached glutaraldehyde and the dense postoperative gel structure have been the concerns and have limited the application of BioGlue®. Fürst et al. investigated the release of glutaraldehyde from BioGlue® and its toxicity in vitro and in vivo [50]. It was found that 100–200 μg/mL of glutaraldehyde was released from 1 mL of gelled BioGlue® into 5 mL of saline, and the supernatant exhibited cytotoxicity in cultured human embryonic fibroblasts (MRC5) and mouse myoblasts (C1C12).
Progel® (Neomend, Inc., Irvine, CA, USA) is based on human serum albumin and PEG disuccinimidyl succinate as a cross-linker. This product is approved by FDA for intraoperative use during pulmonary resection. A review of preclinical and clinical studies demonstrated the efficacy and safety of this product [51]. Another recently approved albumin-based product is ArterX® vascular sealant (Tenaxis Medical, Mountain View, CA, USA), which is composed of bovine serum albumin and polyaldehyde, for use as a sealant around anastomotic sites of synthetic arterial bypass grafts and patches. A randomized prospective multicentre trail, involving 331 anastomotic sites in 217 patients, was undertaken comparing ArterX® vascular sealant with Gelfoam® Plus (i.e. control group), and showed no difference in safety [52]. The operation time was significantly less in the ArterX® vascular sealant group compared with the control group.
Lysine-Based Glue
TissuGlu® (Cohera Medical, Pittsburgh, PA, USA) is a lysine-derived urethane adhesive which cures in the presence of moisture. This is applied to the abdominal wall prior to closure of the abdominoplasty flap to reduce wound drainage and seroma formation. The safety and efficacy of TissuGlu® have been demonstrated in preclinical and clinical studies [53, 54].
Oxidized Cellulose-Based Hemostatic Agent
VerisetTM (COVIDIEN, Inc., Mansfield, MA, USA) is a topical hemostatic agent which is a combination of an oxidized cellulose backing and self-adhesive hydrogel consisting of PEG and trilysine. This ready-to-use hemostatic patch has been clinically compared with TachoSil® in the management of diffuse bleeding after hepatic surgery (n = 50) [55]. They found that the median time to hemostasis in the VerisetTM group was faster than that of the control group (1 vs. 3 min, p < 0.001).
Synthetic Sealants/Adhesives
Cyanoacrylates
Cyanoacrylates are monomers containing esters of cyanoacrylic acid. Various homologues of cyanoacrylate adhesive have been studied including methyl, ethyl, isobutyl, isohexyl, and octyl cyanoaclylates. These monomers rapidly polymerize when in contact with water or hydroxyl groups on the actual surface being glued [56]. These create strong and flexible films as sealants to bond apposed wound skin edges. Use of these topical skin adhesives is faster and less painful than that of suturing. The skin edges are held in apposition and cyanoacrylate is applied in layers along the entire length of the wound. It is recommended by the manufacturers that a total of three layers of the glue is applied. Spontaneous release of heat occurs as the polymer forms. The agent remains adherent to the skin for approximately 7–10 days while the wound heals, and comes off as the superficial layers of skin exfoliate. Cyanoacrylates have been used mainly for external surfaces as tissue adhesives for surgical and traumatic wound repair, due to their toxicity.
The shorter chain esters may be more toxic either directly or through their degradation products, which are cyanacrylates and formaldehyde. The degradation products accumulate in tissues and produce acute and chronic inflammation. In contrast, longer alkyl chains (e.g. 10 carbons) are less toxic. In addition, the shorter chain esters such as butyl cyanoacrylate have been used only for small low-tension lacerations and incisions due to its poor tensile strength and brittleness, whereas octyl cyanoacrylate yields stronger and more flexible adhesives [57]. In an animal model, various commercially available cyanoacrylate products have been compared for wound bursting strengths immediately after closure and 1 and 2 days after [58]. Dermabond® (Colure Medical Corporation, Raleigh, NC, USA), which consists of octyl 2-cyanoacrylate, was significantly stronger and more flexible than all the cyanoacrylate-based adhesives tested at all time points of measurement.
