, Kyle John Wilby2 and Mary H. H. Ensom1
(1)
Faculty of Pharmaceutical Sciences, The University of British Columbia, Vancouver, Canada
(2)
College of Pharmacy, Qatar University, Doha, Qatar
This chapter summarizes the clinical evidence supporting antimalarial combination therapy and provides details of studies that describe in vivo and in vitro drug interactions in which co-administered antimalarial or non-antimalarial drugs affect the pharmacokinetics of various antimalarials and vice versa.
7.1 Summary of Clinical Evidence for Combination Therapy
Over the last few decades, significant amounts of literature have been published regarding combination therapy for treating malaria. Combination therapy is used to enhance efficacy and decrease resistance to single-agent therapy. In particular, the advent of artemisinin-based regimens has greatly improved treatment outcomes for malaria worldwide. As mentioned in Chap. 1, the currently recommended combination therapies for malaria are artemether-lumefantrine, artesunate-amodiaquine, artesunate-mefloquine, artesunate-sulfadoxine-pyrimethamine (WHO 2010). Additionally, dihydroartemisinin-piperaquine is a promising newly established combination.
From a pharmacodynamics perspective, the combinations have been shown to be effective for malaria treatment. Most studies have been completed with P. falciparum but some also exist for P. vivax. As giving single-agent treatment is now unethical, most studies compare combination treatment regimens to each other, in order to assess efficacy. Therefore, it is difficult to assess interactions between agents at this level. However, a recent Cochrane review provides an overview of efficacy data and the results of this review are summarized below (Sinclair et al. 2009).
The Cochrane review identified 50 studies that assessed ACTs head to head in uncomplicated P. falciparum (Sinclair et al. 2009). Overall, all five regimens achieved failure rates of less than 10 %. It was noted that dihydroartemisinin-piperaquine performed well compared to other agents (significantly better than artesunate-mefloquine and artemether-lumefantrine). This may be due in part to less established resistance to this newly available ACT. It was also noted that amodiaquine plus sulfadoxine-pyrimethamine was inferior to ACTs, suggesting the importance of artemisinin-based therapy. Major conclusions from the review are that ACTs still remain first line in malaria management. However, there needs to be continual surveillance throughout endemic regions to ensure therapy maintains efficacy and resistance does not counteract therapeutic success.
7.2 Pharmacodynamic Drug–Drug Interactions In Vitro
Table 7.1 summarizes the known in vitro interactions between recommended antimalarials and other agents.
Table 7.1
Summary of in vitro pharmacodynamic drug interactions
Drug or drug class | Antagonism | Additive | Synergism | None or inconclusive |
---|---|---|---|---|
Artemisinins • Artemisinin • Artemether • Artesunate • DHA | Cepharanthine Choloroquine Ketoconazole | Amphothericin B Azithromycin Chloroquine Clindamycin Clotrimazole Methylene Blue Omeprazole | Amodiaquine Atovaquone Chalcones Clindamycin DBB DEAQ Doxycycline Mefloquine Methylene Blue Pyronaridine Quinine Retinol + Mefloquine Triclosan | Atorvastatin N-Acetylcysteine Pyronaridine (Artesunate) Piperaquine (DHA) Rosuvastatin |
Amodiaquine and DEAQ | Chloroquine Methylene Blue | Artemisinins Atorvastatin Quinine Retinol | ||
Atovaquone | Chloroquine Mefloquine Methylene Blue Quinine | Cepharanthine | Amodiaquine Artemisinins Cepharanthine DEAQ Proguanil Retinol Tetracycline | |
Chloroquine | Amodiaquine Artemisinins Atorvastatin Mefloquine Methylene Blue Omeprazole Quinine Suladoxine-Pyrimethamine | Artemisnins Azithromycin Cepharanthine DECQ Methylene Blue | Azithromycin Cepharanthine Retinol | Piperaquine |
Lumefantrine | Cepharanthine | Cepharanthine DBB | ||
