7.2.2 Crystal growth from solution
Crystals may grow from solution but this requires that the initial concentration be supersaturated (i.e. above its solubility).
Study question 7.1 Why must the solution be supersaturated for crystallisation to occur?
This may take some time and so the process is often accelerated by adding a seed crystal or other nucleation point (as noted above), reducing the volume of solvent (via evaporation), changing the pH, adding a miscible anti-solvent (i.e. a solvent in which the solute has poor solubility) or reducing the temperature (which usually reduces the solubility of the solute).
The process of crystal growth from a supersaturated solution can be considered to be the reverse of the process of dissolution discussed earlier, involving diffusion of solute molecules to the crystal surface and condensation, and so can be described in similar terms to the Noyes–Whitney equation introduced in Chapter 5. The driving force is still a concentration difference, now between saturation (St) and supersaturation (Ss):
The remaining terms are the same as defined earlier. In practice crystals often dissolve faster than they grow and so Equation (7.1) is not always found to be valid. An alternative model considers a growth constant (kg) and an ‘order’ of crystal growth, n (Mullin, 2001):
(7.2)
In cases where multiple crystal forms may exist, selection of crystallisation solvents may be used preferentially to select one form over another. Other solutes may be added to the solution to change the shape of the crystal produced (see below).
- Crystals form when molecules condense from the liquid state or from a supersaturated solution.
- Crystal growth proceeds via deposition of molecules on to a nucleus.
- The nucleus can be formed in situ by alignment of molecules (primary nucleation) or seed crystals can be added (secondary nucleation).
7.3 Crystal structure
When molecules condense into a solid phase they will usually (but not always – see Chapter 8) orient themselves so that they are in structural alignment, forming a crystal lattice. Assuming that the arrangement is such that the enthalpy and volume are minimised, a perfect crystal will have formed. It is not possible to arrange the constituent molecules of a perfect crystal in any other way that would result in a lower enthalpy or volume.
The structure of a crystal lattice may be defined by considering the smallest structural arrangement of its constituent molecules that repeats in three dimensions: the unit cell. Surprisingly, given the myriad molecules in nature, there are only a limited number of unit cell arrangements. Bravais (1850) first worked out the lattice arrangements and showed there are only seven basic unit cells (Table 7.1). The unit cells are shown diagrammatically in Figure 7.2; the molecules in the unit cells are represented as point sources (the black spheres) and in the basic unit cells appear only at the external corners (called primitive centring).
Table 7.1 Axis and angle rules for the seven Bravais unit cells.
Name | Axis rules | Angle rules |
Triclinic | ![]() | ![]() |
Monoclinic | ![]() | ![]() |
Orthorhombic | ![]() | ![]() |
Tetragonal | ![]() | ![]() |
Hexagonal | ![]() | ![]() |
Rhombohedral | a=b=c | ![]() |
Cubic | a=b=c | ![]() |
In addition to primitive centring, molecules may also be located at the centre of the unit cell (body centred), at the centre of each face of the unit cell (face centred) or at the centre of a pair of opposing faces of the unit cell (base centred – three base centred arrangements are possible). This means there are 42 (7×6) possible Bravais lattices, although only 14 are unique (the remainder can be shown to be mathematically equivalent). The 14 unique Bravais lattices are shown in Figure 7.2. Pharmaceutical compounds are typically triclinic, monoclinic or orthorhombic.
The unit cell arrangement then repeats in three dimensions to produce the macroscopic crystal. The gross exterior appearance of a crystal is called its habit. The USP lists six basic habits (acicular (needle-shaped), blade, columnar, equant (or isometric, including cubic and spherical), plate and tabular; Figure 7.3), although other terms are frequently encountered (such as hexagonal, octahedral, prismatic and pyramidal). The names are simply descriptive terms for the macroscopic shape of the crystal and are not necessarily related to the geometry of the unit cell. Crystal habit can significantly affect particle and powder bulk properties, discussed in Chapters 10 and 11.
- Crystal structures are described in terms of the minimum arrangement of molecules that repeats in a three-dimensional pattern – the unit cell.
- Pharmaceutical crystals are usually monoclinic, triclinic or orthorhombic.
- The macroscopic shape of the crystal is called the habit.
7.4 Polymorphism
When a compound can crystallise to more than one unit cell (i.e. the molecules in the unit cells are arranged in different patterns) it is said to be polymorphic (from the Greek, ‘many forms’). The form with the highest melting temperature is called the stable polymorphic form and all other forms are metastable.
