The Chemical Control of Physiology
Outline
Pharmacology and Cellular Drug Response1
New Terminology2
Pharmacological Targets2
Dose–Response Curves5
Linking Observed Pharmacology With Molecular Mechanism12
Descriptive Pharmacology: I15
Summary16
This chapter discusses how drug response is quantified with dose–response curve and how this leads to classifications of drugs based on effect (i.e., drugs that produce observed change in cellular processes are termed agonists and those that block such effects are antagonists). The dependence of observed potency of an agonist upon two drug-related parameters (affinity, efficacy) and two cell-dependent parameters (target density and efficiency of target coupling) is also discussed. The way that different tissues process drug stimulus to provide tissue response is considered, along with the null method which can be used to negate cell-dependent effects on drug activity to provide system-independent indices of drug activity. This is imperative in pharmacology as drugs are almost always studied in test systems, and not in therapeutic one(s).
Keywords
affinity, agonists, antagonists, dose–response curves, efficacy, null method, potency.
Pharmacology and Cellular Drug Response
Pharmacology (from the Greek φάρμακον, pharmakon, “drug” and -λογία, -logia, the study of) concerns drug action on physiological systems (physiology from the Greek φύσις, physis, “nature, origin” and -λογία, -logia is the study of the mechanical, physical and biochemical functions of living organisms). With regard to the application of pharmacology to the discovery of drugs for therapeutic benefit, the main focus of pharmacological theories, procedures and mechanisms relates to the chemical control of physiological processes. Insofar as the understanding of these physiological processes benefits the pharmacologic pursuit of drugs, pharmacology and physiology are intimately related. However, it will also be seen that complete understanding of the physiologic processes involved is not a prerequisite to the effective use of pharmacology in the drug discovery process. In fact, often an operational approach is utilized whereby the complexity of the physiology is represented by simple surrogate mathematical functions.
A unique feature of pharmacology is that the effect of the drug is often observed indirectly, that is, while the drug affects a select biochemical process in the cell, the outcome to an observer is an overall change in the state of the whole organism, and this is often the result of multiple interacting cellular processes. A major aim of pharmacology is to define the molecular events in initiating drug effects, since these define the action of drugs in all systems. If quantified correctly, this information can be used to predict drug effect at the pharmacological target in all systems including therapeutic one(s). At this point, it is useful to define what is meant by pharmacological target.
New Terminology
The following new terms will be introduced in this chapter:
• Affinity: The propensity of a drug molecule to associate closely with a drug target.
• Agonists: Drugs that produce an observable change in the state of a physiological system.
• Antagonists: Drugs that may not produce a direct effect, but do interfere with the production of cellular response to an agonist.
• Dose–response curve: The relationship between doses (if the drug is used in vivo) or concentrations (if used in vitro) of a drug and pharmacologic effect.
• Drug target: The protein (or in some cases DNA, mRNA) to which a drug binds to elicit whatever pharmacologic effect it will produce. These proteins can be seven transmembrane (or one transmembrane) receptors, enzymes, nuclear receptors, ion channels or transport proteins.
• EC50: Concentration of agonist producing half the maximal response to the same agonist; usually expressed for calculation and statistical manipulation as the pEC50, negative logarithm of the molar concentration producing 50% response.
• Efficacy: The change in state of the drug target upon binding of a drug.
• Efficiency of target coupling: The relationship between the net quanta of activation given to a cell and the number of drug targets available for activation.
• Full agonists: Agonists that produce the full maximal response that the system can produce.
• Null method: The comparison of equiactive concentrations (or doses) of drug to cancel the cell-based processing of drug response. The assumption is that equal responses to a given agonist are processed in an identical manner by the cell.
• Partial agonists: Agonists that produce a maximal response that is of lower magnitude than the maximal response that the system can produce to maximal stimulation.
• pEC50: The negative logarithm of EC50 values. For arithmetic and/or statistical manipulation, numbers must be normally distributed. This is true only of pEC50s, not of EC50s; thus all averages, estimates or error and statistical procedures must use pEC50.
• Potency: The concentration (usually molar) of drug needed to produce a defined response or effect.
