where [HA] is the concentration of un-ionized acid, [A−] is the concentration of ionized base, [B] is the concentration of un-ionized base, and [BH+] is the concentration of ionized base. Although pH partition theory is useful, it often does not hold true. For example, most weak acids are well absorbed from the small intestine, which is contrary to the prediction of the pH partition hypothesis. Similarly, quaternary ammonium compounds are ionized at all pH levels but are readily absorbed from the GI tract. These discrepancies arise because pH partition theory does not take into consideration the following factors, among others:
■ Large epithelial surface areas of the small intestine compensate for ionization effects.
■ Long residence time in the small intestine also compensates for ionization effects.
■ Charged drugs, such as quaternary ammonium compounds and tetracyclines, may interact with opposite-charged organic ions, resulting in a neutral species that is absorbable.
■ Some drugs are absorbed by means of active transport.
Solubility and the Noyes–Whitney Equation of Dissolution
Drug solubility is the maximum amount of the drug that is soluble in unit volume of the solvent. For most drugs, the rate at which the solid drug dissolves in a solvent (dissolution) is often the rate-limiting step in the drug’s bioavailability. The rate at which a solid drug of limited water solubility dissolves in a solvent can be determined using the Noyes–Whitney equation:
where dM/dt is the rate of dissolution (in mass/time), k is the dissolution rate constant (in cm/s) (k = D/h), S is the surface area of exposed solid (in cm2), D is the diffusion coefficient of solute in solution (in cm2/s), h is the thickness of the diffusion layer (in cm), Cs is the drug solubility (in mass/mL), and C is the drug concentration in bulk solution at time t (in mass/mL).
Under sink conditions, when C is much less than Cs, the Noyes–Whitney equation can be simplified as follows:
where dC/dt is the dissolution rate (in concentration/time) and V is the volume of the dissolution medium (in mL).
The following factors influence the dissolution rate:
■ The conditions in the GI tract affect the dissolution rate. For example, the presence of foods that increase the viscosity of GI fluids decreases the diffusion coefficient, D, of a drug and its dissolution rate.
■ The thickness of the diffusion layer, h, is influenced by the degree of agitation experienced by each drug particle in the GI tract. Hence, an increase in gastric or intestinal motility may increase the dissolution rate of poorly soluble drugs.
■ The dissolution rate of a weakly acidic drug in GI fluids is influenced by the drug solubility in the diffusion layer surrounding each dissolving drug particle. The pH of the diffusion layer significantly affects the solubility of a drug and its subsequent dissolution rate. The dissolution rate of a weakly acidic drug in GI fluid (pH 1–3) is relatively low because of its low solubility in the diffusion layer. If the pH in the diffusion layer could be increased, the solubility (Cs) exhibited by the weak acidic drug in this layer (and hence the dissolution rate of the drug in GI fluids) could be increased. The potassium or sodium salt form of the weakly acidic drug has a relatively high solubility at the elevated pH in the diffusion layer. Thus, the dissolution of the drug particles takes place at a faster rate. The reverse is true for weakly basic drugs; a reduction in pH of the diffusion layer would increase its solubility in the GI tract.
■ Particle size and the surface area of the drug significantly influence the drug dissolution rate. An increase in the total effective surface area of the drug in contact with GI fluids causes an increase in its dissolution rate. The smaller the particle size is, the greater will be the effective surface area exhibited by a given mass of drug and the higher will be the dissolution rate. However, particle size reduction is not always helpful and may fail to increase the bioavailability of a drug. In the case of certain hydrophobic drugs, excessive particle size reduction tends to cause aggregation into larger particles. Preventing the formation of aggregates requires the dispersion of small drug particles in polyethylene glycol (PEG), polyvinylpyrrolidone (PVP), dextrose, or other agents. For example, a dispersion of griseofulvin in PEG 4,000 enhances its dissolution rate and bioavailability. Certain drugs, such as penicillin G and erythromycin, are unstable in gastric fluids and do not dissolve readily in them. For such drugs, particle size reduction yields an increased rate of drug dissolution in gastric fluid and also increases the extent of drug degradation.
