Introduction
Compared to a neonate, the pulmonary vascular resistance (PVR) in a fetus is almost twice as high. This is the result of intrauterine relative hypoxemia along with several other factors. After birth, the neonatal PVR gradually reduces and usually reaches adult level by 2 weeks of age. When PVR fails to lower, it causes a condition called persistent pulmonary hyper-tension of the newborn (PPHN), which affects 1.5 to 2 out of 1,000 newborns. If left undiagnosed and untreated, PPHN is associated with mortality and morbidity rates as high as 10 to 20%.1,2
Transition from Intrauterine to Extrauterine Circulation
To understand PPHN, clinicians must first understand the differences between fetal and postnatal (adult) circulation. Fetal circulation operates in parallel as opposed to single direction adult circulation. The fetal organ of respiration is the placenta. Oxygenated blood from the placenta is delivered to the right side of the heart via the inferior vena cava. Because the fetal lung does not participate in gas exchange, two channels in the fetal circulation allow blood to bypass the lungs: the foramen ovale, which connects the right and the left atria, and the ductus arteriole, which connects the aorta and the pulmonary trunk. The right ventricle is dominant, and blood shunts from right to left.1
Postnatal circulation, in contrast, operates in series. All venous return enters the right side of the heart and into the lungs. Gas exchange takes place in the lungs, and then oxygenated blood returns to the left side of the heart. The left ventricle pumps oxygenated blood into systemic circulation. The left ventricle is dominant, and there is no mixing of blood between the left and the right side of the heart.1
As a newborn takes the first breath, the removal of the placenta, the catecholamine surge associated with birth, and the relatively cold extrauterine environment all contribute to a steady rise in systemic vascular resistance (SVR). Meanwhile, expansion of the lungs, establishment of adequate alveolar ventilation and oxygenation, and successful clearance of fetal lung fluid all lead to a fall in PVR. Conditions that interfere with this postnatal decline of PVR/SVR ratio cause the persistence of a transitional circulation, resulting in PPHN.1
Pathogenesis and Causes
The abnormalities that are causes of PPHN can be divided into three main categories:
underdevelopment
maldevelopment
maladaptation
Underdevelopment
In underdevelopment abnormalities, the cross-section of vasculature is reduced, which results in increased elevation of PVR. Some conditions in this category are congenital diaphragmatic hernia (CDH), pulmonary hypoplasia, and intrauterine growth restriction. In patients with underdevelopment abnormalities, postnatal adaptation mechanism is limited. Therefore, patients in this category have the highest mortality rate.1
Maldevelopment
In the maldevelopment category, the lungs are normally developed but affected by an abnormally thick muscular layer of pulmonary arterioles. This muscular layer also extends into small vessels that normally have no muscle cells. Maldevelopment is associated with various conditions such as post-term delivery, meconium staining, and meconium aspiration syndrome (MAS) as well as premature closure of the foramen ovale or ductus arteriosus. Patients with idiopathic PPHN also fall under this category. Postnatal remodeling of the vascular bed usually occurs 7 to 14 days after birth, which leads to a gradual fall in PVR.1
Maladaptation
Even when lungs and pulmonary vasculatures are normally developed, the natural postnatal fall in PVR can still be disrupted. Maladaptation causes of PPHN are conditions that cause active vasoconstriction and, therefore, keep PVR elevated. These conditions include perinatal depression, pulmonary parenchymal disease, and bacterial infections. Group B Streptococcus (GBS) infection in particular has a potent effect on PVR. The bacterial phospholipid in GBS can activate vasoactive mediators and induce vasoconstriction.1
MAS is the most common cause of PPHN. Pulmonary hypertension in MAS is a result of airway obstruction, inactivation of surfactant, and chemical pneumonitis. Other PPHN risk factors in MAS are asphyxia, pneumothorax, and change of fetal heart beat pattern.
Risk Factors
The prevalence of PPHN is higher in male infants, infants born by cesarean section, infants who are late preterm, and infants who are large for gestational age. GBS infection is also a risk factor for PPHN.