Surface application of Dermabond® has been shown to be very successful. A randomized, control trial showed that the wounds closed by Dermabond® had no noticeable differences in subjective cosmetic results and was associated with complications at 3 months postoperatively, compared with wounds closed using monofilament sutures. Adhesives also had the advantage of much faster operative time than traditional suturing. A randomized controlled trail of Liquiband® Surgical S (Advanced Medical Solutions, Devon, UK), which is a blend of n-butyl and 2-octyl cyanoacrylates, and sutures for closure of laparoscopic wounds showed closure with Liquiband® was significantly faster by mean of 2 min [59]. In addition, the use of skin adhesives has shown to reduce surgical site infections from some specific bacterial agents [60, 61].
PEG-Based Sealants
PEG-based sealants are flexible and firmly adherent even on wet tissues. These hydrogels also have the ability to prevent adhesions. DuraSealTM (Confluent Surgical Inc., Waltham, MA, USA) is one such product developed for watertight closure after dural suturing. Two solutions, PEG-ester and trilysine amine, are mixed together and sprayed on the site of treatment (Fig. 8.3). They rapidly react via electrophilic nucleation reaction and form an elastic hydrogel. A multicentre, prospective randomized trial involving 237 patients was performed to evaluate the safety of DuraSealTM as a dural sealant in cranial surgery [62]. DuraSealTM was found to be similarly safe as commonly used dural sealing techniques, and demonstrated faster preparation and application times than other techniques.
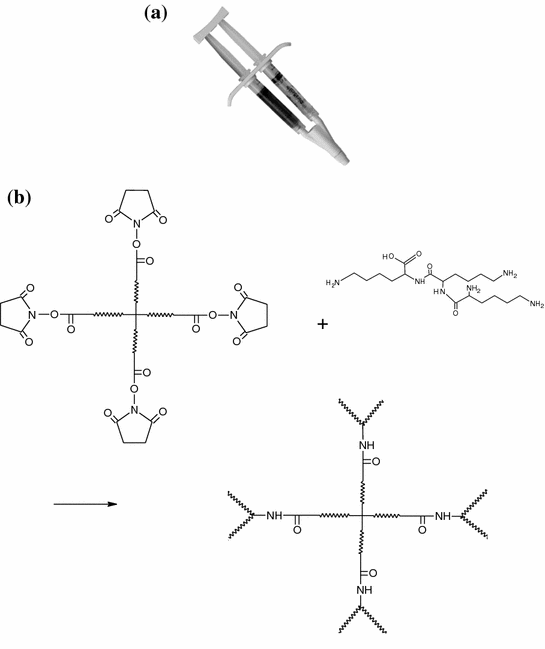
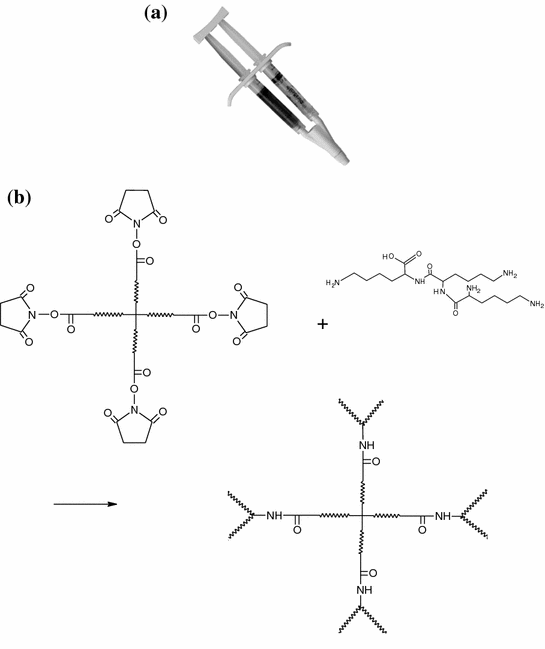
Fig. 8.3
a DuraSealTM applicator and b reaction scheme for DuraSealTM
CoSeal® (Baxter, Fremont, CA, USA) is another PEG-based hydrogel that is used in vascular reconstructions to achieve adjunctive hemostasis by mechanically sealing the region of leakage. Tetrafunctional succinimdylglutaryl PEG macromer (PEG-NHS) reacts with a thiol PEG macromer (PEG-SH) and protein to form a dimer, which leads to the formation of cross-linked polymers (Fig. 8.4). It polymerizes in ~5 s. The hydrogel swells approximately 400 % over 24 h. A randomized, controlled trial was performed to evaluate the efficacy of CoSeal® for anastomotic suture hole bleeding and compare it to Gelfoam® (Upjohn, Kalamazoo, Mich)/Thrombin [63]. One hundred forty-eight patients scheduled for implantation of polytetrafluoroethylene (PTFE) grafts were randomly treated with either CoSeal® (n = 74) or control (n = 74). The efficacy of CoSeal® was equivalent to that of Gelfoam®/Thrombin. Clinical studies have also demonstrated the efficacy of CoSeal® in the anastomotic closure of aortic procedures [64] and preventing prolonged air leaks after lung resection [65].