The formation of polymorphs can be considered using the same thermodynamic argument adopted earlier. When the rate of cooling is slow the molecules have sufficient time to organise themselves in the most efficient (enthalpy-minimised) arrangement possible and the stable polymorph is formed. If the rate of cooling is sufficiently fast that the stable polymorph does not have time to form, the material will remain in the liquid phase below the melting temperature of the stable form; this is termed a supercooled liquid. At some lower temperature the molecules will align themselves in an alternate, but less efficiently packed, crystal form and there will be a discontinuity in enthalpy or volume as a metastable crystal is formed (Figure 7.4). Thus, the metastable form is characterised as having greater enthalpy and volume and a lower melting temperature than the stable form.
Study question 7.2 If other metastable polymorphs exist how would Figure 7.4 be extended?
7.4.1 Thermodynamics of polymorphism
So far it has been assumed that the same form is the stable polymorph under all conditions of temperature (and pressure, but temperature is the more important parameter in the context of preformulation). If so, the compound is said to exhibit monotropic polymorphism. It is possible, however, that with a change in temperature a different form becomes the stable form. In this case the compound exhibits enantiotropic polymorphism.
The concepts of monotropic and enantiotropic polymorphism can be explained with reference to enthalpy and free energy curves plotted against temperature (again, pressure is assumed to be constant, but analogous situations occur with changes in pressure – see Lohani and Grant, 2006). Figure 7.5 shows the case for a monotropic pair of polymorphs. At absolute zero entropy is zero and so enthalpy and free energy are equal. As temperature increases, enthalpy increases and free energy decreases (because entropy will increase) in accordance with the familiar thermodynamic relationship
(7.3)
Figure 7.5 Schematic representation of the change in free energy with temperature for two monotropic polymorphs.
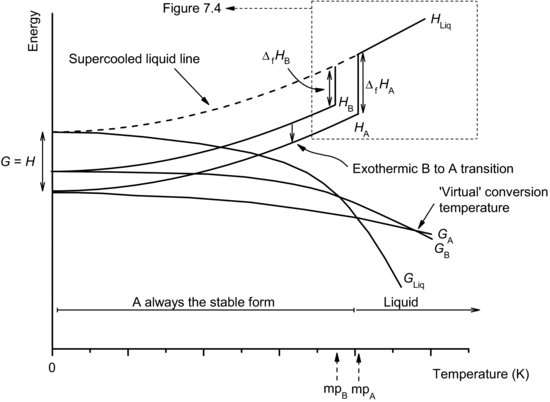
Note that although (as assumed in constructing Figure 7.1) the change in enthalpy or free energy might be assumed to be linear over small temperature changes, over large temperature ranges nonlinear behaviour is seen. This is one of the reasons for exercising caution when using Arrhenius extrapolations during stress testing.
The form with the lowest free energy at any particular temperature is the most stable. In the case of the monotropically related pair represented in Figure 7.5 the most stable solid form is A, at all temperatures up to the melting temperature (the point at which the liquid curve falls below the solid curve). Form B exists as a solid form because it has a lower free energy than the liquid at all temperatures below its melting temperature, but is always metastable with respect to form A. The free energy curves for forms A and B eventually reach a point of intersection (at which point form B would become the most stable form), but not until after intersection with the liquid curve (by which point both forms have melted). If form B were to convert to form A, which from a thermodynamic perspective is inevitable, but from a kinetic perspective may take some considerable time, there would be an exothermic change in enthalpy, as form B always has greater enthalpy than form A. Many drugs exhibit monotropic polymorphism, including, for example, most of the barbiturates, the sulphonamides, spironolactone, premafloxacin, furosemide and indomethacin.
The case for an enantiotropically related pair is shown in Figure 7.6. The changes in enthalpy and free energy are analogous to those described above for a monotropically related pair, with the exception that the point of intersection of the free energy curves of the two solid forms occurs before the intersection of either with the liquid curve. Since the form with the lowest free energy is the most stable, before the temperature of intersection form A is stable and form B metastable; after the temperature of intersection the situation is reversed, form B being stable and form A being metastable. Correspondingly, form A melts at a lower temperature than form B. As the temperature of intersection is exceeded, form A will (from a thermodynamic perspective) convert to form B, now with an endothermic change in enthalpy (as form B always has a higher enthalpy than form A).
Figure 7.6 Schematic representation of the change in free energy with temperature for two enantiotropic polymorphs.