Mefloquine | Atorvastatin Cepharanthine Chloroquine | Methylene Blue | Artemisinins Methylene Blue Retinol | Piperaquine |
Piperaquine | Cepharanthine | Cepharanthine | Quinine Chloroquine DHA Mefloquine | |
Primaquine | Azithromycin | Azithromycin | ||
Proguanil | Atovaquone DBB | |||
Quinidine/Quinine | Atorvastatin Chloroquine | Azithromycin Methylene Blue | Amodiaquine Artemisinins Azithromycin DEAQ Omeprazole Methylene Blue Retinol | Piperaquine |
Sulfadoxine-Pyrimethamine | Chloroquine Methylene Blue |
Early pharmacodynamic studies were important for the advent of ACTs to treat malaria. Gupta et al. (2002a) assessed in vitro interactions of artemisinin with atovaquone, quinine, and mefloquine against P. falciparum. Findings were important, as synergism was shown with quinine and mefloquine, while additive activity to synergism was demonstrated with the atovaquone combination. The authors discussed how potential synergism may be questioned due to the rapid action and half-life of artemisinin but hypothesized that with appropriate timing the synergistic effects can be maximized. These findings, along with the others reported below, laid the foundation for the antimalarial combinations we see today.
Knauer et al. (2008) assessed in vitro interactions of quinine compared with retinol. Parasite isolates of P. falciparum were taken from patients with malaria contracted in Myanmar or Thailand. Thirty-eight isolates were successfully tested. Retinol significantly enhanced the activity of quinine; EC90 (concentration that leads to 90 % of maximal response) values with quinine-retinol were 829 nM, 738 nM, and 762 nM for low, medium, and high concentrations, respectively. This was also reflected by strong reductions in the geometric mean concentration for full inhibition (GMCOC) to 1,990, 1,462, 1,344 nM, respectively; all were deemed to be clinically achievable levels. Results from this study demonstrate that retinol is a potential candidate for future antimalarial combinations and should be assessed in animal and human studies.
Skinner-Adams and Davis (1999) assessed quinine, chloroquine, and artemisinin drugs for in vitro activity with omeprazole. Omeprazole was previously identified as a potential antimalarial agent. Combinations of quinine with omeprazole were found to be synergistic (interaction factor = 0.622, p < 0.001). However, omeprazole and chloroquine were antagonistic (interaction factor = −0.730, p < 0.001). Omeprazole combined with artemisinin drugs (artemisinin, dihydroartemisinin, artesunate, artemether) was found to exhibit additive interactions only. An antagonistic interaction was also found between quinine and chloroquine (interaction factor = −2.836, p = 0.005). The authors concluded that omeprazole could be a potential antimalarial agent. However, due to the limited activity and widespread use of proton-pump inhibitors, this is unlikely to occur.
Kerschbaumer et al. (2010) assessed mefloquine and artemisinin, in addition to enhancement with retinol. Forty-three P. falciparum isolates were taken from patients in Thailand. These isolates were tested for response to retinol alone, mefloquine alone, artemisinin alone, mefloquine-artemisinin 5:1, and mefloquine-artemisinin 5:1 with fixed concentrations of retinol corresponding to the 50th, 65th, and 80th percentile of the mean concentrations found in blood of healthy adults. Full inhibition of parasite maturation was found at concentrations of 38,205.5 nM (mefloquine) and 2,765.8 nM (artemisinin); and for the combination, concentrations were 11,124.0 nM (mefloquine) and 111.2 nM (artemisinin). Retinol further enhanced this synergistic finding (concentrations of 5,412 nM [mefloquine] and 54.2 nM [artemisinin] for low; 4,136.0 nM [mefloquine] and 41.4 nM [artemisinin] for medium; and 3638.0 nM [mefloquine] and 3.4 nM [artemisinin] for high). Again, retinol appeared to be a potential agent to be used in combination for treatment of malaria.
