Fig. 2.1
Critical residues involved in TRPV1 function. TRPV1 topology highlighting residues implicated in the activation, sensitisation, desensitisation, gating properties or permeation of the channel
2.6.1 The N Terminus
Like other vanilloid type TRP proteins (Jin et al. 2006; McCleverty et al. 2006; Erler et al. 2004), TRPV1’s cytosolic N-terminus hosts an ankyrin repeat domain (ARD) that contains six ankyrin repeats (Fig. 2.1). The 33-amino acid sequence of an ankyrin repeat can be found in many proteins with various responsibilities including cytoskeletal integrity, cell-cycle regulation, inflammation and transcription (Mosavi et al. 2004) and is required for channel function (Hellwig et al. 2005). Within the TRPV family, the ARD enables self-association between TRPV6 subunits (Erler et al. 2004) and the oligomerisation required for TRPV4 biogenesis (Arniges et al. 2006). Studies specifically investigating the TRPV1 ARD have identified a multi-ligand binding site for CaM and ATP which partly regulates the Ca2+-dependent desensitisation discussed above (Koplas et al. 1997).
ATP binds to some transporters and receptors via nucleotide-binding sites known as ATP-binding cassettes (ABCs) containing the Walker A and B motifs and a signal sequence between their primary structures (Walker et al. 1982; Decottignies and Goffeau 1997; Hung et al. 1998). While TRPV1 does not possess the complete sequence, ATP was first proposed to interact with the N-terminus via a Walker B motif including D178. Mutation of D178 abolishes ATP-mediated potentiation of capsaicin-induced currents in TRPV1-expressing oocytes (Kwak et al. 2000), though Lishko’s model shows that this residue lies within a helix of the second ARD rather than the typical Walker B β strand (Lishko et al. 2007).
As previously mentioned, extracellular acidity and intracellular alkalinity stimulate TRPV1 independently and the latter is mediated via the N-terminus. The alkaline deprotonation-underlying activation by an increase in cytosolic pH occurs at H378 (Fig. 2.1), a histidine residue located between the ARD and the first transmembrane segment (Dhaka et al. 2009). Point mutation studies have also shown that R114 within the N-terminus (Fig. 2.1) is a structural necessity for the channel’s hydrophilic interaction with capsaicin and RTX (Jung et al. 2002).
In addition to binding sites, the N-terminus houses sites for post-transcriptional modifications. These sites include Y199 (or Y200 in humans; Fig. 2.1), the phosphorylation of which by Src kinase following nerve growth factor-induced activation of the tyrosine kinase A receptor promotes TRPV1 channel’s insertion into the cell membrane (Zhang et al. 2005). The N-terminus is also suitably equipped for mediating the regulation of Ca2+-dependent desensitisation; in addition to its above-mentioned CaM binding region it houses multiple consensus sites for PKA-mediated phosphorylation. Electrophysiological study of TRPV1 mutants has identified both S116 and T370 (Fig. 2.1) as major PKA phosphorylation sites required for reduction of TRPV1 desensitisation following capsaicin stimulation (Bhave et al. 2002; Mohapatra and Nau 2005). While phosphorylation at these sites reduces desensitisation, dephosphorylation at T144 and T370 (Fig. 2.1) by calcineurin promotes desensitisation (Jeske et al. 2006).
2.6.2 The C Terminus
TRPV1’s C-terminus is composed of the distal 155 residues of the molecule (Fig. 2.1). Among its key structural features is the TRP domain (E684-R721; Fig. 2.1), a sequence located near the channel gate that is responsible for the tetramerisation of TRPV1 monomers (Garcia-Sanz et al. 2004) and modulating the channel’s function. Functional TRPV chimaeras in which TRPV1’s TRP domains are replaced with cognate domains from TRPV2-4 require capsaicin and/or heat to exhibit voltage-dependent gating. This, coupled with further biophysical analysis supporting the TRP domain’s role in determining the activation energy of channel gating, supports the region’s role in coupling stimulus reception with channel gating (Garcia-Sanz et al. 2007). Further study has localised key residues that determine activation energy to the TRP box, a region in the TRP domain that is highly conserved between the TRPV, the melastatin type (TRPM) and the canonical type (TRPC) TRP subfamilies. Alanine substitution of I696, W697 and R701 within this six-residue segment significantly impairs voltage-, heat- and capsaicin-induced channel activity in a manner consistent with modification of downstream signalling and TRP box-dependent channel subunit interaction (Valente et al. 2008). Consistent with the C-terminus’ putative role in gating control, truncating the distal 31 or 72 amino acids of TRPV1 progressively reduces pH-, heat- and capsaicin-sensitivity, its thermal threshold and the onset, outward rectification and peak tail current amplitude of voltage-induced currents (Vlachova et al. 2003). Furthermore, TRP chimaeras coupling TRPV1 with the C-terminus of the cold-sensing TRPM8 molecule, or vice versa, exhibit the temperature sensitivity of the donor channel (Brauchi et al. 2006). This demonstrates that the C-terminus confers the channel’s thermosensing phenotype. Subsequent investigation established that deletion of residues 741–752 abolishes TRPV1’s heat-sensitivity and hence localised a major thermosensing region to the C-terminus (Brauchi et al. 2007). In experimenting with these chimaeric receptors, Brauchi noted the exchange of another important C-terminus feature; the modulation site of PIP2. PIP2 binding has been suggested to occur within residues 777–820 (Fig. 2.1) by Prescott and Julius (2003). However, homology modelling challenges the role of this region as a direct binding site and instead suggests that PIP2 makes contact with K694, K698 and K701 within the C-terminus (Fig. 2.1) (Brauchi et al. 2007). As discussed above, PIP2’s role in TRPV1 modulation remains unclear.