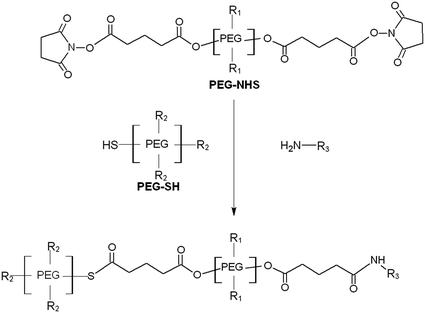
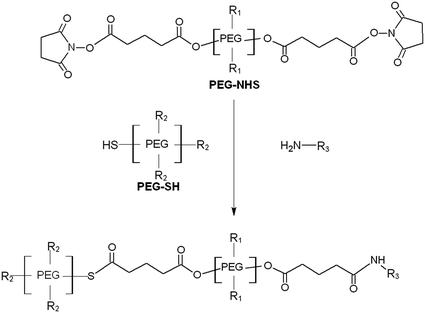
Fig. 8.4
Reaction scheme for CoSeal®
AdvaSealTM (Ethicon Inc, Johnson & Johnson Medical KK, NJ, USA) is based on photo-polymerization of two aqueous solutions consisting of PEG and oligotriethylene carbonate with acrylate ester end caps, in the presence of triethanolamine and eosin Y as a photo-initiator, using visible light from a xenon arc lamp. It has been clinically used for sealing of pulmonary air leakage in Europe [66, 67].
Synthetic Adhesive Sheet
TissuePatch3TM (Tissuemed Ltd., Leeds, UK) is a synthetic, bioabsorbable self-adhesive film that has been developed to prevent air and liquid leaks in surgery. It consists of adhesive terpolymer (61 %) and poly(lactide-co-glycolide) (33.5 %). The terpolymer contains three components: polyvinylpyrrolidone (PVP), acrylic acid (AAc), and acrylic acid/N-hydroxysuccinimide (AAc-NHS), which confer the properties of water solubility, electrostatic interaction, and covalent bonding potential, respectively [68]. The chemical structure of terpolymer is shown in Fig. 8.5. No preparatory step is required and it adheres within 30 s of application. The efficacy of TissuePatch3 was clinically evaluated for sealing of air leakage after lung surgery [69]. It was found that 80 % of patients (12 of 15 patients) became air leakage-free at the end of the surgical procedure. No device-related adverse events were observed. The new generation product, TissuePatchTM, has been investigated for alveolar air leak prevention in vitro [70], as well as in the management of chyle leak in major neck surgery [71].