■ Amorphous or noncrystalline forms of a drug may have faster dissolution rates than crystalline forms.
■ Surface-active agents will increase dissolution rates by lowering interfacial tension, which allows better wetting and penetration by the solvent. Weakly acidic and basic drugs may be brought into solution by the solubilizing action of surfactants.
Rheology
Rheology is the study of flow properties of liquids under the influence of stress. The flow of simple liquids can be described by viscosity, an expression of the resistance to flow. Liquids are divided into two general categories, Newtonian and non-Newtonian, depending on their characteristics. Rheological properties are useful for the formulation and analysis of emulsions, suspensions, and lotions. Pourability, spreadability, and syringeability of an emulsion or a suspension are determined by its rheological properties.
According to Newton’s law of viscous flow, the rate of flow (D) is directly proportional to the applied stress (τ). That is, τ = η • D, where η is the viscosity. Fluids that obey Newton’s law of flow are referred to as Newtonian fluids, and fluids that deviate are known as non-Newtonian fluids. The force per unit area (F′/A) required to bring about flow is called the shearing stress (F):
where η is the viscosity, dv/dr is the rate of shear = G (s−1), and F′/A units are in dynes per cm2. For simple Newtonian fluids, a plot of the rate of shear against shearing stress gives a straight line (Figure 3-1A); thus, η is a constant. In the case of Newtonian fluids, viscosity does not change with increasing shear rate. Various types of water and pharmaceutical dosage forms that contain a high percentage of water are examples of liquid dosage forms that have Newtonian flow properties.
Most pharmaceutical fluids (including colloidal dispersions, emulsions, and liquid suspensions) do not follow Newton’s law of flow, and the viscosity of the fluid varies with the rate of shear. There are three types of non-Newtonian flow: plastic, pseudoplastic, and dilatant (Figure 3-1B, C, and D).
Plastic flow
Fluids that undergo plastic flow are called Bingham bodies; these materials are defined as fluids that exhibit a yield value (Figure 3-1B). Plastic flow is associated with the presence of flocculated particles in concentrated suspensions. Flocculated solids are light, fluffy conglomerates of adjacent particles held together by weak van der Waals forces. The yield value exists because a certain shearing stress must be exceeded to break up van der Waals forces. A plastic system resembles a Newtonian system at shear stresses above the yield value. Yield value, f, is an indicator of flocculation (the higher the yield value, the greater the degree of flocculation). The characteristics of plastic flow materials can be summarized as follows:
■ Plastic flow does not begin until a shearing stress, corresponding to a yield value, f, is exceeded.
■ The curve intersects the shearing stress axis but does not cross through the origin.
■ The materials are said to be “elastic” at shear stresses below the yield value.
■ Viscosity decreases with increasing shear rate at shear stress below the yield value.
Pseudoplastic flow
Pseudoplastic flow is exhibited by polymers in solution. A large number of pharmaceutical products, including natural and synthetic gums (e.g., liquid dispersions of tragacanth, sodium alginate, methyl cellulose, and sodium carboxymethylcellulose), exhibit pseudoplastic flow properties. The characteristics of pseudoplastic flow materials can be summarized as follows:
Figure 3-1. Plots of Rate of Shear as a Function of Shearing Stress for (A) Newtonian, (B) Plastic, (C) Pseudoplastic, (D) Dilatant, and (E) Thixotropic Flow
■ Pseudoplastic substances begin to flow when a shearing stress is applied: that is, there is no yield value (it does cross the origin).
■ The viscosity of a pseudoplastic substance decreases with increasing shear rate.
■ With increasing shearing stress, the rate of shear increases; these materials are called shear-thinning systems.