As with the proposed Walker B motif within the N-terminus, the C-terminus was proposed to contain the Walker A motif normally found within ABC transporters. Point mutation of a residue within this sequence at K735 prevents the sensitising effects of ATP in TRPV1-expressing oocytes (Kwak et al. 2000), suggesting the C-terminus possesses a nucleotide binding domain. Despite not possessing any previously defined CaM binding motifs, the C-terminus was also found to bind CaM within a 35-residue region (Numazaki et al. 2003). Point mutations within this region subsequently established the involvement of 5 residues in CaM binding (V769, R771, R785, K788 and R797). These findings were consistent with a model by which the C-terminus’ binding motif docks into CaM as a basic amphiphilic α-helix (Grycova et al. 2008). Additionally, E761 was found to be of structural significance in vanilloid binding alongside R114 within the N-terminus (Jung et al. 2002).
There are further functional similarities between the structural components of the C- and N-termini. Point mutations of PKA consensus sites revealed that mimicking phosphorylation via S774D and S820D mutations significantly reduces capsaicin-induced desensitisation, demonstrating the presence of phosphorylation sites on the C-terminus. However, raising cAMP levels with forskolin pre-treatment in the presence of these mutants decreased tachyphylaxis further still. As this was not observed with S116D and T370D mutations, N-terminus-based phosphorylation sites appear to play the major roles in PKA-mediated TRPV1 modulation (Mohapatra and Nau 2003). Conversely, TRPV1 phosphorylation by PKC occurs at the C- rather than the N-terminus. Point mutation studies have identified that S800 is a major phosphorylation site underlying PKC’s sensitising TRPV1 to capsaicin (Fig. 2.1) (Numazaki et al. 2002; Bhave et al. 2003), while S774 and S820 serve as minor PKC phosphorylation sites (Bhave et al. 2003). Furthermore, the ability of phorbol esters to activate TRPV1 in addition to stimulating PKC was identified and the potentiation of resulting currents by PKC was shown to be dependent on S704 (Fig. 2.1) (Bhave et al. 2003). The C-terminus hence hosts a variety of important structural features that regulate tetramerisation, gating properties, temperature sensitivity and channel sensitisation.
2.6.3 The Pore Domain
TRPV1’s pore domain is formed by S5, S6 and the adjoining pore loop (P-loop) between them (Fig. 2.1). S6 constitutes the inner pore helix while the P-loop serves as the channel’s selectivity filter. Subsequently, many key residues that determine the channel’s gating and permeability properties have been identified here. Employing the substituted cysteine accessibility method to scan S6 of rat TRPV1 elucidated the presence of two intracellular constrictions that block the ion conduction pathway. One, located at L681, prevents the ingress of large (~6 Å) molecules. The other, at Y671, prevents the passage of small ions (~2 Å) and comprises TRPV1’s activation gate in response to both capsaicin and heat (Fig. 2.1). Given the periodicity of an alpha helix, the accessibility of these residues was consistent with their positioning within the aqueous pore-facing side of a helical transmembrane domain (Salazar et al. 2009). This arrangement is consistent with the previous findings that T671 modulates Ca2+-permeation properties and is implicated in Ca2+-dependent desensitisation (Mohapatra et al. 2003). Beyond in addition to identifying pore constriction sites, point mutation studies have elucidated a range of residues with alternative functional relevance in the pore region. Following evidence that D646 neutralisation reduces divalent cation permeability, it has been suggested that this residue might form a ring of negative charges in order to construct a high affinity cation binding site near the channel’s extracellular entrance. Such an arrangement could serve to coordinate cation movement and hence modulate channel permeation and blockade (Garcia-Martinez et al. 2000). Further, E636 mutation weakly modulated pore blockade consistently with this residue serving to stabilise the channel’s selectivity filter or tetrameric structure. The suggested roles of these residues are consistent with TRPV1’s proposed structural and operational similarity to the potassium crystallographically-sited activation channel KcsA (Garcia-Martinez et al. 2000).