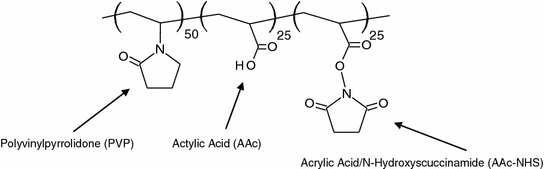
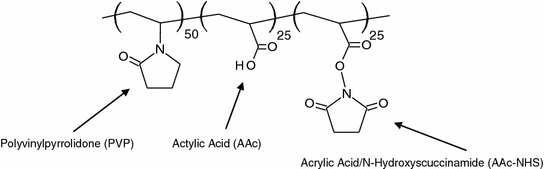
Fig. 8.5
Terpolymer of TissuePatchTM
Recent Developments
Albumin-Based Sealant
Taguchi et al. developed biological glues combining an organic acid derivative and proteins such as collagen, gelatin, and human serum albumin. The active ester groups of the organic acid derivative react with amino groups of not only proteins of glue but also applied tissue surfaces. Citric acid derivative was used as a cross-linking agent. Citric acid, which is the source of citric acid derivative, is metabolized in the tricarboxylic acid cycle in the mitochondria. Although gels consisting of citric acid derivative and collagen or human serum albumin have exhibited sufficient bonding strength in vitro and insignificant toxicity, both required comparatively long periods of time to reach a certain bonding strength. To reduce the bonding time, tartaric acid derivative (TAD) was used as a cross-linking agent and the bonding strengths of glues (TAD-A) developed by reacting different concentrations of TAD (0.1, 0.3 and 0.5 mmol) and human serum albumin (44 w/w%) were compared with those of fibrin glue and GRF [72]. The bonding strengths of glues increased with the increasing TAD concentration, and even the lowest TAD glue exhibited significantly higher bonding strength than fibrin glue. The bonding strengths of glues with 0.3 and 0.5 mmol TAD were significantly higher than that of GRF. When the glue was implanted subcutaneously in mice to investigate the inflammatory reaction in surrounding tissues, the glue with low TAD concentrations (0.1 and 0.2 mmol) exhibited very mild inflammation, whereas the 0.3 and 0.5 mmol TAD gels were associated with moderate inflammation, possibly due to the local acidification by n-hydroxysuccinimide, which separates from carboxyl groups after the cross-linking reaction.
Fibrin-Based Sealant
Fibrinogen has been rapidly photo-cross-linked in the presence of ruthenium as the trisbipyridyl complex and the oxidant ammonium or sodium persulfate by light to yield high-molecular-weight products that have strong in vitro adhesive properties [73]. A mixture of fibrinogen (150 mg/mL), 2 mM RuII(bpy)3Cl2, and 20 mM sodium persulfate was prepared, and applied immediately to wounds, followed by illumination with a 600 W tungsten-halide light source at 150 mm for 20 s [73]. The sealant was well tolerated and persisted up to 8 weeks but completely dissolved by 18 weeks with minimal inflammatory response. The photo-cross-linked fibrinogen sealed skin incisions in rats, and as an arterial hemostat in pig, it stopped bleeding within 20 s of application.
Gelatin-Based Sealant
Different cross-linking types of gelatin-based glues have been developed. One of such is the sealant consisting of gelatin and glutaraldehyde as a cross-linking agent [74]. The glue consisting of 23 wt% gelatin and 0.12 wt% glutaraldehyde exhibited more than 90 % degradation in 7 days when implanted subcutaneously in rats, and the bonding strength was much higher than that of fibrin glue. The gelatin glue was applied with or without rubbing in with fingers to seal the needle hole on the PTFE vascular graft (Gore-Tex®). The water pressure in the graft was increased and the burst pressure was measured when water leakage occurred. The burst pressure of the gelatin glue applied with rubbing was found to be 400 mmHg, while that of fibrin glue was 200 mmHg. The high viscosity of gelatin solution also prevents leakage through suture line needle-holes, which is often a problem with the low viscosity of serum albumin in Bioglue® [75].
Various gelatins, such as porcine type A (~300 g Bloom), bovine type B (~225 g Bloom) and cold water fish gelatin (~60 kDa), were photo-cross-linked and evaluated in vitro and in vivo for a pulmonary sealant in an animal study [76]. As previously described for photo-cross-linking of fibrinogen, gelatin was also rapidly cross-linked using blue light, a ruthenium catalyst, and a persulfate oxidant. The adhesive strength of porcine gelatin was similar to that of photo-polymerized fibrinogen and approximately 5 times higher than that of the commercial fibrin glue. The maximum adhesion strength was reached within 30 s, but with a light-emitting diode (LED) lamp high adhesive strength was achieved instantaneously. The photo-polymerized porcine gelatin swelled substantially with increase in weight of 240 % within 24 h. To reduce swelling, the gelatin was derivatized to increase content of phenolic (Tyr-like) residues using Bolton-Hunter reagent (N-succinimidyl-3-[4-hydroxy]propionate), and thus to increase its cross-linking density after polymerization. The resultant gel increased the elastic modulus (stiffness) approximately 5-fold, and reduced its swelling to less than 10 % at 24 h. In an animal study, the photo-polymerized gelatin effectively sealed a wound in lung tissue from blood and air leakage, and was not cytotoxic and did not produce any inflammatory response.