• Target density: The concentration of drug targets at the site of activation, i.e., on the cell surface for receptors.
Pharmacological Targets
The term “pharmacological target” refers to the biochemical entity to which the drug first binds in the body to elicit its effect. There are a number of such entities targeted by drug molecules. In general, they can be proteins such as receptors, enzymes, transporters, ion channels, or genetic material such as DNA. The prerequisite for pharmacologic targets is that they have the ability to discern differences in electronic structure minute enough to be present in small drug-like molecules; in this regard the most predominant targets for drugs are protein in nature. Proteins have the tertiary three-dimensional structure necessary for detailed definition of the electronic forces involved in small molecule binding. Signals are initiated through complementary binding of drug molecules to protein conformations that have a physiological purpose in the cell. The act of these molecules binding to the protein will change it, and with that change a pharmacologic effect will occur.
At this point, it is worth considering the beginning and end processes. The first process is the drug binding to the target. The result(s) of this process are totally dependent on the affinity and efficacy of the drug. These are drug parameters unique to its chemical structure. In pharmacologic terms, this is the most important effect, since it occurs in each and every tissue and organ possessing the target. Therefore, characterization of this event enables a general quantification of drug-target activity to be made in the test system, which will also be true for all systems including the therapeutic one. Therefore, the characterization of affinity and efficacy become the primary aim of pharmacologic analysis. However, it can be seen that the various (and variable) biochemical reactions linking the target to cellular response intervene, thereby causing a tissue-dependent abstraction of the link between affinity and efficacy and observed cellular potency. The magnitude of this abstraction depends upon the number of responding target units and the efficiency of target coupling.
The major protein target classes are membrane receptors, enzymes, ion channels and transporter proteins. Of these, the most prominent drug targets are receptors. While there are a number of types of receptor, one of the most important from the standpoint of therapeutic drug targets is seven transmembrane receptors (7TMRs). These are so-called because they span the cell membrane seven times to form complex recognition domains both outside and inside the cell. These proteins are capable of recognizing chemicals such as hormones and neurotransmitters present in the extracellular space, and transmit signals from these to the cell interior. Due to the fact that these are on the cell surface and thus exposed to the extracellular space, these entities were the subject of experiments that originally defined the receptor concept (see Box 1.1 for history).
Box 1.1
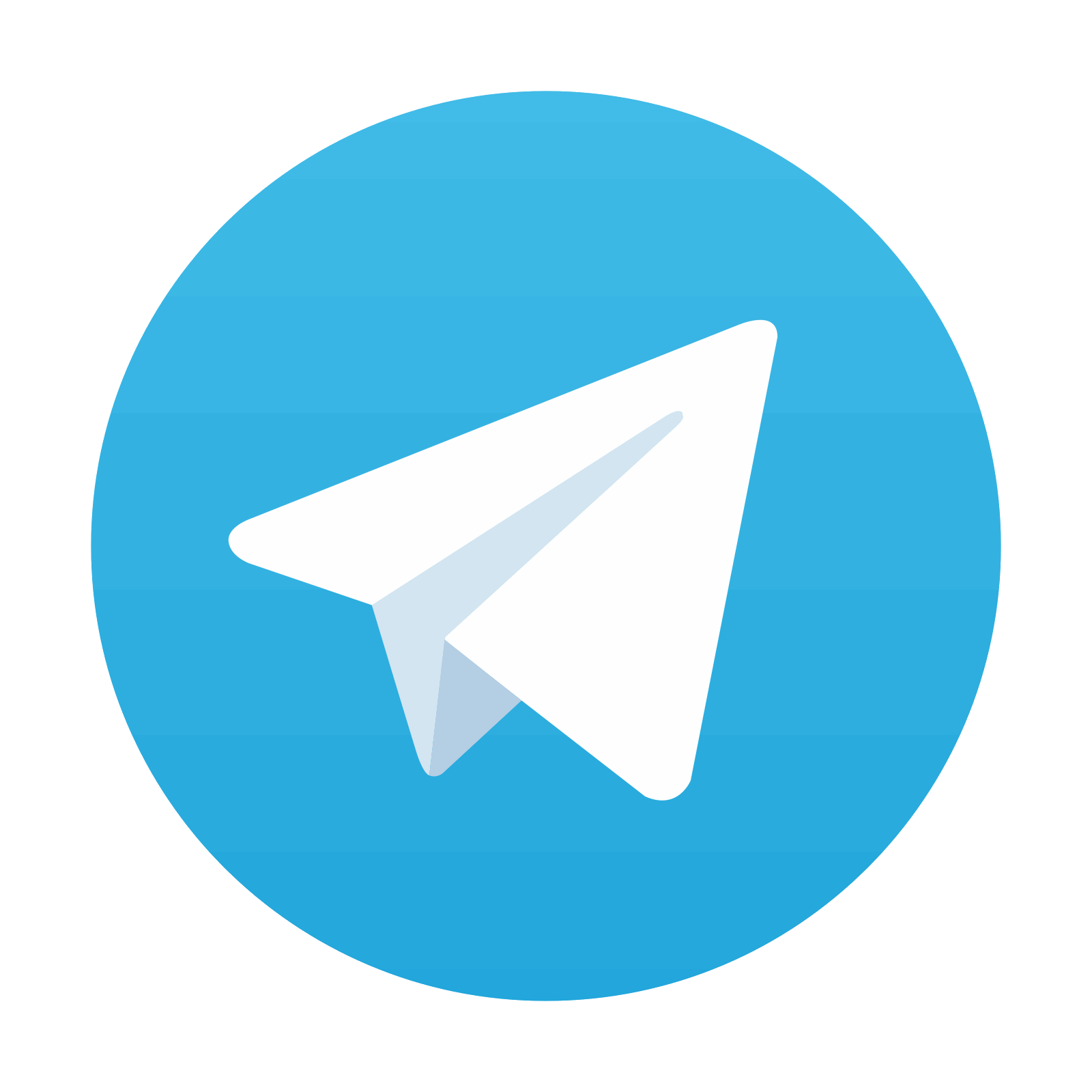