Gruber et al. (2009) evaluated the interaction between mefloquine and retinol after observing synergism with quinine. Thirty-seven isolates of P. falciparum were obtained from Thai patients. Concentrations of retinol at the 50th, 65th, and 80th percentiles of physiological levels were studied. The mean IC50, IC90, and IC99 (inhibitory concentrations at 50, 90, and 99 %) values for mefloquine were 1.76, 9.81 and 39.78 μM, respectively, for mefloquine alone; 0.33, 1.37, 4.33 μM for low retinol concentrations; 0.29, 1.15, and 3.48 μM for medium concentrations; and 0.20, 0.85, and 2.70 μM for high concentrations. The versatility of retinol makes it a leading candidate for new antimalarial combinations.
Ley et al. (2008) assessed synergy with chloroquine or amodiaquine and retinol. Twenty-nine isolates of P. falciparum were obtained from patients in Thailand. Synergism was found with chloroquine; however, this combination is clinically irrelevant due to resistance patterns of P. falciparum to chloroquine. Synergy was also demonstrated with amodiaquine. The GMCOC decreased from 2,520 nM with amodiaquine alone to 1,092, 800, and 745 nM with low, medium, and high concentrations of retinol, respectively. Similar trends were observed with EC50, EC90, and EC99. As above, evidence is growing for the potential use of retinol in combination regimens.
Mariga et al. (2005) evaluated in vitro pharmacodynamic interactions with amodiaquine and its metabolite desethylamodiaquine with artemisinin, quinine, and atovaquone. The three strains of P. falciparum originated from Tanzania, Gambia, and Thailand. Synergism (based on EC90) was found between all combinations but most commonly with atovaquone and artemisinin. Some antagonism was found between the other agents but this was mostly strain-specific and synergism was also consistently demonstrated. Findings give promising results that amodiaquine may be combined with agents outside of the artemisinin class, which may be an important consideration for emergence of future resistance patterns to this class.
In vitro atovaquone pharmacodynamic interactions have been described in a number of studies. Canfield et al. (1995) compared multiple antimalarial combinations for potential therapy to enhance efficacy. Findings included antagonistic interactions between atovaquone and the quinolones and artemisinins with synergism established with biguanides and tetracycline. Proguanil emerged as the leading candidate for the combination regimen. A second study by Lutgendorf et al. (2006) compared atovaquone plus proguanil in addition to artemisinin. This study reported synergism between atovaquone and artemisinin alone; but, synergism was more pronounced when proguanil was added. The authors concluded that this triple combination may be considered for a future clinical treatment regimen. In order to further understand the mechanism of interaction between atovaquone and proguanil, a third study by Thapar et al. (2003) evaluated combinations of each. Based on the achieved EC50 and EC90 values, it was determined that the synergism was due to atovaquone and proguanil and may not require the presence of cycloguanil. This is likely due to differences in targets of proguanil and cycloguanil.
Retinol has also been studied with atovaquone in a study by Exner et al. (2007). The EC90 values were lower with the combination therapy at low, medium, and high concentrations. Additionally, the GMCOCs were also lower (p < 0.05). Therefore, the authors concluded that retinol may be used to enhance the antimalarial activity of atovaquone, which is in line with previously reported studies that describe synergistic interactions of retinol with antimalarial agents.
Stahel et al. (1988) evaluated chloroquine and its active metabolite desethylchloroquine with the antimalarial drugs, quinine, amodiaquine, mefloquine, pyrimethamine-sulfadoxine, and artemisinin. Findings showed an additive interaction between chloroquine and desethylchloroquine but antagonistic interactions with all the other combinations. The authors concluded that decreased therapeutic efficacy may be a consequence of chloroquine being administered with or around doses of other antimalarials. For these reasons, as well as chloroquine resistance worldwide, it is not recommended as part of first-line combinations for P. falciparum.