PEG-Based Sealant
PEG/dextran aldehyde was developed as biocompatible tissue adhesive [77]. It consisted of a relatively short-chain PEG polymer (linear PEG of 2 kDa or 8-arm PEG of 10 kDa) containing amine-terminated groups, and dextran containing aldehyde groups. Aldehyde to amine ratios of 1, 3, and 10 were examined for linear and star-PEG-based constructs. The adhesive forces of these polymers were determined by the number of aldehyde groups within the gel and reached saturation at a dextran aldehyde content of ~20 wt%. Cytotoxicity and proliferation of 3T3 rat fibroblast tests revealed that PEG/dextran was significantly less cytotoxic than octyl cyanoacrylate. The higher-molecular-weight dextran aldehyde exhibited lower cytotoxicity as well as higher cell proliferation [77].
A topical hemostatic agent comprised of polycarbonate of dihydroxyacetone (pDHA) and methoxypoly(ethylene glycol) (MPEG) was studied by Henderson et al. [78]. DHA is the fifth metabolite of glucose as it is metabolized to pyruvic acid. Polymerized sequence of dihydroxyacetone was PEGylated (MPEG-pDHA). The edge of rat liver was cut and treated with MPEG-pDHA (50 mg), normal saline (0.5 ml), or InstatTM (50 mg). The MPEG-pDHA had significantly decreased bleeding time (97 s) and total blood loss (1.35 g) compared to those with normal saline (464 s and 3.83 g) and InstatTM (165 s and 2.04 g).
Chitosan-Based Sealant
Chitosan, which is an inexpensive and widely available material, has been investigated for its usefulness as a hemostatic material because it is cationic and antimicrobial, but it may not be effective for severe wounds. Photo-cross-linkable chitosan molecules which contain both lactose moieties and photoactive azide groups (Az-CH-LA) have been developed. The Az-CH-LA hydrogel was combined with a chitosan sponge (PCM-S), and its hemostatic efficacy was compared with that of TachoComb® in a heparinized rat model [79]. Even under heparinized condition, the bleeding time and blood loss of the PCM-S group were significantly lower than those of the TachoComb® group. In another study, the photo-curable chitosan was evaluated as an adhesive for peripheral nerve anastomosis to restore continuity to severed peripheral nerves [80]. Photo-chemical tissue bonding has been utilized for the adhesion of chitosan film [81]. In this technique, a solution of rose bengal is applied between two tissue edges and the area is irradiated with a green light to cross-link collagen fibers with minimal heat production. The resulting adhesive strength of the film to intestine was 15 ± 2 kPa, and the average temperature of the adhesive increased from 26 °C to only 32 °C during laser exposure [81].
Dowling et al. [82] developed a chitosan-based amphiphilic biopolymer that exhibits clotting ability in the presence of blood by self-assembly that is readily reversible by introducing a sugar-based supramolecule. First, chitosan was hydrophobically modified by attaching a small number of hydrophobic tails to the backbone (hm-chitosan). The hydrophobes from the chitosan anchor into the hydrophobic interior of blood cell membranes, connecting them into a gel network which can halt the flow of blood. The reaction is not affected by heparinization of blood. To reverse the gel into a sol, α-cyclodextrin, a supramolecular compound with an inner hydrophobic pocket, is added. The strong affinity of α-cyclodextrin for hydrophobes causes these polymer moieties to unhook from the cell membranes and bind to α-cyclodextrin. The initial blood gel based on 0.25 wt% hm-chitosan exhibits an elastic, gel-like response, whereas addition of 3 wt% α-cyclodextrin results in a viscous liquid response. Preliminary tests with small and large animal-injury models demonstrated its increased efficacy at achieving hemostasis.