![]() |
Numerous physiologists and pharmacologists contributed to the concept of “receptor” as minimal recognition units for chemicals in cells. Paul Ehrlich (1854–1915) studied dyes and bacteria and determined that there are “chemoreceptors” (he proposed a collection of “amboreceptors,” “triceptors” and “polyceptors”) on parasites, cancer cells and microorganisms that could be exploited therapeutically.
![]() |
John Newport Langley (1852–1926), as Chair of the Physiology Department in Cambridge, studied the drugs jaborandi (containing the alkaloid pilocarpine) and atropine. He concluded that receptors were “switches” that received and generated signals and that these switches could be activated or blocked by specific molecules.
![]() |
A. J. Clark (1885–1941), who could be considered the father of modern receptor pharmacology, was one of the first to suggest from studies of acetylcholine and atropine that a unimolecular interaction occurs between a drug and a “substance on the cell.” As stated by Clark: “… it is impossible to explain the remarkable effects observed except by assuming that drugs unite with receptors of a highly specific pattern…”
Historically, while the actual physical nature of receptors was unknown, it was realized that a distinct entity on the cell surface allows cells to recognize drugs and read the chemical information encoded in them. Early concepts of receptors likened them to locks with drugs as keys (i.e., as stated by the biologist Paul Ehrlich: “… substances can only be anchored at any particular part of the organism if they fit into the molecule of the recipient complex like a piece of mosaic finds its place in a pattern…”). The main value of receptors is that they put order into the previously disordered world of physiology. For example, it has been observed that the hormone epinephrine produces a wealth of dissimilar physiological responses such as bronchiole muscle relaxation, cardiac muscle positive inotropy, chronotropy and lusitropy, melatonin synthesis, pancreatic, lacrimal and salivary gland secretion, decreased stomach motility, urinary bladder muscle relaxation, skeletal muscle tremor and vascular relaxation. The understanding of how such a vast array of biological responses could be mediated by a single hormone is difficult until it is realized that these processes are all mediated by the interaction of epinephrine with a single receptor protein, in this case the β-adrenoceptor. Thus, when this receptor is present on the surface of any given cell it will respond to epinephrine, and the nature of that response will be determined by the encoding of the receptor excitation produced by epinephrine to the cytosolic biochemical cascades controlling cellular function. In a conceptual sense, the term “receptor” can refer to any single biological entity that responds to drugs (i.e., enzymes, ion channels, transport proteins, DNA and structures in the nucleus). This information is transmitted through changes in protein shape (conformation) i.e., the drug does not enter the cell nor does the receptor change the nature of the drug (as an enzyme would).