While TRPV1’s response to intracellular pH increase is mediated by the N-terminus, the effects of low pH, such as that occurring within damaged tissue due to local acidosis, are presumed to result from protonation of its extracellular acidic residues. Two such residues found near the channel’s pore confer different means of proton-mediated gating (Fig. 2.1). E600 appears to determine the channel’s response to other activators at low pH. Wild type TRPV1 exhibited dynamic heat-response potentiation when the extracellular pH decreased across a range reflecting tissue-damage associated local acidosis though, upon E600 mutation, the channel failed to exhibit such dynamic and robust response modulation (Jordt et al. 2000). E648 instead appears to confer the channel’s direct activation by protons as its mutation selectively abrogates proton-induced channel activation while responses to capsaicin and heat remained unaffected (Jordt et al. 2000). The E648A mutation hence supports the co-existence of independent activation pathways for different stimuli within TRPV1. However, it is worth noting that an alternative mutation of this residue, E648Q, has conversely been reported to selectively potentiate responses to capsaicin and not protons. Furthermore, the study described this same effect as a result of mutating residues E636 and D646 (Welch et al. 2000), both discussed above. In addition to E600 and E648, a study demonstrating that both proton-induced activation and sensitisation of TRPV1 are mediated by shifting the voltage dependence of activation curves towards more physiological membrane potentials elucidated that both of these processes depend on F660 within the pore region (Aneiros et al. 2011). Further still, the hydrophobic interactions that appear to mediate the coupling of proton binding with channel gating appear to rely on additional residues, one of which is located in the pore region at T633 (Ryu et al. 2007).
The pore region has further function in addition to regulating TRPV1’s permeability and mediating responses to acidic pH. S-nitrosylation of C616 and C621, which are located within the proximal pore domain, underlies NO-evoked responses of TRPV1 (Yoshida et al. 2006). Further, the lipid cholesterol, an essential structural component of cell membranes, has been shown to be an important regulator of TRPV1 and seems to bind to a cholesterol recognition amino acid consensus (CRAC) sequence within S5 (Liu et al. 2006b; Szoke et al. 2010; Jansson et al. 2013; Santha et al. 2010; Picazo-Juarez et al. 2011; Morales-Lazaro et al. 2013). The substitutions R579D and F582Q within this CRAC motif decrease the cholesterol response. However, depletion of cholesterol has also been shown to reduce TRPV1-mediated currents and, furthermore, decrease TRPV1 channel membrane expression (Liu et al. 2006b).
Along with the C-terminus (Brauchi et al. 2007), the pore domain appears to confer some of the channel’s thermosensing capability as mutating residues 613–627, thereby giving TRPV1 an artificial pore turret sequence, prevents the channel from responding to heat. This region was then observed to undergo heat-induced conformational changes not observed with ligand- and voltage-dependent activation (Yang et al. 2010). In addition, triple (N628K, N652T and Y653T) and double (N652T and Y653T) point mutant channels exhibit a shorter open time specifically in response to heat, while responses to capsaicin and pH are unaffected (Grandl et al. 2010). Endogenous heat-induced TRPV1 gating incorporates multiple open states of varying durations; those of greater length are diminished in these mutants. As with other regions in the molecule, TRPV1’s pore region appears to be functionally regulated by post-transcriptional modification. This was first established with the identification of an exclusive N-glycosylation site at N604 in cell lines (Jahnel et al. 2001). Western blotting of primary sensory nerve extracts later indicated the presence of both glycosylated and unglycosylated TRPV1. This variation in glycosylation state was observed in wild type TRPV1 (WT-TRPV1) but not the unglycosylated N604T mutant (N604T-TRPV1) upon expression in HEK293 cells. Variations in glycosylation state were reflected in the variability of capsaicin-induced currents and desensitisation observed across different WT-TRPV1-tranfected cells, while responses mediated by N604T-TRPV1 were uniform. Glycosylation ultimately appears to alter the maintenance of capsaicin-induced intracellular calcium level increases, acute desensitisation and pore dilation in a manner consistent with a substantial variability of endogenous TRPV1 glycosylation (Veldhuis et al. 2012).