Maternal risk factors include preconception body mass index >27, diabetes mellitus, and asthma. Histological chorioamnionitis and funisitis are also maternal risk factors. During pregnancy, maternal use of some medications such as non-steroidal anti-inflammatory drugs has also been linked to PPHN.1
Clinical Features and Diagnosis
Clinical Manifestations
Within 24 hours after birth, most infants with PPHN will develop signs of respiratory distress such as tachypnea, retractions, grunting, and cyanosis. Low APGAR (Appearance, Pulse, Grimace, Activity, Respiration) score and early delivery room interventions (e.g., intubation, bag and mask ventilation, oxygen) are also commonly observed. Meconium staining of skin and nails—indicative of intrauterine stress—may also occur.
Infants with PPHN often present with other respiratory complications. The Neonatal Research Network at the National Institute of Child Health and Human Development conducted a multicenter cohort study that included 385 infants. This study reported the relative frequencies of different respiratory complications: MAS, 41%; respiratory distress syndrome (RDS), 13%; pneumonia and/or RDS, 14%; CDH, 10%; and pulmonary hypoplasia, 4%. Among the 385 infants, 17% had no other complications and were diagnosed with idiopathic PPHN.1
Diagnosing PPHN
The following tests are helpful in differentiating the primary cause of hypoxemia in a term infant and diagnosing PPHN:
Hyperoxia Test—PPHN is characterized by hypoxemia that is non-responsive to supplemental oxygen. The hyperoxia test is a simple test, which can be performed at bedside. Place the infant in 100% oxygen. If PPHN is present, the partial pressure of oxygen (PaO2) in the blood of the infant usually does not increase.1
Simultaneous Pre- and Postductal Arterial Blood Gases—This test helps detect the right-to-left shunting across the patent ductus arteriosus (PDA). An arterial blood gas is obtained from the right radial artery (preductal) and umbilical artery catheter or posterior tibial artery (post ductal) simultaneously. A higher PaO2 in the right radial sample by ∼ 20 mmHg difference indicates right-to-left shunting.1
Chest Radiography—Although no specific chest radiography can confirm PPHN, the test is useful in suggesting or ruling out other diagnoses.1
Echocardiogram—The echocardiogram plays a key role in diagnosing PPHN and is an important tool in managing it. The initial echocardiogram rules out structural heart disease, determines the predominant direction of shunting at the patent foramen ovale and PDA, and assesses ventricular function. The PPHN diagnosis is not certain until predominantly bidirectional or right-to-left shunting is confirmed by echocardiogram.1
Determining the Severity of PPHN
The oxygenation index (OI), used to assess the severity of hypoxemia in PPHN, is a valuable tool in managing PPHN because it helps guide the timing of interventions.
OI = [mean airway pressure × FiO2 ÷ PaO2] × 100
OI is calculated based on the infant’s mean airway pressure (MAP), oxygen concentration of supplemental oxygen (FiO2), and PaO2. Most of the time when the OI is used, the infant is receiving ventilation with a FiO2 of 1 (100% oxygen). The OI can be calculated simply from the MAP displayed on the ventilator and the PaO2.