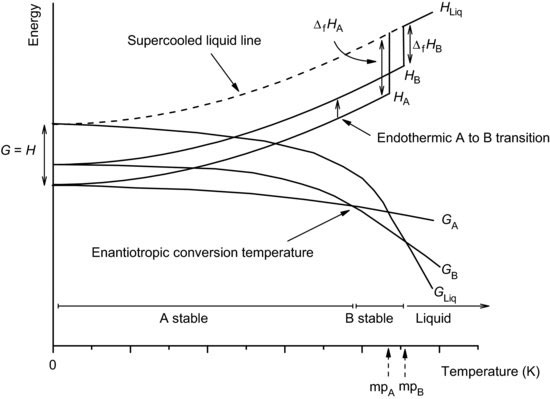
This leads to the heat of transition rule for determining whether a pair of polymorphs are monotropically or enantiotropically related:
- An exothermic enthalpy of transition is seen for monotropic pairs.
- An endothermic enthalpy of transition is seen for enantiotropic pairs.
Other rules for determining whether a monotropic or an enantiotropic relationship exits are:
Examples of drugs that exhibit enantiotropic polymorphism include neotame, albendazole and sulphathiazole.
- If the same compound can form crystals with different unit cells it is said to be polymorphic.
- The polymorph with the highest melting temperature is the stable form and all others are metastable.
- With time, accelerated with temperature and/or plasticisers, metastable forms will transform to the stable form.
- If one form is stable at all temperatures (and pressures) the compound exhibits monotropic polymorphism.
- If different forms are stable at different temperatures (and pressures) the compound exhibits enantiotropic polymorphism.
7.4.2 Physicochemical properties of polymorphs
Figure 7.7 (top) shows a schematic representation of two polymorphic forms of a drug. Although nothing of the chemical structure or nature of the drug is specified it is apparent from the diagram that the two polymorphs probably exhibit different physical properties; one that is evident is density, with form I appearing to have a greater number of molecules per unit volume. Since the molecules are more closely packed, form I is also likely to have a greater lattice energy (strength between molecules) and hence a higher melting point than form II.
Figure 7.7 Schematic representation of polymorphic forms of a drug (top left and right) as well as a monohydrate or solvate (bottom left) and a co-crystal (bottom right).
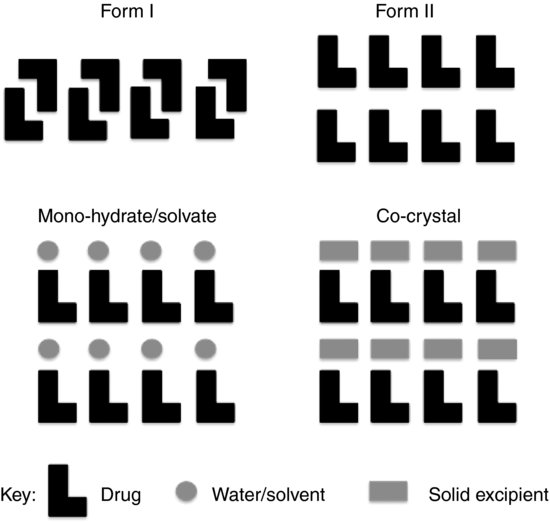
Study question 7.3 What other properties might change with physicochemical form?
A defining feature of polymorphs is thermodynamic stability (in this case referring to the physical form itself rather than any chemical decomposition or interaction of its constituent molecules). Since only the stable form can be considered to be at a position of thermodynamic equilibrium, over time the metastable forms will eventually convert to the stable form. It is tempting therefore to consider formulating only the stable polymorph of a drug substance, since this ensures that there can be no change in the polymorph upon storage.
If the stable polymorph shows acceptable bioavailability then it is of course the best option for development (patent issues aside). However, sometimes the stable form has poor processing characteristics (the stable form I of acetaminophen, for instance, has poor compressibility, unlike the metastable form II). Moreover, with the increasing number of poorly soluble drug substances being developed, formulation of a metastable form can be an attractive option for increasing bioavailablity, the classic example being provided by chloramphenicol palmitate (Figure 7.8), where the β form shows much greater bioavailability than the α form (and so there are strict limits in the pharmacopoeia monograph on the amount of the α form permissible in the drug product). Selection of the most appropriate polymorph for development is therefore often a compromise between increased bioavailability and decreased physical stability.
Figure 7.8 Blood plasma concentrations versus time for two polymorphs of chloramphenicol palmitate (redrawn from Aguiar et al. (1967), with permission from John Wiley & Sons, Inc.).