■ Shear thinning occurs when molecules (polymers) align themselves along their long axes and slip and slide past each other.
Dilatant flow
Certain suspensions with a high percentage of dispersed solids exhibit an increase in resistance to flow with increasing rates of shear. Dilatant systems are usually suspensions with a high percentage of dispersed solids that exhibit an increase in resistance to flow with increasing rates of shear. Dispersions containing a high percentage (≥ 50%) of small, deflocculated particles may exhibit this type of behavior. The characteristics of dilatant flow materials can be summarized as follows:
■ Dilatant materials increase in volume when sheared.
■ They are also known as shear-thickening systems (the opposite of pseudoplastic systems).
■ When the stress is removed, the dilatant system returns to its original state of fluidity.
■ Viscosity increases with increasing shear rate.
■ Dilatant materials may solidify under conditions of high shear.
Thixotropy
Thixotropy is a nonchemical isothermal gel–sol–gel transformation. If a thixotropic gel is sheared (by simple shaking), the weak bonds are broken, and a lyophobic solution is formed (Figure 3-1E). On standing, the particles collide, leading to flocculation and formation of gel. Thixotropy is a desirable property in liquid pharmaceutical preparations. The advantage associated with thixotropic preparations is that the particles remain in suspension during storage, but when required for use, the gels are readily made fluid by tapping or shaking. The shearing force on the injection as it is pushed through the needle ensures that it is fluid when injected; however, the rapid resumption of the gel structure prevents excessive spreading in the tissues, and consequently a more compact depot is produced than with nonthixotropic suspensions. A well-formulated thixotropic suspension will not settle out readily in the container and will become fluid on shaking. Flow curves (rheograms) for thixotropic materials are highly dependent on the rate at which shear is increased or decreased and the length of time a sample is subjected to any one rate of shear.
Negative thixotropy
Negative thixotropy is also known as antithixotropy, which represents an increase rather than a decrease in consistency on the down curve (an increase in thickness or resistance to flow with an increased time of shear). It may result from an increased collision frequency of dispersed particles (or polymer molecules) in suspension, which causes increased interparticle bonding with time.
3-4. Surfactants and Micelles
Surface-active agents, or surfactants, are substances that adsorb to surfaces or interfaces to reduce surface or interfacial tension. They may be used as emulsifying agents, solubilizing agents, detergents, and wetting agents. Surfactants have two distinct regions in one chemical structure. One area is hydrophilic (water loving); another is hydrophobic (water repelling). The existence of two such moieties in a molecule is known as amphipathy, and the molecules are consequently referred to as amphipathic molecules or amphiphiles. Depending on the number and nature of the polar and nonpolar groups present, the amphiphile may be predominantly hydrophilic, lipophilic, or somewhere in between. For example, alkyl chain alcohols, amines, and acids are amphiphiles that change from being predominantly hydrophilic to lipophilic as the number of carbon atoms in the alkyl chain is increased. The hydrophobic portions are usually saturated or unsaturated hydrocarbon chains or, less commonly, heterocyclic or aromatic ring systems.
Surfactants are classified according to the nature of the hydrophilic or hydrophobic groups. In addition, some surfactants possess both positively and negatively charged groups and can exist as either anionic or cationic, depending on the pH of the solution. These surfactants are known as ampholytic compounds.
At low concentrations in solutions, amphiphiles exist as monomers. As the concentration is increased, aggregation occurs over a narrow concentration range. These aggregates, which may contain 50 or more monomers, are called micelles. Therefore, micelles are small spherical structures composed of both hydrophilic and hydrophobic regions. The polar head groups of the surfactant molecules are arranged in an outer shell while their hydrocarbon chains are oriented toward the center, forming a hydrophobic core. The concentration of monomer at which micelles are formed is called the critical micellization concentration, or CMC. Surface tension decreases up to the CMC but remains constant above the CMC. The longer the hydrophobic chain or the lower the polarity of the polar group, the greater the tendency for monomers to “escape” from the water to form micelles and hence lower the CMC.
