Phosgene and Toxic Gases
Melissa L. Givens
NAME AND DESCRIPTION OF AGENT
Toxic gases, also known as choking agents, are the forerunners of modern chemical warfare. Chlorine gas was first deployed during World War I. On April 22, 1915, the Germans released 150 tons of chlorine gas along the battlefront in Ypres, Belgium, creating multiple casualties who taxed medical resources. The novel use of toxic gas created widespread fear of this new chemical weaponry. Subsequently, battle stress precipitated by this horrific threat compounded the treatment challenges faced by medical professionals. Phosgene was also used during World War I, both alone and mixed with chlorine gas. Phosgene was the likely culprit in nearly 80% of poison gas deaths that occurred during the war (1,2). Use of phosgene on the battlefield was estimated to have resulted in 311,000 man-days lost to hospitalization during the war, the equivalent of 852 man-years (3). This degree of hospitalization only underscores the potential for toxic gas exposure to strain hospital resources in the event of a terrorist attack.
Toxic gas use is not isolated to World War I. The use of phosgene by Egyptian bombers has been reported in attacks against the Yemeni royalist forces in the Yemeni civil war during the 1980s (4). More recently, on April 20, 1995, the Aum Shrinrikyo (“Supreme Truth”) cult, the same organization linked to the sarin release in the Tokyo subway, was implicated in the release of a phosgene-type gas in a train station in Yokohama, resulting in the hospitalization of over 300 people.
Chlorine and phosgene are produced in large quantities worldwide and used extensively in multiple industries. Chlorine is commonly used in cleaning products, in water purification, and as an intermediate in the manufacturing of plastics and synthetics. Chlorine is the most common cause of accidental industrial and household inhalational injury in the United States, and the release of toxic amounts of chlorine has occurred worldwide more than 200 times since the early 20th century (5, 6, 7).
Phosgene is also used extensively in industry as a chemical precursor in the production of dyes, pesticides, plastics, polyurethane, isocyanates, and pharmaceuticals. Phosgene is also formed by combustion of chlorinated fluorocarbons, which are found in refrigeration units, and toxicity has been reported in refrigeration workers who were welding refrigeration conduits and welders using chlorinated solvents (8,9). Over 1 million tons of phosgene are used yearly in the United States (10). An estimated 5 billion pounds are produced worldwide (11). Identification of phosgene can be confusing because there are many other nomenclatures including: carbonyl chloride, carbon oxychloride, carbonic acid chloride, D-Stoff, and green cross (2,12). The military designation for phosgene is CG. The widespread availability of phosgene and chlorine makes them attractive agents of terror. Both agents are mass-produced and are stored and transported in large-volume containers that could have devastating effects if vaporized. The ease of attainment and distribution, coupled with the significant strain on medical resources that accompanies injury with these agents makes toxic gases potential terrorist agents of warfare. The psychological harm that can be inflicted with a chemical attack is only a bonus feature in terms of terrorist potential.
There are other agents that also may produce toxic injury to the airways such as smoke and obscurants, including zinc oxide, phosphorous smokes, sulfur trioxide-chlorosulfonic acid, and perfluoroisobutylene (a combustion product of Teflon). These agents are less likely to be used in a direct chemical attack. They are very irritating to victims, causing them to evacuate the smoke cloud quickly, thus limiting exposure. Consequently, these agents are considered less desirable as offensive weapons. The discussion of such agents is beyond the scope of this chapter. This chapter will focus mainly on the acute and the delayed injury pattern associated with phosgene exposure. Chlorine will be discussed briefly to highlight the clinical differences that can occur when dealing with a patient exposed to a toxic gas.
THEORETICAL AND SCIENTIFIC BACKGROUND
Toxic gas injury is determined by the chemical properties of the toxic gas and the conditions in which the exposure occurs.