2.6.4 The S1–S4 Module
The final TRPV1 component, the S1–S4 module (Fig. 2.1), confers many functional qualities also enabled by other regions of the molecule. However, by possessing the ‘vanilloid pocket’ between the first intracellular loop and S4, it is set apart by its predominant interaction with capsaicinoids and resiniferonoids. These compounds are highly lipophilic and it is presumed that they bind intracellularly having crossed the cell membrane. Mutational analysis supports an arrangement in which capsaicin interacts with the cytosolic aromatic residue Y511 via its vanillyl moiety and other polar residues such as S512 and R491 via hydrogen bonding (Jordt and Julius 2002). Alternatively, following the demonstration that I550T mutation in rabbit TRPV1 is sufficient to confer capsaicin sensitivity and that the reverse T550I mutation in rat and human TRPV1 prevents it, it has been suggested that capsaicin’s vanillyl moiety interacts at T550 while hydrophobic interaction occurs at Y511 (Gavva et al. 2004). Similarly, an additional mutation, L547M, was sufficient to enable high affinity binding of the ultra-potent vanilloid RTX in rabbit TRPV1 and the reverse mutations at positions 550 and 547 caused a loss of RTX binding in human and rat TRPV1 (Gavva et al. 2004). It was also demonstrated that anandamide, an endocannabinoid and endovanilloid with significant structural similarity to capsaicin, failed to activate the Y511A mutant. It hence appears that there are shared structural determinants of sensitivity to capsaicin and endogenous ligands (Jordt and Julius 2002).
Consistent with these mutational analyses, a modelling study suggested that capsaicin’s vanillyl moiety does indeed interact at Y511 via π–π and hydrophobic interactions and at S512 via hydrogen bonding, while its carbonyl group interacts via hydrogen bonds with both Y511 and K571 (Lee et al. 2011). The π–π and hydrophobic interactions at Y511 also appeared to occur with RTX binding while the residue’s hydroxyl group formed a hydrogen bond with K571 in order to embrace RTX’s phenyl ring. Additionally, RTX’s longer tail region contributed to hydrophobic interaction with M547, its C4-OH group hydrogen-bonded with T550 and its orthophenyl group exhibited hydrophobic interaction with L515. As the RTX molecule has phenyl rings at both ends, and there are hydrophobic residues at both ends of the binding region, the model also supported a secondary binding mode by which RTX’s vanillyl moiety pointed towards M547, its orthophenyl group oriented towards Y511, its C20-ester seemed to form hydrogen bonds with N551 and its C13-propenyl group formed hydrophobic interaction with L515 (Lee et al. 2011). In addition, the importance of a second hydrophobic site at F543 (which had been implicated in a previous RTX model alongside Y555 (Chou et al. 2004)) in binding a simplified RTX analogue was established and has interesting implications for the design of novel ligands (Lee et al. 2011).
In addition to its major roles in vanilloid binding, the S1-4 module houses residues key to TRPV1’s pH sensitivity. The mutational analyses that elucidated the above-described vanilloid binding site concomitantly established the involvement of S512 and R491 in mediating the channel’s response to protons. R491G and S512F mutations dramatically reduced proton-induced currents, while alternative mutations of these residues would preserve the responses to a greater extent (Jordt and Julius 2002). Additionally, as with T633 within the pore region, V538 within the second extracellular loop is required for the hydrophobic interactions involved in coupling proton binding with channel gating (Ryu et al. 2007). The S1–S4 module enables further post-transcriptional modification via S502, a consensus phosphorylation site for both PKA and PKC located within the first intracellular loop. Upon comparison with other predicted phosphorylation site mutants including T370D, PKA-mediated potentiation of heat response currents were most prominently reduced with S502D (Rathee et al. 2002), supporting the particular functional significance of this PKA phosphorylation site. As with S800 within the C-terminus, mutation studies have demonstrated that phosphorylation of S502 by PKC potentiates capsaicin-induced currents (Numazaki et al. 2002). Although TRPV1’s molecular determinants of voltage sensing have not yet been identified, such activation is generally associated with a series of positively charged amino acids within the channel’s S4. This is supported by the effect of mutating such residues within various voltage-gated ion channels on gating charge (Stuhmer et al. 1989; Papazian et al. 1991). Employing this approach has since confirmed that S4 and the second intracellular loop do indeed constitute part of the voltage sensor in TRPM8, offering interesting insight into how TRPV1 might sense voltage (Voets et al. 2007).