Types of Micelles
In the case of amphiphiles in water, in dilute solution (still above but close to the CMC), the micelles are considered to be spherical in shape. At higher concentrations, they become more asymmetric and eventually assume cylindrical or lamellar structures. Oil-soluble surfactants have a tendency to self-associate into reverse micelles in nonpolar solvents, with their polar groups oriented away from the solvent.
Factors Affecting CMC and Micellar Size
■ Structure of hydrophobic group: An increase in the hydrocarbon chain length causes a logarithmic decrease in the CMC.
■ Nature of hydrophilic group: An increase in chain length increases hydrophilicity and the CMC. In general, nonionic surfactants have very low CMC values and high aggregation numbers compared with their ionic counterparts with similar hydrocarbon chains.
■ Nature of counterions: Note that Cl− < Br− < I− for cationic surfactants, and Na+ < K+ for anionic surfactants.
■ Electrolytes: The addition of electrolytes to ionic surfactants decreases the CMC and increases the micellar size. In contrast, micellar properties of nonionic surfactants are affected only minimally by the addition of electrolytes.
■ Temperature: At temperatures up to the cloud point, an increase in micellar size and a decrease in CMC is noted for many nonionic surfactants but has little effect on that of ionic surfactants.
■ Alcohol: CMCs are increased by the addition of alcohols.
Hydrophilic–Lipophilic Balance Systems
Griffin’s method of selecting emulsifying agents is based on the balance between the hydrophilic and lipophilic portions of the emulsifying agent, now widely known as the hydrophilic–lipophilic balance (HLB) system. The higher the HLB value of an emulsifying agent, the more hydrophilic it is. The emulsifying agents with lower HLB values are less polar and more lipophilic. The Spans (i.e., sorbitan esters) are lipophilic and have low HLB values (1.8–8.6); the Tweens (polyoxyethylene derivatives of the Spans) are hydrophilic and have high HLB values (9.6–16.7). Surfactants with the proper balance of hydrophilic and lipophilic affinities are effective emulsifying agents because they concentrate at the oil-in-water (o/w) interface. The type of an emulsion that is produced depends primarily on the property of the emulsifying agent. The HLB of an emulsifier or a combination of emulsifiers determines whether an o/w or water-in-oil (w/o) emulsion results. In general, o/w emulsions are formed when the HLB of the emulsifier is within the range of about 9 to 12; w/o emulsions are formed when the range is about 3 to 6. The type of emulsion is a function of the relative solubility of the supernatant. An emulsifying agent with high HLB is preferentially soluble in water and results in the formation of an o/w emulsion. The reverse situation is true with surfactants of low HLB value, which tend to form w/o emulsions.
Micellar Solubilization
Micelles can be used to increase the solubility of materials that are normally insoluble or poorly soluble in the dispersion medium used. The interior of micelles is composed of a hydrophobic core in which compounds that are poorly soluble in the dispersion medium can be dissolved. For example, surfactants are often used to increase the solubility of poorly soluble steroids. The factors affecting micellar solubilization are the nature of surfactants, the nature of solubilizates, and the temperature.
3-5. Dispersed Systems
Dispersed systems consist of particulate matter, known as the dispersed phase, distributed throughout a continuous or dispersion medium. The particulate matter, or dispersed phase, consists of particles that range from 1 nanometer (nm) to 0.5 micrometer (10−9 m to 5 × 10−7 m). Depending on the dispersed phase, dispersed systems are classified as follows:
■ Molecular dispersions: Less than 1 nm, invisible under electron microscopy. Examples are oxygen molecules, ions, and glucose.
■ Colloidal dispersions: From 1 nm to 0.5 micrometer, visible under electron microscopy. Examples are colloidal silver sols and natural and synthetic polymers.