Polysaccharide-Based Sealant
Adhesives made of aldehyded polysaccharides and ε-poly(l-lysine), which are anti-bacterial additives for medical use and food, have been investigated for use in sutureless amniotic membrane transplantation [83]. The adhesive gel is prepared using a syringe-like container with two cylinders, one with 2 ml of 14 % aldehyded dextran (Mw 75 kDa) solution and the other with 2 mL of 7 % ε-poly(l-lysine) (Mw 4 kDa) solution containing 2 % acetic anhydride. The acetic anhydride concentration added to the ε-poly(l-lysine) solution determines the gelation time. The gel formed by cross-linking via Schiff base in about 30 s at 37 °C and degrades within 4 days in vivo. The bonding strength of the glue was 4 times that of commercial fibrin glue and almost no cytotoxicity was observed. The efficacy of the glue in fixing amniotic membrane without suturing for ocular surface reconstruction was evaluated in an animal model. The sutureless amniotic membrane placement using the adhesive was safely and successfully performed onto a rabbit sclera surface.
Natural Adhesives/Sealants
Mussel-derived adhesive protein is known to be the most powerful natural adhesive, and has both flexibility and elasticity [84, 85]. In 1981, Waite and Tanzer discovered 3,4-dihydroxy-l-phenylalanine (DOPA) as a key component for the wet-resistant adhesion of mussel adhesive proteins [86]. Non-toxic and non-immunogenic effects of mussel proteins have been reported [87–89]. More interestingly, it maintains its adhesion in wet environment, and adheres to virtually any types of synthetic and natural surfaces [90]. However, expensive extraction, which requires 10,000 mussels to obtain 1 g of one type of adhesive proteins, and unsuccessful large-scale production limit its practical applications [84].
DOPA has been used to increase the adhesion strength of PEG-based sealant [91]. Adhesive consisting of star PEG amine and linear dextran aldehyde was developed, but after swelling of the gel the strength of adhesion to soft tissues significantly decreased. Incorporation of DOPA into the PEG: dextran sealant was investigated to determine enhancement of post-swelling sealant performance. Homogeneous solutions of DOPA and lyophilized dextran aldehyde were dialyzed against water followed by freeze-drying. The PEG: dextran doped with 3 mM DOPA aldehyde swelled 50 % less, had 3-fold greater stiffness and 50 % greater functional adhesive strength than the neat hydrogel. Increasing the DOPA concentration to 11 mM decreased the swelling and mitigated loss of properties with hydration, but reduced the initial functional adhesive strength, material modulus, and biocompatibility.
Dermal exudate from Notaden bennetti frogs, another natural adhesive, was investigated for medical use [92]. The exudate rapidly forms a protein-based pressure-sensitive adhesive that functions well in wet environments. The glue implanted subcutaneously in mice was bioabsorbed and exhibited no long-term adverse effects. The non-protein components of the frog glue caused local and transient necrosis, although they did not contribute to the adhesive properties of the glue. The glue could bond severed cartilage tissue both ex vivo and in vivo.
The hemostatic characteristics of keratin derived from human hair were investigated using a rabbit model of lethal liver injury [93]. Keratin was extracted from human hair, and the protein solution was concentrated to 20 wt% using a rotary evaporation system prior to surgery and exposed to air overnight to form a cross-linked hydrogel. The keratin gel exhibited adhesion to the tissue, and when deposited onto the bleeding surface of liver it was sufficiently adhesive and absorbed blood and became more adherent within a few minutes. The keratin gel demonstrated efficacy in arresting hemorrhage and improving survivability similar to those of two commercial products, QuickClot® and HemCon®. The gel was non-toxic and non-immunogenic.