Pharmacologic targets can be used to modify physiological processes. Specifically, chemicals can be used to cause activation, blockade or modulation of protein receptors and ion channel targets. For enzymes and transporter proteins the main drug effect is inhibition of ongoing basal activity of these targets (Chapter 6 discusses these targets in detail). Another difference between these target classes is location; while receptors, ion channels and transporter proteins are usually found on the cell surface (exposed to the extracellular space), enzymes are most often found in the cytosol of the cell (drugs must enter the cell to act on enzymes). Exceptions to this general rule are nuclear receptors which reside in the cell nucleus. Finally, it should be recognized that there are other drug targets present in the cell, such as DNA, and that chemicals can have physical effects (i.e., membrane stabilization) that can change cellular function.
Pharmacologic effects on cells can include a wide variety of outcomes, from changes in the mechanical function of cells (i.e., cardiac contractility, contraction of bronchiole smooth muscle), biochemical metabolic effects (levels of second messengers such as calcium ion or cyclic AMP) and modulation of basal activity (level of catalytic degradation of cyclic AMP by enzymes such as phosphodiesterase, rate of uptake of neuroamines such as norepinephrine and serotonin).
It is worth considering the process of target choice in the drug discovery process. Specifically, effective prosecution of any drug target requires a minimal effort in resources and time (perhaps 1 to 2 years per target), thus it can be seen how an incorrect choice of target could lead to a serious dissimulation in the drug discovery process. While there are considerations in target choice, such as target tractability (how difficult it is to produce a molecule to alter the behavior of the target), one of the most important factors is a strong association with the disease that is being treated. It has