■ Coarse dispersions: Greater than 0.5 micrometer, visible under light microscopy. Examples are grains of sand, activated charcoal, emulsions, suspensions, and red blood cells.
Types of Colloidal Systems
On the basis of the interaction of the particles, molecules, or ions of the dispersed phase with the molecules of dispersion medium, colloidal systems are classified into three groups: lyophilic, lyophobic, and association colloids.
Lyophilic or hydrophilic colloids
Systems containing colloidal particles that interact with the dispersion medium are referred to as lyophilic colloids. In the case of lipophilic colloids, organic solvent is the dispersion medium, whereas water is used as the dispersion medium for hydrophilic colloids. Because of their affinity for the dispersion medium, such materials form colloidal dispersions with relative ease. For example, the dissolution of acacia or gelatin in water, or celluloid in amyl acetate, leads to the formation of a solution. Most lyophilic colloids are polymers (e.g., gelatin, acacia, povidone, albumin, rubber, and polystyrene).
Lyophobic or hydrophobic colloids
Lyophobic colloids are composed of materials that have little attraction for the dispersion medium. Lyophobic colloids are intrinsically unstable and irreversible. Hydrophobic colloids are generally composed of inorganic particles dispersed in water.
Association colloids
Association colloids (referring to amphiphilic colloids) are formed by the grouping or association of amphiphiles (i.e., molecules that exhibit both lyophilic and lyophobic properties). At low concentrations, amphiphiles exist separately and do not form a colloid. At higher concentrations, aggregation occurs at around 50 or more monomers, leading to micelle formation. As with lyophilic colloids, formation of association colloids is spontaneous if the concentration of the amphiphile in solution exceeds the CMC.
Zeta Potential and Its Effect on Colloidal Stability
Zeta (ζ) potential is defined as the difference in potential between the surface of the tightly bound layer (shear plane) and the electroneutral region of the solution. The zeta potential governs the degree of repulsion between adjacent, similarly charged, dispersed particles. If the zeta potential is reduced below a certain value, the attractive forces exceed the repulsive forces, and the particles come together. This phenomenon is known as flocculation.
Stabilization is accomplished by providing the dispersed particles with an electric charge and a protective solvent sheath surrounding each particle to prevent mutual adherence attributable to collision. This second effect is significant only in the case of lyophilic colloids. Lyophilic and association colloids are thermodynamically stable and exist in a true solution so that the system constitutes a single phase. In contrast, lyophobic colloids are thermodynamically unstable but can be stabilized by preventing aggregation or coagulation by providing the dispersed particles with an electric charge, which can prevent coagulation through repulsion of like particles.
3-6. Pharmaceutical Ingredients
Turning a drug into a pharmaceutical dosage form or a drug delivery system requires pharmaceutical ingredients. For example, in the preparation of tablets, the addition of diluents or fillers increases the bulk of the formulation. Binders are added to promote adhesion of the powdered drug to other ingredients. Lubricants assist the smooth tableting process. Disintegrants promote tablet breakup after administration. Coatings improve stability, control disintegration, or enhance appearance. In the preparation of pharmaceutical solutions, preservatives are added to prevent microbial growth, stabilizers are added to prevent drug decomposition, and colorants and flavorants are added to ensure product appeal. Thus, for each dosage form, the pharmaceutical ingredients establish the primary features of the product and control the physicochemical properties, drug-release profiles, and bioavailability of the product. Table 3-1 lists some typical pharmaceutical ingredients used in different dosage forms.
Table 3-1. Typical Pharmaceutical Ingredients
3-7. Types of Commonly Used Dosage Forms
Solutions
Solutions are homogeneous mixtures of one or more solutes dispersed in a dissolving medium (solvent). Aqueous solutions containing a sugar or sugar substitute with or without added flavoring agents and drugs are classified as syrups. Sweetened hydroalcoholic (combinations of water and ethanol) solutions are termed elixirs. Hydroalcoholic solutions of aromatic materials are termed spirits. Tinctures are alcoholic or hydroalcoholic solutions of chemical or soluble constituents of vegetable drugs. Most tinctures are prepared by an extraction process. Mouthwashes are solutions used to cleanse the mouth or treat diseases of the oral membrane. Antibacterial topical solutions (e.g., benzalkonium chloride and strong iodine) will kill bacteria when applied to the skin or mucous membrane.