References
Ahern GP (2003) Activation of TRPV1 by the satiety factor oleoylethanolamide. J Biol Chem 278:30429–30434PubMed
Ahern GP, Brooks IM, Miyares RL, Wang XB (2005a) Extracellular cations sensitize and gate capsaicin receptor TRPV1 modulating pain signaling. J Neurosci 25:5109–5116PubMed
Ahern GP, Brooks IM, Miyares RL, Wang XB (2005b) Extracellular cations sensitize and gate capsaicin receptor TRPV1 modulating pain signaling. J Neurosci: Official J Soc Neurosci 25:5109–5116
Almasi R, Szoke E, Bolcskei K, Varga A, Riedl Z, Sandor Z, Szolcsanyi J, Petho G (2008) Actions of 3-methyl-N-oleoyldopamine, 4-methyl-N-oleoyldopamine and N-oleoylethanolamide on the rat TRPV1 receptor in vitro and in vivo. Life Sci 82:644–651PubMed
Aneiros E, Cao L, Papakosta M, Stevens EB, Phillips S, Grimm C (2011) The biophysical and molecular basis of TRPV1 proton gating. EMBO J 30:994–1002PubMedCentralPubMed
Appendino G, Daddario N, Minassi A, Moriello AS, de Petrocellis L, Di Marzo V (2005a) The taming of capsaicin. Reversal of the vanilloid activity of N-acylvanillamines by aromatic iodination. J Med Chem 48:4663–4669PubMed
Appendino G, de Petrocellis L, Trevisani M, Minassi A, Daddario N, Moriello AS, Gazzieri D, Ligresti A, Campi B, Fontana G, Pinna C, Geppetti P, Di Marzo V (2005b) Development of the first ultra-potent “capsaicinoid” agonist at transient receptor potential vanilloid type 1 (TRPV1) channels and its therapeutic potential. J Pharmacol Exp Ther 312:561–570PubMed
Appendino G, Harrison S, de Petrocellis L, Daddario N, Bianchi F, Schiano Moriello A, Trevisani M, Benvenuti F, Geppetti P, Di Marzo V (2003) Halogenation of a capsaicin analogue leads to novel vanilloid TRPV1 receptor antagonists. Br J Pharmacol 139: 1417–1424
Arniges M, Fernandez-Fernandez JM, Albrecht N, Schaefer M, Valverde MA (2006) Human TRPV4 channel splice variants revealed a key role of ankyrin domains in multimerization and trafficking. J Biol Chem 281:1580–1586PubMed
Bang S, Yoo S, Yang TJ, Cho H, Hwang SW (2012) 17(R)-resolvin D1 specifically inhibits transient receptor potential ion channel vanilloid 3 leading to peripheral antinociception. Br J Pharmacol 165:683–692PubMedCentralPubMed
Baumann TK, Martenson ME (2000) Extracellular protons both increase the activity and reduce the conductance of capsaicin- gated channels. J Neurosci 20:RC80
Bevan S, Hothi S, Hughes G, James IF, Rang HP, Shah K, Walpole CS, Yeats JC (1992) Capsazepine: a competitive antagonist of the sensory neurone excitant capsaicin. Br J Pharmacol 107:544–552PubMedCentralPubMed
Bhave G, Hu HJ, Glauner KS, Zhu W, Wang H, Brasier DJ, Oxford GS, Gereau RWT (2003) Protein kinase C phosphorylation sensitizes but does not activate the capsaicin receptor transient receptor potential vanilloid 1 (TRPV1). Proc Natl Acad Sci USA 100:12480–12485PubMedCentralPubMed