Phosgene is a gas at temperatures above 47°F (8°C) (13). At temperatures below the boiling point, phosgene is a liquid and has limited toxicity. Chlorine enters the gaseous state at F (-34°C) (13). It is often stored in a compressed state because of its low boiling point. The ambient heat, humidity, and air currents can all affect the properties of these gases and the clinical response generated by the exposure. A drop in ambient pressure may result in an increase of the toxin in the gaseous state. Strong wind currents can dissipate the gas to nontoxic levels and changes in wind patterns can result in the gas distributing in an unpredictable direction.
Phosgene is a gas at temperatures above 47°F (8°C) (13). At temperatures below the boiling point, phosgene is a liquid and has limited toxicity. Chlorine enters the gaseous state at F (-34°C) (13). It is often stored in a compressed state because of its low boiling point. The ambient heat, humidity, and air currents can all affect the properties of these gases and the clinical response generated by the exposure. A drop in ambient pressure may result in an increase of the toxin in the gaseous state. Strong wind currents can dissipate the gas to nontoxic levels and changes in wind patterns can result in the gas distributing in an unpredictable direction.
Once an individual is exposed to a toxic gas, the extent of injury is defined by the exposure characteristics. Exposure is defined by duration (t) and intensity or concentration (C) where Ct = concentration in mg/m3multiplied by time in minutes. Confounding variables that alter the concentration over time include respiratory rate, depth of respirations (minute ventilation), and even body position. Of note, all can be affected by the sympathetic discharge likely to occur in the event of a terrorist attack. Patients are likely to be breathing rapidly and deeply and may not think to protect their airway as a first line of defense. Also, one cannot forget to consider underlying conditions such as history of hyperreactive airways and tobacco use when trying to predict the clinical outcome of a toxic gas exposure. The presence of underlying disease can magnify the clinical response and can result in severe symptoms at even negligible concentration exposures.
The effects of toxic gases on the human airway depend on the chemical properties of the gas. The anatomic site of injury is related to the solubility of the gas in water. Gases that are more water-soluble, such as chlorine, primarily affect upper airways and central airways. Less soluble gases, like phosgene, penetrate deeper into lungs and affect peripheral airways and alveoli. The end result of this deep injury is damage to the capillary-alveolar wall and leakage of plasma into the alveoli.
Chlorine (Cl2), discovered in 1774, is a greenish-yellow gas, with an offensive, pungent odor that fortunately acts as a warning signal at concentrations below lethal exposures (14). Coughing occurs at 30 parts per million (ppm) and exposures of 40 to 60 ppm for more than 30 minutes may cause severe damage. Lethality has been reported at exposures of 500 ppm for 5 minutes (13). Once chlorine reaches the respiratory tract, it reacts with water to form hydrochloric and hypochlorous acids. Hypochlorous acid reacts with sulfhydryl groups of cysteine, and also causes enzyme inhibition (15). Additionally, chlorine hydrolysis generates free radicals capable of penetrating cell membranes that can result in cell injury and death. The formation of hydrochloric acid is most likely to occur in the moist areas of the eyes, mouth, and upper airways, thus chlorine is much more irritating when compared to phosgene, which undergoes little hydrolysis. However, the exact mechanisms of chlorine toxicity in the respiratory tract cannot entirely be attributed to this formation of hydrochloric acid, and pathogenesis is still controversial (14).
Phosgene (COCl2), which was first manufactured in 1812 by Sir Humphrey Davey in Great Britain, is a colorless, volatile gas that is heavier than air; it has an odor of freshly mown hay. Toxic exposure occurs below the odor threshold which is 0.4 ppm (16). This is important because victims may be exposed to dangerous levels without even realizing that they are in danger. Estimated LCt50 for phosgene is 500 to 800 ppm for a 2-minute exposure (2,17). Once phosgene is inspired, hydrolysis occurs upon contact with water resulting in the formation of carbon dioxide and hydrogen chloride.

This liberation of hydrogen chloride was once thought to be the mechanism of injury; however, the amount of hydrogen chloride released is miniscule and cannot account for the pulmonary damage incurred by phosgene exposure (18). The formation of hydrogen chloride may account for the small degree of mucous membrane irritation similar to that seen in chlorine exposure.