Kyavar et al. (2006) assessed chloroquine with artemisinin and desbutyl-benflumetol (DBB) for P. vivax. Although not a typical combination, interaction studies found an additive interaction when chloroquine was combined with artemisinin at EC50, EC90, and EC99 values. The DBB combination with artemisinin revealed a significant activity correlation at EC50, EC90, and EC99, demonstrating both additive and synergistic (EC99) interactions. The authors concluded that this combination may be a potential therapeutic alternative for falciparum and vivax malaria. Further studies are needed to confirm in vivo.
Pereira et al. (2011) assessed azithromycin, purported to have antimalarial properties, in combination with chloroquine for chloroquine-sensitive P. falciparum in patients in Malawi. Results showed mostly additive interactions for in vitro samples at 96 h. However, at EC90 values, synergy was apparent. The authors also tested the combination in addition to amlodipine in an in vivo mouse model and found 99.9 % of parasitemia suppressed. Amlodipine is known to have resistance reversal properties when used in combination with chloroquine. However, pharmacokinetic/pharmacodynamic modeling suggested that a dose of 1.8 g of amlodipine would be needed to achieve similar efficacy in humans and this would likely not be achieved due to its adverse effect profile. Although azithromycin shows promise, studies are needed to determine true efficacy and resistance profile of this agent.
Bwijo et al. (1997) evaluated the combination of artemisinin and mefloquine in vitro. Chloroquine-sensitive strains of P. falciparum were used under repetitive dosing to mimic in vivo conditions. The period of drug dosing was 3 days. Findings showed EC50, 90, and 99 values were significantly lower for both artemisinin and mefloquine when used in combination and produced synergy at concentrations normally reached in vivo (p = 0.016). The findings of this study helped support the development of mefloquine as a component in combination therapy.
Arreesrisom et al. (2007) assessed the effect of N-acetylcysteine (NAC) on the anti-P. falciparum activity of artesunate. NAC may have antimalarial properties and be a candidate for adjunctive treatment. Interestingly, inhibition of the antimalarial activity of artesunate was observed during the first 6 h and when NAC was pre-incubated with P. falciparum. However, no inhibition was noted when NAC was added 2 h after parasite exposure to artesunate. Although positive, this combination is unlikely to add value for malaria treatment.
Piperaquine is a newer antimalarial agent currently recommended in combination with dihydroartemisinin. Davis et al. (2006) aimed to assess in vitro interactions with piperaquine, pyronaridine, naphthoquine with DHA, quinine, mefloquine, and chloroquine. Results found no interaction or only mild antagonism with all combinations. Findings suggested that the clinical significance of any observed antagonism is unknown but likely to be minimal.
Two studies assessed the antimalarial activity of methylene blue in combination with other agents. Methylene blue was formerly used as an antimalarial but research is being conducted to determine its appropriateness for future combination regimens. Garavito et al. (2007) assessed the activity of methylene blue for P. falciparum in combination with amodiaquine, artemether, atovaquone, chloroquine, doxycycline, mefloquine, primaquine, pyrimethamine, and quinine. Findings showed antagonism with amodiaquine, atovaquone, doxycycline, and pyrimethamine; additive behavior with artemether, chloroquine, mefloquine, and primaquine; and synergy with quinine.
Dormoi et al. (2012) assessed methylene blue in combination with chloroquine, monodesethylamodiaquine, quinine, mefloquine, dihydroartemisinin, and atorvastatin for P. falciparum. Findings showed antagonism with chloroquine, additive effects with monodesethylamodiaquine, and synergistic effects with mefloquine and quinine. High synergism was noted with dihydroartemisinin and atorvastatin. These findings suggest that methylene blue could become a new target agent for future antimalarial combination regimens.