Solutions intended for oral administration usually contain flavorants and colorants to make the medication more attractive and palatable to the patient. They may contain stabilizers to maintain the physicochemical stability of the drug and preservatives to prevent the growth of microorganisms in the solution. A drug dissolved in an aqueous solution is in the solution; no dissolution step is necessary before systemic absorption occurs. Solutions that are prepared to be sterile, that are pyrogen free, and that are intended for parenteral administration are classified as injectables.
Some drugs, particularly certain antibiotics, have insufficient stability in aqueous solution to withstand long shelf lives. These drugs are formulated as dry powder or granule dosage forms for reconstitution with purified water immediately before dispensing to the patient. The dry powder mixture contains all of the formulation components—that is, drug, flavorant, colorant, buffers, and others—except for the solvent. Examples of dry powder mixtures intended for reconstitution to make oral solutions include cloxacillin sodium, nafcillin sodium, oxacillin sodium, and penicillin V potassium.
Sucrose is the sugar most frequently used in syrups; in special circumstances, it may be replaced in whole or in part by other sugars (e.g., dextrose) or nonsugars (e.g., sorbitol, glycerin, and propylene glycol). Most syrups consist of between 60% and 80% sucrose. Sucrose not only provides sweetness and viscosity to the solution, but also renders the solution inherently stable (unlike dilute sucrose solutions, which are unstable).
Compared with syrups, elixirs are usually less sweet and less viscous because they contain a lower proportion of sugar, and they are consequently less effective than syrups in masking the taste of drugs. In contrast to aqueous syrups, elixirs are better able to maintain both water-soluble and alcohol-soluble components in solution because of their hydroalcoholic properties. These stable characteristics often make elixirs preferable to syrups. All elixirs contain flavoring and coloring agents to enhance their palatability and appearance. Elixirs containing over 10% to 12% ethanol are usually self-preserving and do not require the addition of antimicrobial agents for preservation. Alcohols precipitate tragacanth, acacia, agar, and inorganic salts from aqueous solutions; therefore, such substances should either be absent from the aqueous phase or be present in such low concentrations as not to promote precipitation on standing. Examples of some commonly used elixirs include dexamethasone elixir U.S. Pharmacopeia (USP), pentobarbital elixir USP, diphenhydramine hydrochloride elixir, and digoxin elixir.
Tablets
Depending on the physicochemical properties of the drug, site and extent of drug absorption in the GI tract, stability to heat or moisture, biocompatibility with other ingredients, solubility, and dose, the following types of tablets are commonly formulated:
■ Swallowable tablets are intended to be swallowed whole and then disintegrate and release their medicaments in the GI tract.
■ Effervescent tablets are dissolved in water before administration. In addition to the drug substance, these tablets contain sodium bicarbonate and an organic acid such as tartaric acid. These additives react in the presence of water, liberating carbon dioxide, which acts as a disintegrator and produces effervescence.
■ Chewable tablets are used when a faster rate of dissolution or buccal absorption is desired. Chewable tablets consist of a mild effervescent drug complex dispersed throughout a gum base. The drug is released from the dosage form by physical disruption associated with chewing, chemical disruption caused by the interaction with the fluids in the oral cavity, and the presence of effervescent material. For example, antacid tablets should be chewed to obtain quick relief of indigestion.