Phosgene also reacts with sulfhydryl, amine, and hydroxyl groups, which are cellular constituents of biological molecules, in a process known as acylation (19).

Acylation results in protein and lipoid denaturation, changes in membrane structure, and disruption of enzymes (2). Phosgene also disrupts the surfactant layer, thus impairing mechanical performance that depends on adequate surface tension for alveolar gas exchange (20). Phosgene can undergo heterolytic and/or homeolytic cleavage into a reactive carbamoyl monochloride radical. This may be responsible for its reactivity with lung tissue components (21).
Animal studies of phosgene show changes in energy metabolism. Cyclic adenosine triphosphate (cATP) and cyclic adenosine monophophate (cAMP) are decreased, which may result in inadequate energy to maintain fluid homeostasis within the lung (22,23). Decreases in oxygen uptake and depressed cellular glycolysis are also observed. Phosgene additionally causes disruption of the glutathione redox cycle (glutathione acts as a natural antioxidant), with subsequent increase in opportunities for oxidant injury by free radicals (24,25,26). The oxidant stress of phosgene is demonstrated by elevated antioxidant enzyme levels for days after acute exposure (24,25,26,25). Phosgene exposure has also been shown to cause lipid peroxidation and release of arachidonic acid metabolites to include sulfidopeptide leukotrienes TC4/LTD4/LTE4. These leukotrienes are of interest because they are the primary components of SRS-A. SRS-A is the slow reacting substance of anaphylaxis. Leukotrienes can be tied to vasoconstriction of bronchial smooth muscle, coronary vasoconstriction, and increased vascular permeability. LTB4 is a neutrophil chemotactic agent and is elevated in rats exposed to phosgene, and subsequent neutrophil migration is noted in conjunction with elevated levels of this leukotriene. The exact role of these mediators in phosgene-induced injury is still unclear, and there are conflicting animal studies in regards to how leukotrienes may
modulate or incite injury. The inflammatory process may be the cause of toxin-mediated injury or a response to the direct toxic effects (28,29,30,31).
modulate or incite injury. The inflammatory process may be the cause of toxin-mediated injury or a response to the direct toxic effects (28,29,30,31).
In extremely high concentrations (greater than 200 ppm), phosgene’s effects may no longer be isolated to the lungs. Phosgene may cross the blood air barrier in the lung and cause hemolysis and red blood cell hyperaggregation (32). This causes pulmonary sludging, which in turn may cause cor pulmonale and subsequent death (18).
SIGNS AND SYMPTOMS
Toxic gases can produce clinical signs and symptoms in several ways. If the gas is released in an enclosed space, the gas can displace oxygen and result in asphyxia from lack of oxygen. Toxic gases may also cause direct damage to the respiratory tract resulting in airway obstruction, interstitial damage, and alveolar-capillary damage, all with impaired oxygen exchange. Additionally, an allergic response that results in cellular damage or tissue swelling and possible systemic inflammatory damage may be initiated. Each of these sequelae of toxic gas exposure can affect how the patient presents clinically, and the clinician must be prepared to deal with a combination of clinical responses to toxic gas exposure. A comparison of chlorine and phosgene gas exposures is seen in Table 4-1 in the summary section of the chapter.