been estimated that there are approximately 600 to 1500 possible drug targets that may be valid to pursue for therapy. These are made up of genes that are known to be associated with diseases and that also code for protein that may be modified through binding to a small molecule. [1] No discovery program could pursue a number of genes close to the number available, making target validation a very important step in the process. Table 1.1 shows some of the factors involved in the process of target validation, with particular reference to the problem of HIV-1 viral entry to cause Acquired Immune Deficiency Syndrome (AIDS). As a preface to the discussion of cellular drug effect, it is useful to consider the major pharmacological tool used to quantify it, namely, the dose–response curve.
1Genetically altered mouse that does not naturally express the CCR5 receptor. | ||
Factor | CCR5 in AIDS | Reference |
---|---|---|
• Target is linked to sensitivity to disease | • CCR5 receptors must be present on cell surface for HIV-1 infection | [2] and [3] |
• Cell level of target alters sensitivity and course of disease | • Down-regulation of CCR5 leads to resistance to HIV-1 infection | [4] |
• Genetically high levels of CCR5 lead to rapid progression to AIDS | [5] | |
• Interference with target will not lead to harm | • CCR5 knockout mouse1 lacks the receptor but is otherwise healthy | [6] |
• Ligands for target interfere with disease | • CCR5 interaction with chemokines interfere with HIV-1 infection | [7], [8], [9], [10] and [11] |
• Patients with high circulating levels of chemokine have retarded progression to AIDS | [12] and [13] | |
• Specific genetic association | • Δ32 deletion in CCR5 gene leads to lack of receptor expression and complete resistance to AIDS | [14], [15], [16], [17] and [18] |
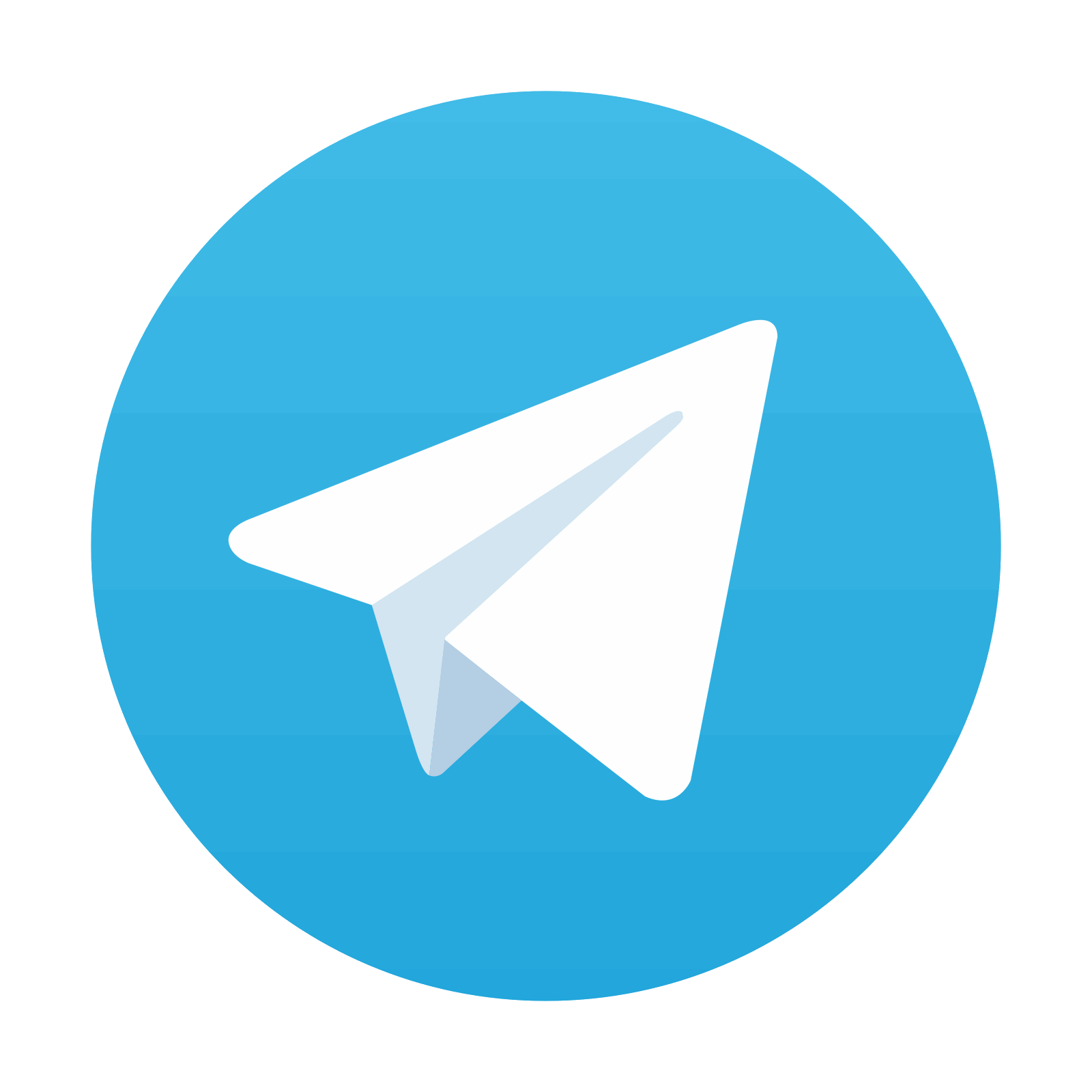
Stay updated, free articles. Join our Telegram channel

Full access? Get Clinical Tree
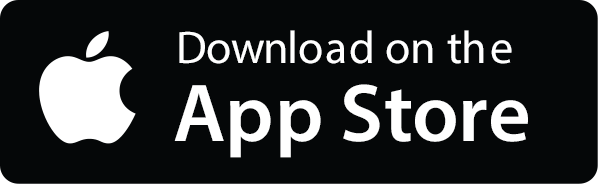
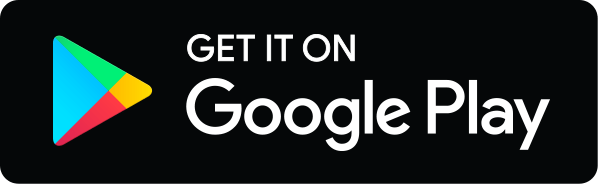