Bhave G, Zhu W, Wang H, Brasier DJ, Oxford GS, Gereau RWT (2002) cAMP-dependent protein kinase regulates desensitization of the capsaicin receptor (VR1) by direct phosphorylation. Neuron 35:721–731PubMed
Blednov YA, Harris RA (2009) Deletion of vanilloid receptor (TRPV1) in mice alters behavioral effects of ethanol. Neuropharmacology 56:814–820PubMedCentralPubMed
Bours MJ, Swennen EL, di Virgilio F, Cronstein BN, Dagnelie PC (2006) Adenosine 5’-triphosphate and adenosine as endogenous signaling molecules in immunity and inflammation. Pharmacol Ther 112:358–404PubMed
Brand L, Berman E, Schwen R, Loomans M, Janusz J, Bohne R, Maddin C, Gardner J, Lahann T, Farmer R et al (1987) NE-19550: a novel, orally active anti-inflammatory analgesic. Drugs Exp Clin Res 13:259–265PubMed
Brauchi S, Orta G, Mascayano C, Salazar M, Raddatz N, Urbina H, Rosenmann E, Gonzalez-Nilo F, Latorre R (2007) Dissection of the components for PIP2 activation and thermosensation in TRP channels. Proc Natl Acad Sci USA 104:10246–10251PubMedCentralPubMed
Brauchi S, Orta G, Salazar M, Rosenmann E, Latorre R (2006) A hot-sensing cold receptor: C-terminal domain determines thermosensation in transient receptor potential channels. J Neurosci 26:4835–4840PubMed
Burnstock G (2008) Purinergic signalling and disorders of the central nervous system. Nat Rev Drug Discov 7:575–590PubMed
Burnstock G (2009) Purinergic receptors and pain. Curr Pharm Des 15:1717–1735PubMed
Burnstock G, Kennedy C (2011) P2X receptors in health and disease. Adv Pharmacol 61:333–372PubMed
Carrier EJ, Kearn CS, Barkmeier AJ, Breese NM, Yang W, Nithipatikom K, Pfister SL, Campbell WB, Hillard CJ (2004) Cultured rat microglial cells synthesize the endocannabinoid 2-arachidonylglycerol, which increases proliferation via a CB2 receptor-dependent mechanism. Mol Pharmacol 65:999–1007PubMed
Caterina MJ, Schumacher MA, Tominaga M, Rosen TA, Levine JD, Julius D (1997) The capsaicin receptor: a heat-activated ion channel in the pain pathway. Nature 389:816–824PubMed
Cavanaugh DJ, Chesler AT, Braz JM, Shah NM, Julius D, Basbaum AI (2011) Restriction of transient receptor potential vanilloid-1 to the peptidergic subset of primary afferent neurons follows its developmental downregulation in nonpeptidergic neurons. J Neurosci 31:10119–10127PubMedCentralPubMed
Cesare P, Dekker LV, Sardini A, Parker PJ, McNaughton PA (1999) Specific involvement of PKC-epsilon in sensitization of the neuronal response to painful heat. Neuron 23:617–624PubMed
Cesare P, McNaughton P (1996) A novel heat-activated current in nociceptive neurons and its sensitization by bradykinin. Proc Natl Acad Sci U S A 93:15435–15439PubMedCentralPubMed