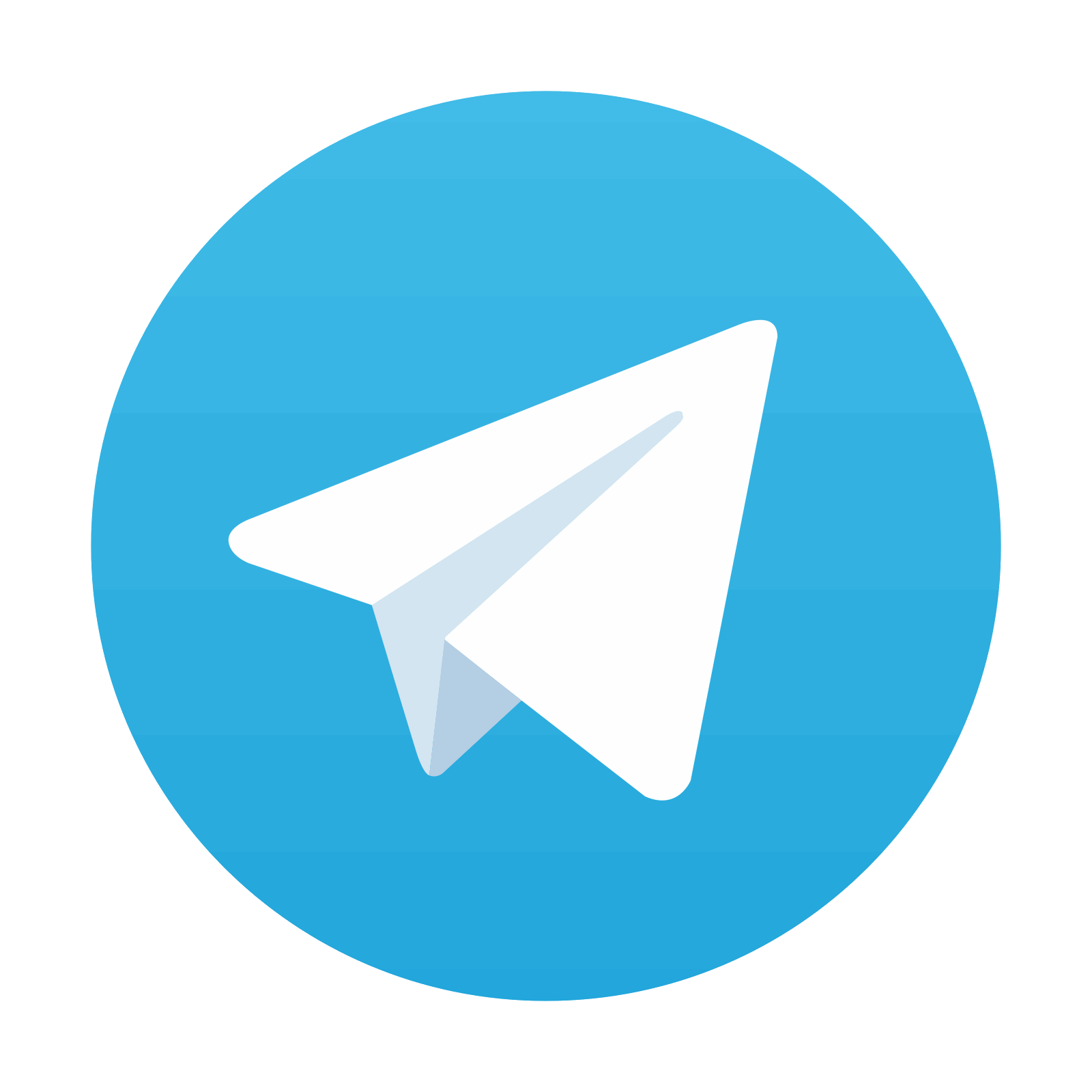
Stay updated, free articles. Join our Telegram channel

Full access? Get Clinical Tree
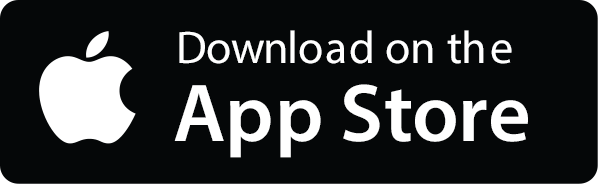
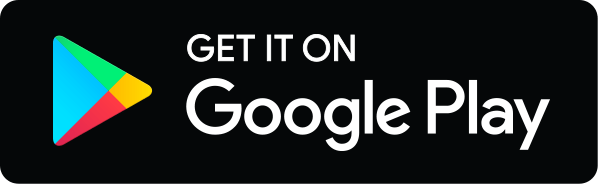