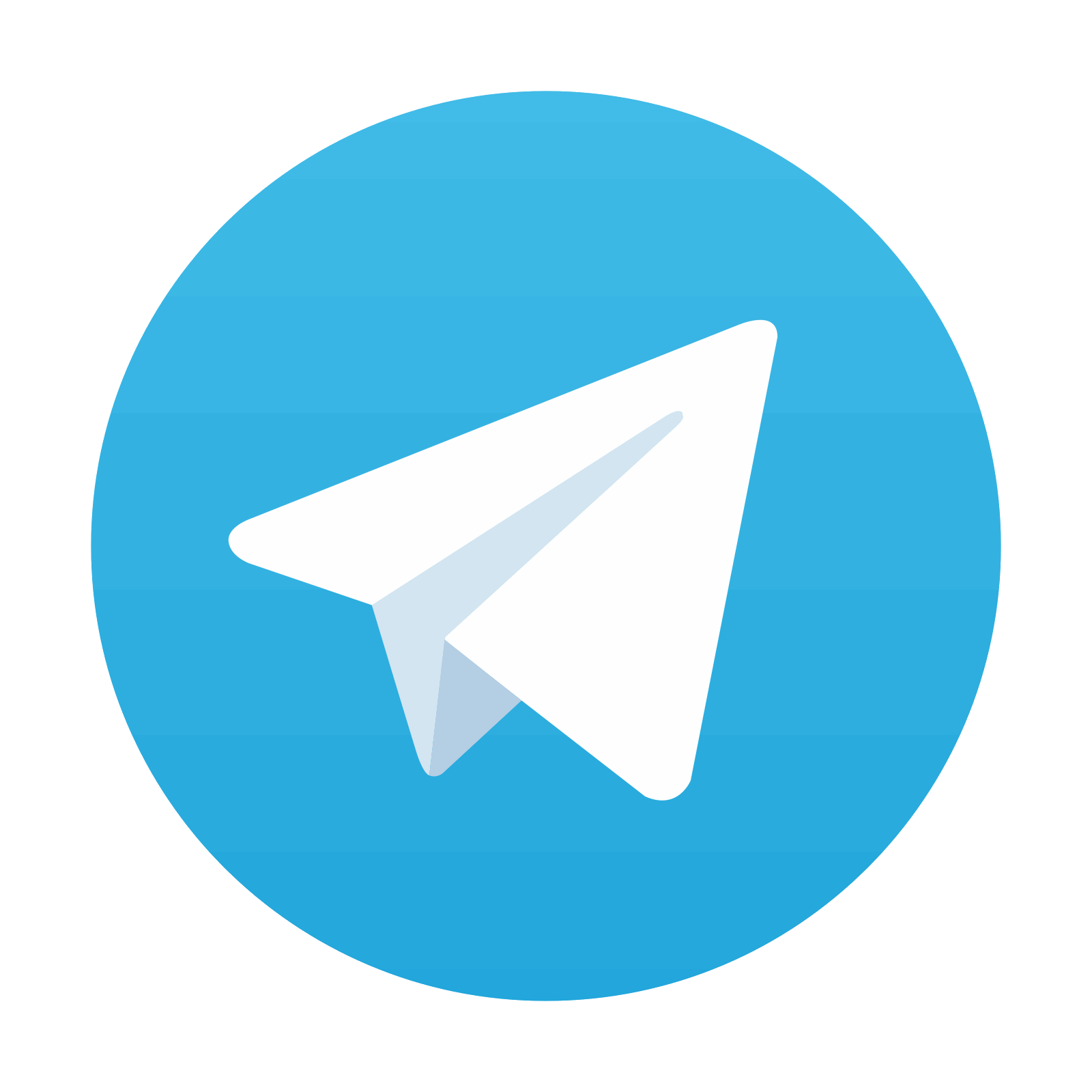
Stay updated, free articles. Join our Telegram channel

Full access? Get Clinical Tree
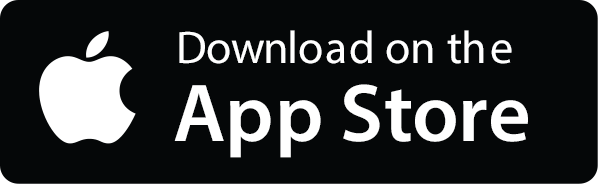
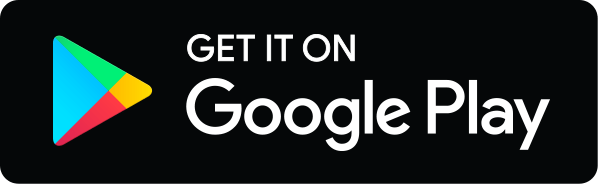