Pharmacogenomics
Isabelle Mercier
Andrew M. Peterson
Amalia M. Issa
PHARMACOGENOMICS AND PERSONALIZED MEDICINE
Interpatient variability in drug therapy response is a well-known pharmacotherapeutic concept. Indeed, as far back as 1892, William Osler is reputed to have said “if it were not for the great variability among individuals, medicine might be a science and not an art” (Golden, 2004). In addition to factors such as age, sex, drug-drug interactions, and comorbidities, genetics also is known to play a role in interpatient variability of drug response.
The ability of genetic variability (inherited differences) to influence therapeutic drug response is the basis of pharmacogenetics and pharmacogenomics. Generally, pharmacogenetics refers to single or a few gene variations (called polymorphisms), whereas pharmacogenomics refers more broadly to the genome-wide (or an individual’s entire DNA sequence) effects on drug therapy. Personalized medicine is a more recently coined term that includes pharmacogenetics/pharmacogenomics and refers to “an emerging practice of medicine that uses an individual’s genetic profile to guide decisions made in regard to the prevention, diagnosis, and treatment of disease” (National Human Genome Research Institute, 2015) according to the National Institutes of Health (NIH). This term is now evolving to be “precision medicine.”
We can think of pharmacogenomics as the basic science of personalized medicine, and indeed, much of the progress that has been made in the field of personalized medicine to date has been largely focused on pharmacogenomics.
This chapter is geared toward future clinicians, particularly nurse practitioners and physician assistants, to provide them with an overview of pharmacogenomics, the current state of the science, including some pertinent examples, some relevant applications, and promises, pitfalls, and policy implications.
BASIC CONCEPTS
Because pharmacogenomics is rooted in genetics, it is helpful to review some basic genetic concepts. Several of the definitions of key terms that are associated with genetics and pharmacogenomics can be found in Box 10.1.
The human genome is the underpinning to every human’s individuality. With the exception of identical twins, the genome is different for every individual, though in the grand scheme of things there are only small differences among people’s DNA that make us unique. The human genome consists of approximately 3 billion base pairs, 99.9% of which are the same among all humans with only 0.1% variation among individuals. The variations that occur with DNA (polymorphisms), along with environmental and dietary factors, create a patient’s individuality, susceptibility to disease, and response to treatments. Included in this individuality is a person’s ability to absorb, distribute, metabolize, and excrete drugs. Understanding the genetic components affecting these pharmacokinetic processes can help the clinician tailor treatment for a patient.
Each human has 23 pairs of chromosomes—22 are autosomal and look the same in males and females, and 1 pair is the sex chromosome, in which females have two X chromosomes and males have an X and a Y chromosome (Figure 10.1). These chromosomes reside in the nucleus of a cell (Figure 10.2). Each chromosome is composed of DNA, which carries the genetic information for the individual. Each chromosome can have hundreds or thousands of genes; it is estimated that there are more than 25,000 genes on the human genome. However, genes only make up about 1% of the total DNA found in humans.
Genes function to produce proteins involved in the millions of biological processes that support the function of
the body every day. Genes that mutate or malfunction can have profound effects on the body. In the case in which a single gene mutates or malfunctions, the result is a monogenic disease, such as sickle cell anemia or cystic fibrosis. In most cases, however, there are multiple genes involved in the disease process. These are referred to as polygenic disorders.
the body every day. Genes that mutate or malfunction can have profound effects on the body. In the case in which a single gene mutates or malfunctions, the result is a monogenic disease, such as sickle cell anemia or cystic fibrosis. In most cases, however, there are multiple genes involved in the disease process. These are referred to as polygenic disorders.
BOX 10.1
Definitions
Definitions
Adenine (A)—One of the four nucleotide bases. Pairs with thymine
Alleles—Multiple versions of a gene. Each person typically inherits two alleles of each gene, one from the mother and one from the father.
Biomarkers—Molecules that indicate the status of a biological process Chromosome—The organized structure of DNA and proteins, the “double helix.” It contains genes and nucleotide sequences.
Cytosine (C)—One of the four nucleotide bases. Pairs with guanine
DNA (deoxyribonucleic acid)—A nucleic acid that contains genetic information and/or instructions used in the function of living organisms
Exon is the portion of a gene that codes for amino acids
Gene—A sequence of DNA that codes for a type of protein or RNA, serving a particular function in a cell
Genome—All of the genetic material in chromosomes of an organism
Guanine (G)—One of the four nucleotide bases. Pairs with cytosine
Haplotype—A combination of alleles. A haplotype may be a single locus of alleles, multiple loci, or even an entire chromosome.