Ohrt et al. (2002) assessed in vitro outcomes of azithromycin in combination with multiple agents against P. falciparum. Studies with chloroquine demonstrated additive and synergistic interactions while quinine, tafenoquine, and primaquine were additive to synergistic. Dihydroartemisinin was additive but trended toward antagonism. Findings suggest that chloroquine-azithromycin may be considered for prophylaxis, while quinine-azithromycin has the potential for malaria treatment. Noedl et al. (2007) assessed azithromycin in combination with dihydroartemisinin or quinine. Findings showed azithromycin to have significant antimalarial activity and when combined with either agent, demonstrated additive (trending toward synergistic) interactions. Again, more research is needed with this agent, especially to consider potential for resistance.
Studies have assessed the interaction between artemisinin and monodebutyl-benflumetol. Muller et al. (2008) assessed this combination in a 1:1 M/M ratio. Interaction studies showed moderate synergism at EC50 and strong synergism at EC90 and EC99. The positive interaction was most pronounced in isolates with reduced sensitivity against artemisinin and monodebutyl-benflumetol. Another study by Raffelsberger et al. (2008) assessed the combination with P. falciparum in a 1:3 M/M ratio. Synergism was found between these two agents but became less evident after subsequent analysis. This study also assessed monodebutyl-benflumetol and proguanil in combination. Moderate synergism was found that may be beneficial for future therapeutic use. Findings from these studies warrant in vivo analysis to assess the efficacy and safety of these agents in combination.
Clindamycin has been purported to have antimalarial properties. Ramharter et al. (2003) assessed the combination of clindamycin with dihydroartemisinin in P. falciparum isolates. Interaction studies showed additive or synergistic interactions at various concentration ratios (e.g. EC50). No antagonism was identified. A fixed combination showed additive activity at EC90 values and the authors concluded that this combination may be a potential candidate for clinical use. Clindamycin is now recommended as part of second-line combinations for some indications (Sect. 7.1).
Vivas et al. (2008) evaluated the efficacy of pyronaridine and artesunate. In vitro studies showed slight antagonism with P. falciparum but this was deemed to be negligible. In vivo studies of P. berghei found increased activity when the agents were used in combination, suggesting additive or synergistic interactions. This combination should be further explored in clinical settings.
As statin agents have been purported to have antimalarial activity, Wong and Davis (2009) assessed atorvastatin and rosuvastatin in combination with chloroquine and dihydroartemisinin. Results showed no beneficial interactions and authors deemed any antimalarial activity present was not sufficient to warrant further study of these drugs as potential therapeutic agents. Based on these findings, it is unlikely that statins will be further assessed as antimalarials.
Cepharanthine is an alkaloid isolated from the plant Stephania rotunda. Desgrouas et al. (2014) completed interaction studies to assess the potential of this agent as a component of an antimalarial combination. In vitro testing showed enhanced efficacy with chloroquine, lumefantrine, atovaquone, piperaquine, and monodesethylamodiaquine. However, antagonism was demonstrated with dihydroqrtemisinin and mefloquine. In vivo results showed improved survival of mice when cepharanthine was used in combination with chloroquine or amodiaquine. These findings warrant future study with this agent as part of antimalarial combination therapy.
Leeb et al. (2010) assessed the interaction between lumefantrine and monodesbutyl-benflumetol in 44 isolates of P. falciparum. Geometric mean values for complete inhibition of schizont maturation were 1036 nM for lumefantrine, 655 nM for monodesbutyl-benflumetol and 223 nM for the combination. Moderate synergism was found at the IC50 and increased to the highest level at IC99. The authors concluded that this combination may be suitable for future use pending results from clinical trials. This is consistent with other studies that also assessed the utility of desbutyl-benflumetol (Kyavar et al. 2006).
Starzengruber et al. (2008) assessed the same agents in 35 isolates of P. falciparum. Results were very similar giving GMCOC values of 537 nM for lumefantrine, 246 nM for monodesbutyl-benflumetol, 236 nM for lumefantrine-monodesbutyl-benflumetol 999:1, and 155 nM for lumefantrine-monodesbutyl-benflumetol 995:5. For the 995:5 combination, synergism was found and increased with effective inhibitory concentrations. These findings further support the development of this drug as an antimalarial combination agent.