Chavez AE, Chiu CQ, Castillo PE (2010) TRPV1 activation by endogenous anandamide triggers postsynaptic long-term depression in dentate gyrus. Nat Neurosci 13:1511–1518PubMedCentralPubMed
Cho W, Stahelin RV (2005) Membrane-protein interactions in cell signaling and membrane trafficking. Annu Rev Biophys Biomol Struct 34:119–151PubMed
Cholewinski A, Burgess GM, Bevan S (1993) The role of calcium in capsaicin-induced desensitization in rat cultured dorsal root ganglion neurons. Neuroscience 55:1015–1023PubMed
Chou MZ, Mtui T, Gao YD, Kohler M, Middleton RE (2004) Resiniferatoxin binds to the capsaicin receptor (TRPV1) near the extracellular side of the S4 transmembrane domain. Biochemistry 43:2501–2511PubMed
Chuang HH, Prescott ED, Kong H, Shields S, Jordt SE, Basbaum AI, Chao MV, Julius D (2001) Bradykinin and nerve growth factor release the capsaicin receptor from PtdIns(4,5)P2-mediated inhibition. Nature 411:957–962PubMed
Cortright DN, Szallasi A (2004) Biochemical pharmacology of the vanilloid receptor TRPV1. An update. Europ J Biochem/FEBS 271:1814–1819
Cromer BA, McIntyre P (2008) Painful toxins acting at TRPV1. Toxicon 51:163–173PubMed
Culotta E, Koshland DE Jr (1992) No news is good news. Science 258:1862–1865
Decottignies A, Goffeau A (1997) Complete inventory of the yeast ABC proteins. Nat Genet 15:137–145PubMed
Devane WA, Hanus L, Breuer A, Pertwee RG, Stevenson LA, Griffin G, Gibson D, Mandelbaum A, Etinger A, Mechoulam R (1992) Isolation and structure of a brain constituent that binds to the cannabinoid receptor. Science 258:1946–1949PubMed
Dhaka A, Uzzell V, Dubin AE, Mathur J, Petrus M, Bandell M, Patapoutian A (2009) TRPV1 is activated by both acidic and basic pH. J Neurosci 29:153–158PubMedCentralPubMed
Di Marzo V, Blumberg PM, Szallasi A (2002) Endovanilloid signaling in pain. Curr Opin Neurobiol 12:372–379PubMed
Di Marzo V, de Petrocellis L, Sepe N, Buono A (1996) Biosynthesis of anandamide and related acylethanolamides in mouse J774 macrophages and N18 neuroblastoma cells. Biochem J 316(Pt 3):977–984PubMedCentralPubMed
Di Marzo V, Fontana A, Cadas H, Schinelli S, Cimino G, Schwartz JC, Piomelli D (1994) Formation and inactivation of endogenous cannabinoid anandamide in central neurons. Nature 372:686–691PubMed
Dietrich A, Kalwa H, Rost BR, Gudermann T (2005) The diacylgylcerol-sensitive TRPC3/6/7 subfamily of cation channels: functional characterization and physiological relevance. Pflugers Arch 451:72–80PubMed
Dinitto JP, Cronin TC, Lambright DG (2003) Membrane recognition and targeting by lipid-binding domains. Sci STKE: Sig Transduct Knowl Environ 213:re16
Docherty RJ, Yeats JC, Bevan S, Boddeke HW (1996) Inhibition of calcineurin inhibits the desensitization of capsaicin-evoked currents in cultured dorsal root ganglion neurones from adult rats. Pflugers Arch 431:828–837PubMed