■ Buccal and sublingual tablets dissolve slowly in the mouth, cheek pouch (buccal), or under the tongue (sublingual). Buccal or sublingual absorption is often desirable for drugs subject to extensive hepatic metabolism, often referred to as the first-pass effect. Examples are isoprenaline sulfate (a bronchodilator), glyceryl trinitrate (a vasodilator), nitroglycerin, and testosterone tablets. These tablets do not contain a disintegrant and are compressed lightly to produce a fairly soft tablet.
■ Lozenges are compressed tablets that do not contain a disintegrant. Some lozenges contain antiseptics (e.g., benzalkonium) or antibiotics for local effects in the mouth.
■ Controlled-release tablets are used to improve patient compliance and to reduce side effects. Some water-soluble drugs are formulated as sustained-release tablets so that their release and dissolution are controlled over a long period. The combination of high- and low-viscosity grades of hydroxypropyl methylcellulose (HPMC) was used as the matrix base to prepare zileuton sustained-release tablets. A ternary polymeric matrix system composed of protein, HPMC, and highly water-soluble drugs such as diltiazem hydrochloride was developed by the direct compression method. Sustained-release tablets can also be prepared by formulating inert polymers such as polyvinyl chloride, polyvinyl acetate, and methyl methacrylate. These polymers protect the tablet from disintegration and reduce the dissolution rate of the drug inside the tablet. Examples of commonly used sustained-release drug delivery products are listed in Table 3-2.
Table 3-2. Examples of Sustained-Release Drug Delivery Products
Boldface indicates one of top 100 drugs for 2012 by units sold at retail outlets, www.drugs.com/stats/top100/2012/units.
■ Coated tablets are used to prevent decomposition or to minimize the unpleasant taste of certain drugs. Several types of coated tablets are made: film coated, sugar coated, gelatin coated (gel caps), or enteric coated. Enteric coatings are resistant to gastric juices but readily dissolve in the small intestine. These enteric coatings can protect drugs against decomposition in the acidic environment of the stomach. Commonly used polymers for enteric coating are acid-impermeable polymers such as cellulose acetate trimellitate, HPMC phthalate, polyvinyl acetate phthalate, cellulose acetate phthalate, and EUDRAGIT. Aspirin formulated as enteric-coated sustained-release tablets has been shown to produce less gastric bleeding than do conventional aspirin preparations. Film-coated tablets are compressed tablets coated with a thin layer of a water-insoluble or water-soluble polymer such as methylcellulose phthalate, ethylcellulose, povidone, or polyethylene glycol. Abacavir is a capsule-shaped film-coated tablet containing a nucleoside reverse transcriptase inhibitor, which is a potent antiviral agent for the treatment of HIV infection.
Tablet formulation
In addition to the drug, the following materials are added to make the powder system compatible with tablet formulation by the compression or granulation methods:
■ Diluents or bulking agents are invariably added to very-low-dose drugs to bring overall tablet weight to at least 50 mg, which is the minimum desirable tablet weight. Commonly used diluents are lactose, very low-dose, starches, micro-crystalline cellulose, dextrose, sucrose, mannitol, and sodium chloride. Dicalcium phosphate absorbs less moisture than lactose and is therefore used with hygroscopic drugs such as meperidine hydrochloride.