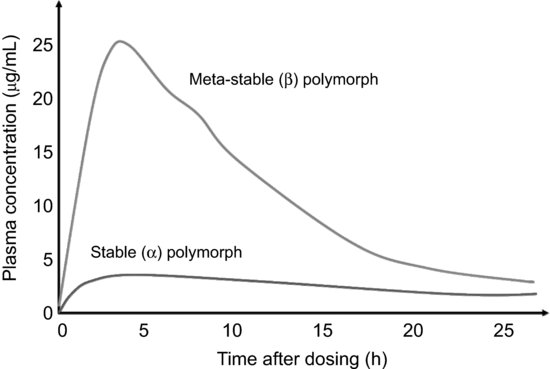
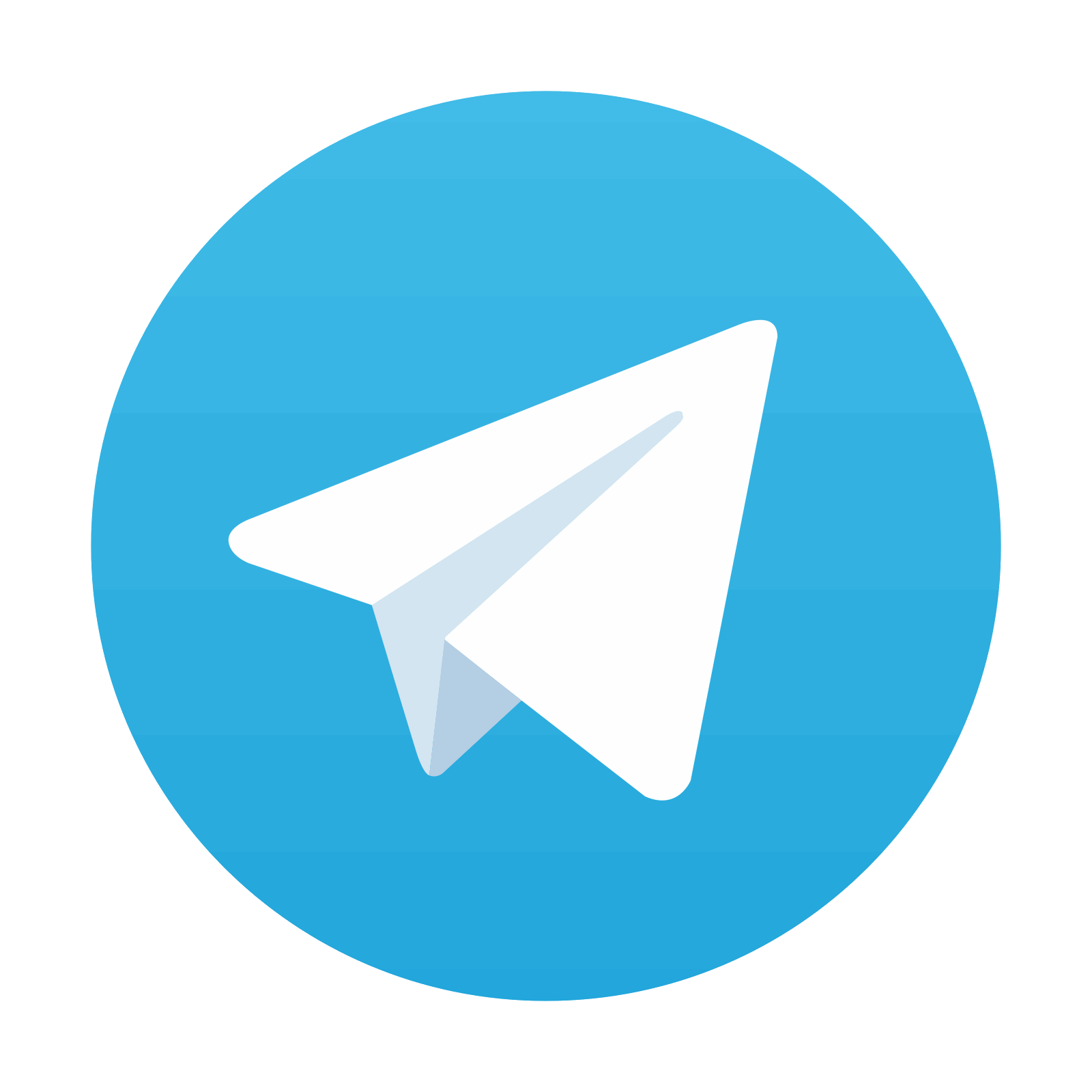