Dray A (1992) Neuropharmacological mechanisms of capsaicin and related substances. Biochem Pharmacol 44:611–615PubMed
Eisenach JC, Hood DD, Curry R (2002) Preliminary efficacy assessment of intrathecal injection of an American formulation of adenosine in humans. Anesthesiology 96:29–34PubMed
el Kouhen R, Surowy CS, Bianchi BR, Neelands TR, McDonald HA, Niforatos W, Gomtsyan A, Lee CH, Honore P, Sullivan JP, Jarvis MF, Faltynek CR (2005) A-425619 [1-isoquinolin-5-yl-3-(4-trifluoromethyl-benzyl)-urea], a novel and selective transient receptor potential type V1 receptor antagonist, blocks channel activation by vanilloids, heat, and acid. J Pharmacol Exp Ther 314:400–409PubMed
Erler I, Hirnet D, Wissenbach U, Flockerzi V, Niemeyer BA (2004) Ca2+-selective transient receptor potential V channel architecture and function require a specific ankyrin repeat. J Biol Chem 279:34456–34463PubMed
Everaerts W, Gees M, Alpizar YA, Farre R, Leten C, Apetrei A, Dewachter I, van Leuven F, Vennekens R, de Ridder D, Nilius B, Voets T, Talavera K (2011) The capsaicin receptor TRPV1 is a crucial mediator of the noxious effects of mustard oil. Curr Biol 21:316–321PubMed
Fajardo O, Meseguer V, Belmonte C, Viana F (2008) TRPA1 channels mediate cold temperature sensing in mammalian vagal sensory neurons: pharmacological and genetic evidence. J Neurosci 28:7863–7875PubMed
Faussone-Pellegrini MS, Taddei A, Bizzoco E, Lazzeri M, Vannucchi MG, Bechi P (2005) Distribution of the vanilloid (capsaicin) receptor type 1 in the human stomach. Histochem Cell Biol 124:61–68PubMed
Fernandes ES, Fernandes MA, Keeble JE (2012) The functions of TRPA1 and TRPV1: moving away from sensory nerves. Br J Pharmacol 166:510–521PubMedCentralPubMed
Fischer MJ, Btesh J, McNaughton PA (2013) Disrupting sensitization of transient receptor potential vanilloid subtype 1 inhibits inflammatory hyperalgesia. J Neurosci 33:7407–7414PubMed
Fu J, Gaetani S, Oveisi F, Lo Verme J, Serrano A, Rodriguez de Fonseca F, Rosengarth A, Luecke H, di Giacomo B, Tarzia G, Piomelli D (2003) Oleylethanolamide regulates feeding and body weight through activation of the nuclear receptor PPAR-alpha. Nature 425:90–93
Fujiwake H, Suzuki T, Oka S, Iwai K (1980) Enzymatic formation of capsaicinoid from vanillylamine and iso-type fatty acids by cell-free extracts of Capsicum annuum var. annuum cv. Karayatsubus. Agric Biol Chem 44:2907–2912
Garcia-Martinez C, Morenilla-Palao C, Planells-Cases R, Merino JM, Ferrer-Montiel A (2000) Identification of an aspartic residue in the P-loop of the vanilloid receptor that modulates pore properties. J Biol Chem 275:32552–32558PubMed
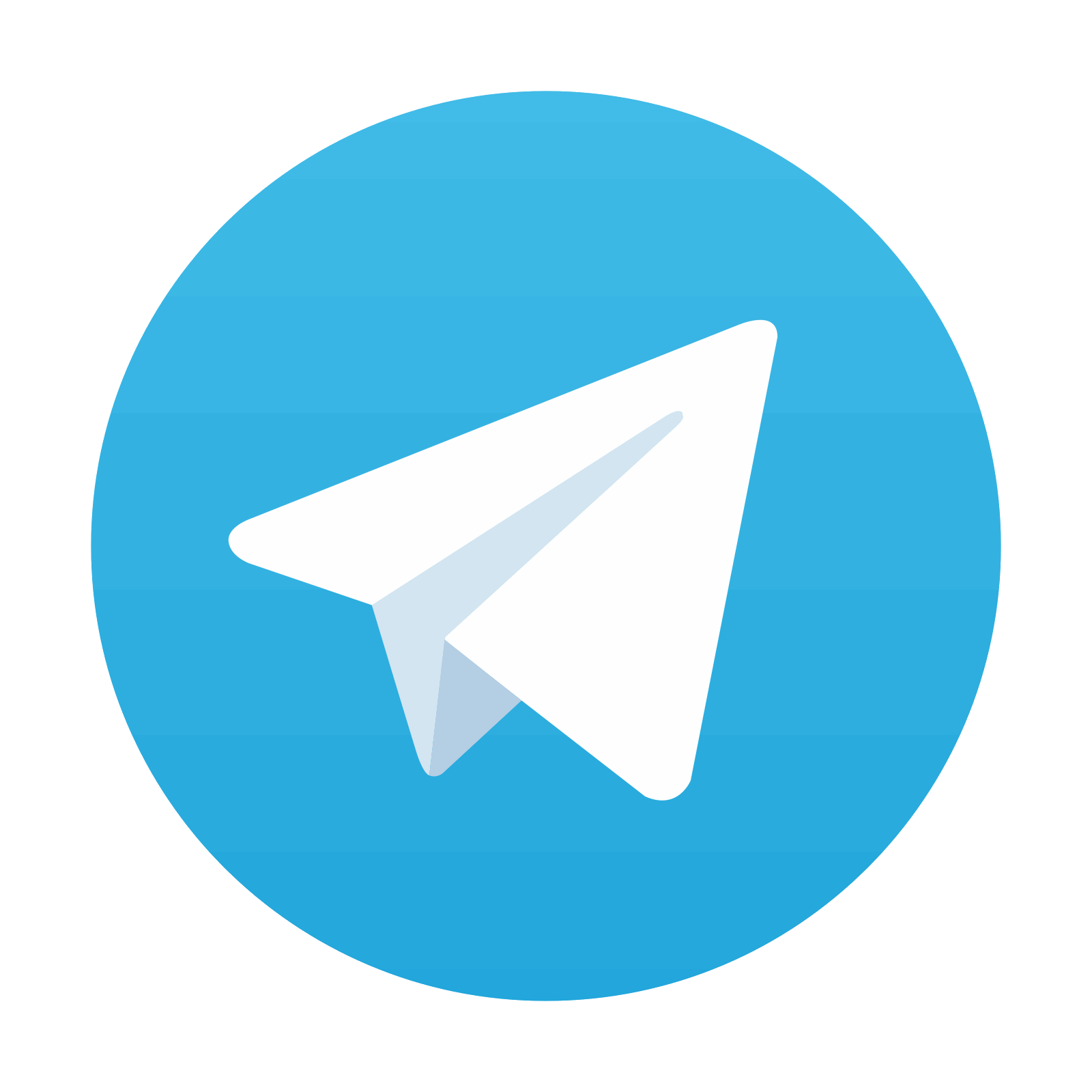
Stay updated, free articles. Join our Telegram channel

Full access? Get Clinical Tree
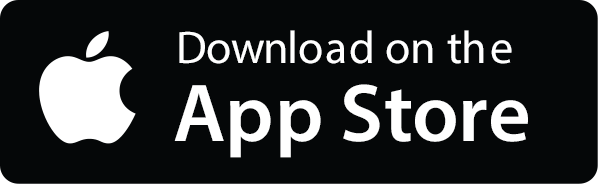
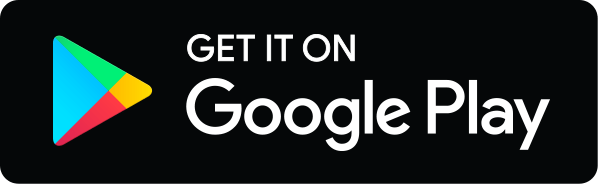