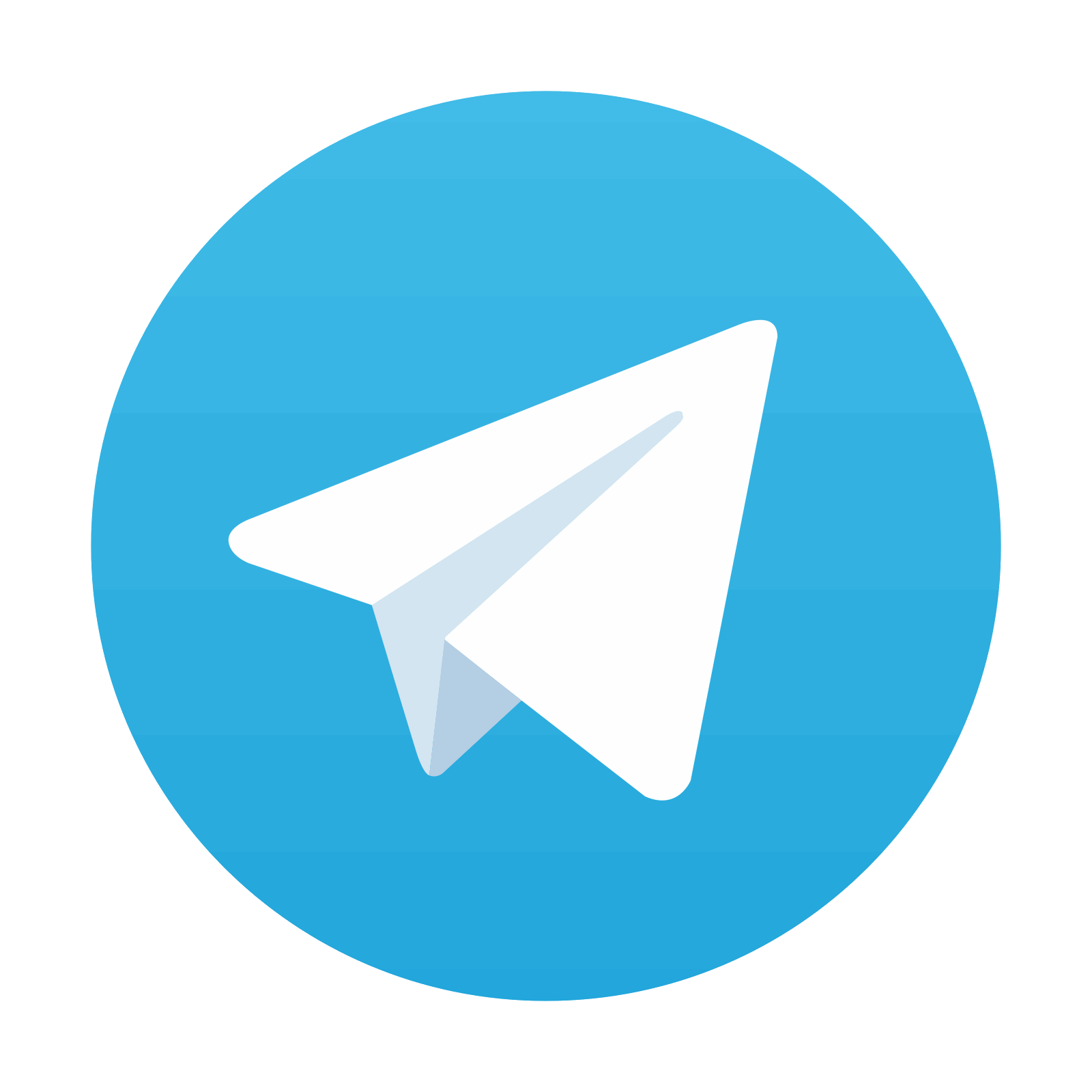
Stay updated, free articles. Join our Telegram channel

Full access? Get Clinical Tree
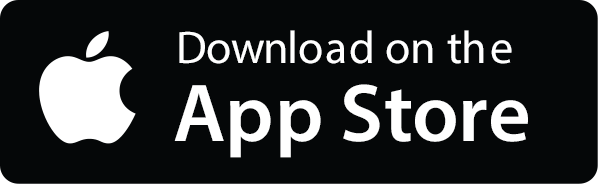
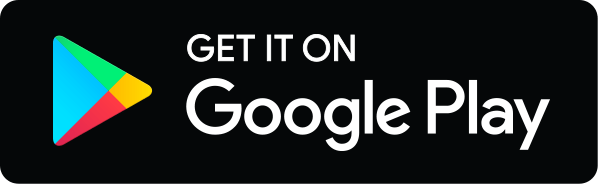