Nucleotide—Molecules that make up the structural units of DNA and RNA. The four DNA nucleotides are adenine, cytosine, guanine, and thymine. For RNA, uracil is substituted for thymine.
Personalized medicine—A more recently coined term that includes pharmacogenetics/pharmacogenomics and refers to “an emerging practice of medicine that uses an individual’s genetic profile to guide decisions made in regard to the prevention, diagnosis, and treatment of disease” (National Human Genome Research Institute, 2015).
Pharmacogenetics refers to the study of inherited differences in single gene variations (called polymorphisms) or a few genes, in drug metabolism and response.
Pharmacogenomics refers to the effects of genome-wide (or an individual’s entire DNA sequence) effects on drug therapy.
Polymorphism—DNA sequence variation
RNA (ribonucleic acid)—A nucleic acid that carries genetic information and produces proteins used in the function of living organisms
SNPs (single nucleotide polymorphism)—A DNA sequence variation occurring when a single nucleotide differs between members of a species
Thymine (T)—One of the four nucleotide bases. Pairs with adenine
Wild type—The normal, as opposed to the mutant, gene, or allele
Polygenic disorders may appear as a single clinical disorder but at the molecular level have multiple biomarkers. Biomarkers are molecules that indicate the status of a biological process. Examples of biomarkers include prostate-specific antigen (PSA) for prostate cancer or blood glucose level for diabetes. Genetic biomarkers, specific DNA sequences, are also being discovered.
The building blocks of DNA are the four nucleotide bases, including the two purines—adenine (A) and guanine (G)—and the two pyrimidines—thymine (T) and cytosine (C). DNA strands are linked through base pairing of the pyrimidines with the purines (A with T, G with C), conceptually forming the well-known double helix (see Figure 10.2). The arrangement of these base pairs along each chromosome is
called the DNA sequence. Variations in the base pairings range from single nucleotide polymorphisms, insertions or deletions of a nucleotide base, to changes in the number of copies of genes. These variations can alter the production or function of proteins, thus creating the variation in the expression of a disease or the response to drug therapy.
called the DNA sequence. Variations in the base pairings range from single nucleotide polymorphisms, insertions or deletions of a nucleotide base, to changes in the number of copies of genes. These variations can alter the production or function of proteins, thus creating the variation in the expression of a disease or the response to drug therapy.
Single Nucleotide Polymorphisms
An SNP is a variation in the DNA sequence that differs between members of a species or paired chromosomes in an individual. For example, the following are two sequenced DNA fragments from different individuals: AAGCTA and AAGTTA. Note the only difference between these sequences is the substitution of thymidine (T) for cytosine (C). In this case, there are two versions (or alleles) of this gene. Each person typically inherits two alleles of each gene: one from the mother and one from the father. There can be up to 10 million SNPs in humans, but only those SNPs on coding regions of the gene or the area of the DNA responsible for turning genes on or off have an effect on humans. This concept of SNPs and differing alleles is important in the study of pharmacogenomics, as many of the genes responsible for drug activity and metabolism (e.g., cytochrome P-450 [CYP]) have different alleles on the same gene, producing different metabolic effects.
CLINICAL APPLICATIONS OF PHARMACOGENOMICS
A major current issue in medical care is that many therapies given to patients to treat their diseases (e.g., cardiovascular, cancer, diabetes) are misaligned with the patient’s genetic makeup. There are two main consequences that directly result from this lack of molecular knowledge at the time of drug treatment: (1) patients can be given a therapy that inefficiently treats the underlying cause of the disease (lack of therapeutic effect) and (2) patients can be given a medication that can lead to adverse drug reactions (ADRs) that can be harmful or even fatal due to a difference in their genetic makeup. Clinicians and health care professionals must understand and acknowledge that some patients could be genetically predisposed to respond differently to a given drug. This genetic information should then be utilized to assure tailored therapy and safety.
The main organ involved in detoxification/metabolism of drugs is the liver. The cytochrome P-450 (CYP) super-families of liver enzymes are key players in drug metabolism as they are directly involved in the modification and processing of approximately 75% of all medications taken (Di, 2014; Guengerich, 2004). The impact of genetic modifications in these CYP enzymes has therefore an important impact on
patient treatment. The following examples are focused on genetic alterations in these CYP enzymes and their clinical implications.
patient treatment. The following examples are focused on genetic alterations in these CYP enzymes and their clinical implications.