■ Adsorbents
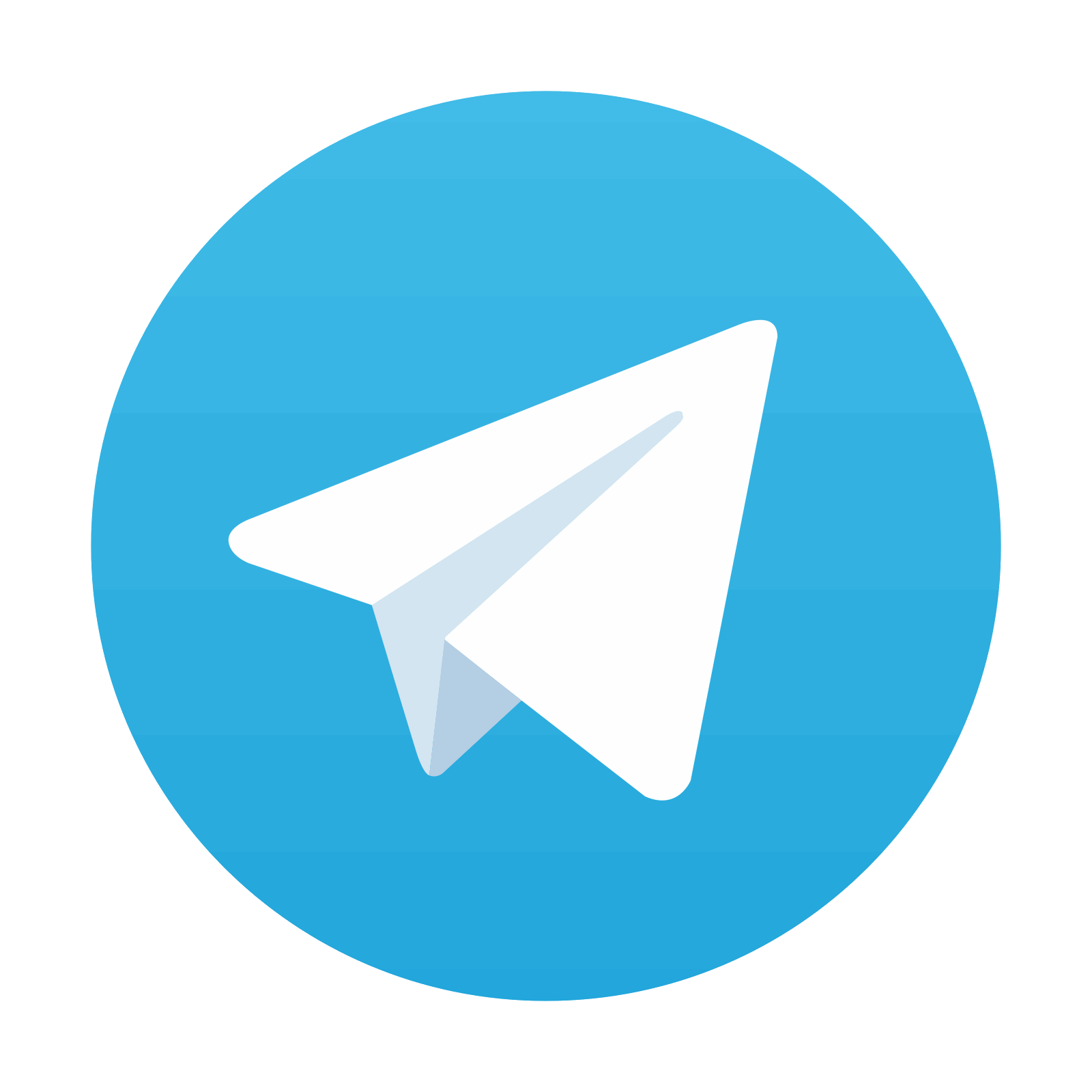
Stay updated, free articles. Join our Telegram channel

Full access? Get Clinical Tree
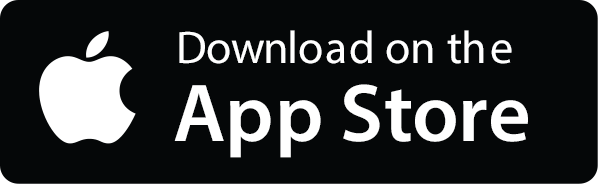
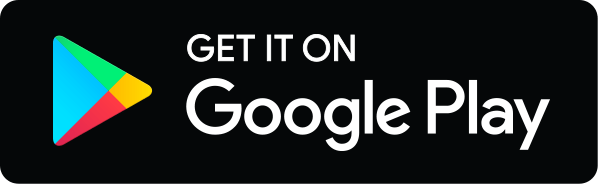