Tripathi et al. (2013) attempted to use pharmacokinetic principles to identify a new combination option. Ketoconazole, a potent CYP3A4 inhibitor, was combined with a/B arteether in vitro against P. falciparum. Findings showed an additive interaction. The study was taken further with an in vivo analysis using mice and multidrug-resistant P. yoelii nigeriensis. Results showed that sub-curative doses of ketoconazole combined with a/B arteether achieved 100 % curative action. While the exact mechanism of action is unknown, the authors speculated that the pharmacokinetic properties of ketoconazole may contribute to these findings.
Ketoconazole was also assessed by Mishra et al. (2007) with artemisinin. Interactions between artemisinin and ketoconazole as well as triclosan were evaluated in cultures of P. falciparum. Ketoconazole was found to be antagonistic in vitro. However, triclosan showed mild synergism. The authors stated that no firm conclusions can be made regarding ketoconazole until the combination is tested in vivo. Although there were discrepant findings between this study and those of Tripathi et al. (2013), further testing can be justified to develop ketoconazole as a potential antimalarial combination agent.
Sponer et al. (2002) assessed the pharmacodynamic interactions between doxycycline, a known antimalarial agent, and artemisinin against 31 fresh isolates of P. falciparum. Findings suggested a synergistic interaction at each of the EC50, EC90, and EC99 values. The authors acknowledged that clinical trials with these agents have yielded inconclusive results but their findings suggest a potential therapeutic benefit of this combination. Doxycycline is currently recommended as a prophylaxis agent but not as first-line for treatment.
Bhattacharya et al. (2008) evaluated the pharmacodynamic interaction between amphotericin B or clotrimazole with artemisinin against P. falciparum in vitro. Findings showed additive interactions for both agents. These interactions occurred at therapeutically safe concentrations. These agents were also active at different stages of the lifecycle as compared to artemisinin. Authors hypothesized that by aiming for different molecular targets, therapeutic efficacy may be enhanced without development of resistance. This information is useful for development of these agents as well as other drug combinations.
The same authors (Bhattacharya et al. 2009) studied the pharmacodynamics of chalcone derivatives in combination with artemisinin against P. falciparum in vitro. Chalcones are aromatic ketones and form a group of natural compounds that are easy to synthesize. Licochalcone A was previously reported to have antimalarial activity. When assessed in combination with artemisinin, these derivatives showed synergistic or additive interactions. Thus, this group of compounds may have potential for future drug development against malaria.
Gupta et al. (2002b) studied a synergistic pharmacodynamic interaction between artemisinin and amodiaquine. Combinations of artemisinin with amodiaquine, pyronaridine, and chloroquine were tested in three strains (2 chloroquine-sensitive, one chloroquine-resistant) of P. falciparum. Findings showed synergism between artemisinin, amodiaquine and pyronaridine. However, chloroquine showed only additive properties. The authors concluded that amodiaquine may be suitable for combination therapy with artemisinin. Artesunate-amodiaquine is now recommended as a first-line combination.