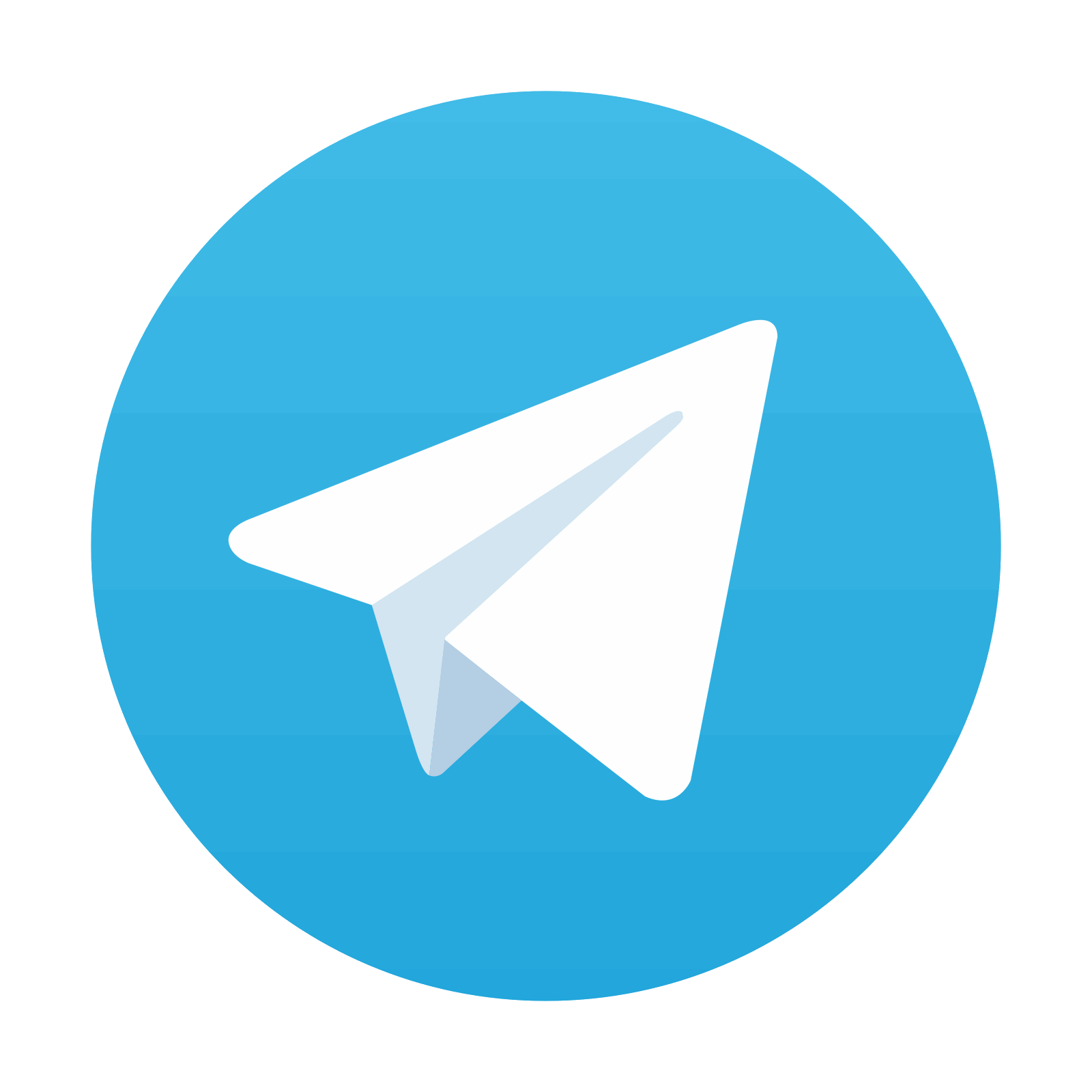
Stay updated, free articles. Join our Telegram channel

Full access? Get Clinical Tree
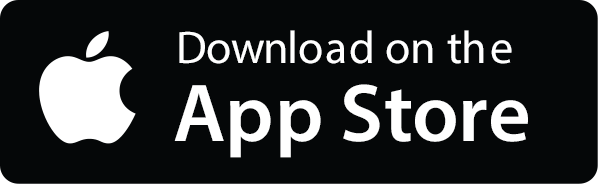
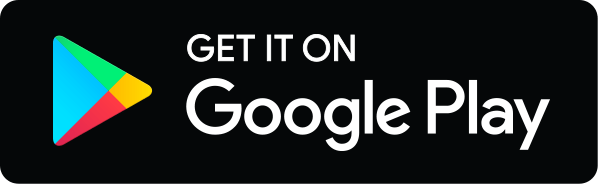