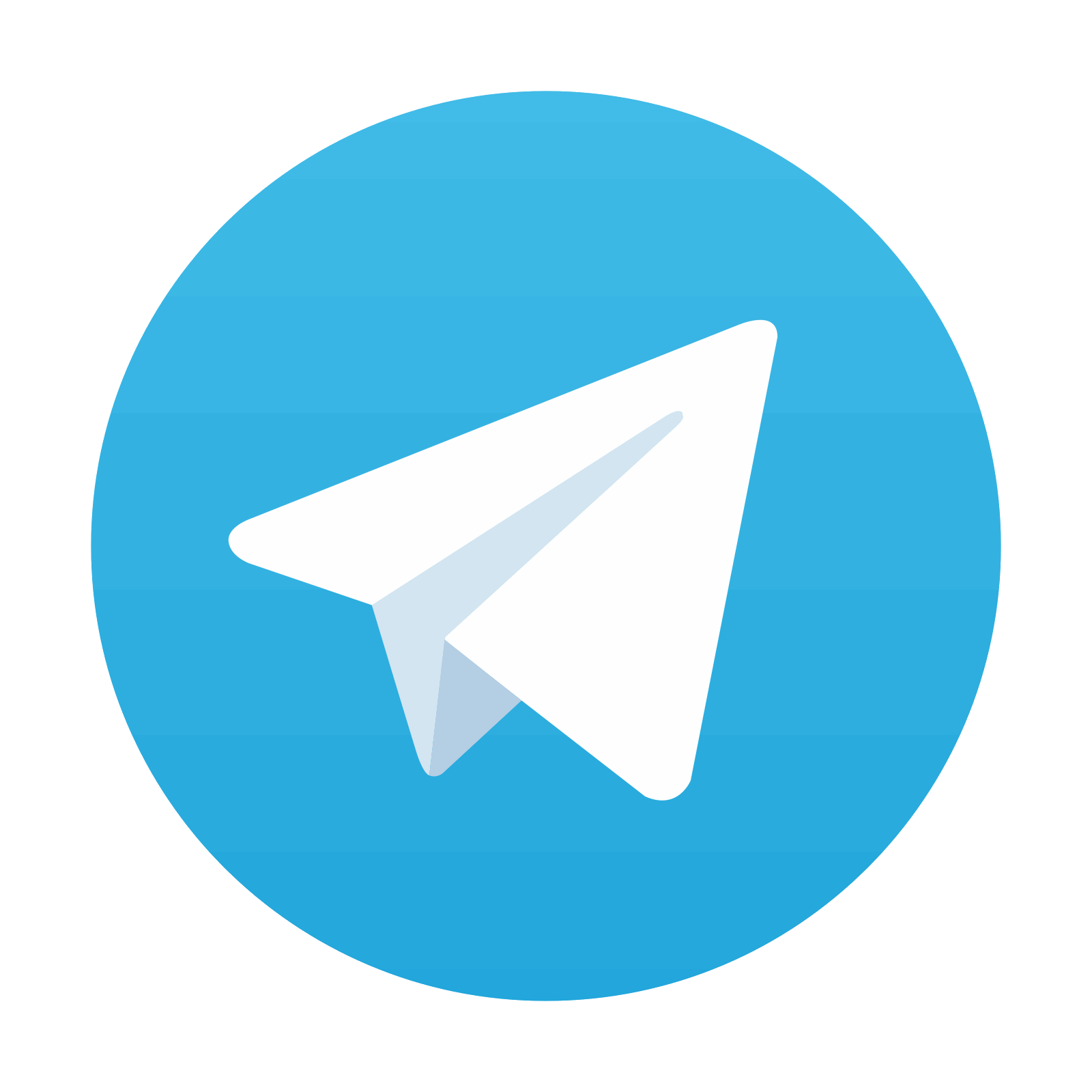
Stay updated, free articles. Join our Telegram channel

Full access? Get Clinical Tree
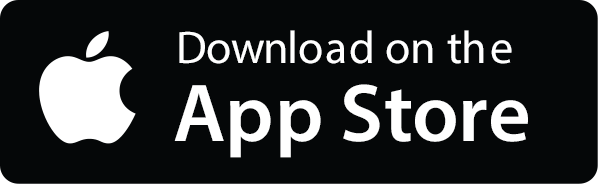
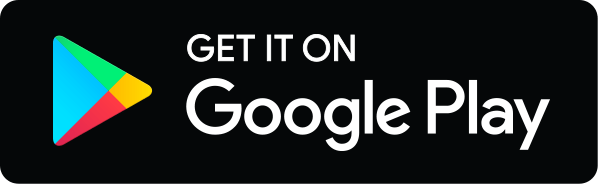