Objectives
- Understand the role played by the pancreas in digestion and absorption of a mixed meal
- Understand the structure of the exocrine pancreas and the cell types that give rise to proteinaceous and fluid components of the pancreatic juice
- Identify key constituents of the pancreatic juice and the enzymes that are secreted in inactive forms
- Describe the factors that regulate the release of secretin and the role of this hormone in stimulating pancreatic ductular secretion
- Understand the ion transport pathways expressed in pancreatic ducts and their mechanisms of action
- Understand the role of CCK and other factors in regulating pancreatic acinar cells
- Discuss the relative roles of monitor peptide and CCK-releasing peptide in regulating CCK release
- Identify signaling events activated in pancreatic acinar cells by secretagogues
- Compare and contrast the structure of the salivary glands with that of the exocrine pancreas
- Identify the functions of saliva and the constituents responsible for these
- Define ion transport pathways that produce saliva and modify its composition
- Define regulatory pathways for saliva production
- Understand conditions where production of saliva may be abnormal
Basic Principles of Pancreatic Secretion
The pancreas is the source of the majority of enzymes required for digestion of a mixed meal (i.e., carbohydrate, protein, and fat). Pancreatic enzymes are produced in great excess, underscoring their importance in the digestive process. However, unlike the digestive enzymes produced by the stomach and in the saliva, some level of pancreatic function is necessary for adequate digestion and absorption. In general, nutrition is impaired if production of pancreatic enzymes falls below 10% of normal levels, or if outflow of the pancreatic juice into the intestine is physically obstructed.
We should distinguish between the exocrine pancreas, responsible for producing secretions that flow out of the body, and the endocrine pancreas, the site of synthesis of various important hormones that regulate whole-body homeostasis, the most notable of which is insulin (Figure 4–1). These dual secretory functions of the pancreas are segregated to distinct anatomic locations. The functions and regulation of the exocrine pancreas are the province of gastrointestinal physiology, whereas the endocrine functions are a topic for discussion in a general endocrinology course. Thus, the latter will not be discussed further here.
The exocrine pancreas is the site of synthesis and secretion predominantly of enzymes. These fall into four main groups—proteases, amylolytic enzymes, lipases, and nucleases—as shown in Table 4–1. In addition, other proteins are produced that modulate the function of pancreatic secretory products, such as colipase and trypsin inhibitors. Finally, the pancreas secretes a peptide known as monitor peptide, which represents an important feedback mechanism linking pancreatic secretory capacity with the requirements of the intestine for digestion at any given moment after the ingestion of a meal; more on that topic later. The quantities of each of the secretory products differ greatly. Almost 80% by weight of the proteins secreted by the exocrine pancreas are proteases, with much lower quantities of the enzymes responsible for breaking down other classes of nutrients. Of the proteases, trypsinogen, the inactive precursor of trypsin, is by far the most abundant, accounting for approximately 40% by weight of pancreatic secretory products. This likely reflects a central role for trypsin in initiating the digestion of proteins, which will be discussed further in Chapter 15.
Proteases | Amylolytic enzyme | Lipases | Nucleases | Others |
---|---|---|---|---|
Trypsinogen* | Amylase | Lipase | Deoxyribonuclease | Procolipase* |
Chymotrypsinogen* | Nonspecific esterase | Ribonuclease | Trypsin inhibitors | |
Proelastase* | Prophospholipase A2* | Monitor peptide | ||
Procarboxypeptidase A* | ||||
Procarboxypeptidase B* |
As we learned for pepsinogen in the stomach, the proteases synthesized by the pancreas are packaged and stored as inactive precursors. This is also true for at least one lipolytic enzyme, prophospholipase A2. The need to store these enzymes in their inactive forms relates to the toxicity of the active products toward the pancreas itself. Under normal circumstances, therefore, the pancreas does not digest itself. Only in the setting of disease, particularly if the secretions are retained in the pancreas for a prolonged period, do the enzymes become inappropriately activated resulting in the very painful condition of pancreatitis.
Anatomic Considerations in Pancreas
As alluded to above, the pancreas has both exocrine and endocrine functions. The latter are restricted to endocrine cells located in the islets of Langerhans, which are scattered throughout the bulk of the pancreatic parenchyma. The exocrine functions, on the other hand, are conducted by a series of blind-ended ducts that terminate in structures known as acini. Many such acini, arranged like clusters of grapes, disgorge their products into a branching ductular system that empties into larger and larger collecting ducts, eventually reaching the main pancreatic duct or Wirsung’s duct. This duct merges with the common bile duct, coming from the liver, and the mixed bile plus pancreatic juice enters the duodenum a short distance distal to the pylorus, under the control of a sphincter called the sphincter of Oddi. A minor part of the pancreas is drained by an accessory collecting duct, known as the duct of Santorini, which has a separate opening to the duodenum. Both the acinar and ductular cells contribute distinct products to the pancreatic juice and both are regulated during the course of responding to a meal.
Pancreatic acinar cells are specialized exocrine secretory cells that are the source of the majority of the proteinaceous components of the pancreatic juice. They are somewhat triangular in shape when viewed in cross-section, with a basolaterally-displaced nucleus. The basolateral membrane faces the bloodstream and contains receptors for a variety of neurohumoral agents responsible for regulating pancreatic secretion. The apical pole of the cell, on the other hand, is packed at rest with large numbers of zymogen granules that contain the digestive enzymes and other regulatory factors. These granules are closely apposed to the apical membrane and thus to the lumen of the acinus. When the cell is stimulated by secretagogues, the granules undergo a process of compound exocytosis and fuse with each other and the apical membrane, thereby discharging their contents into the lumen.
The cells lining the intercalated ducts of the pancreas also play an important role in modifying the composition of the pancreatic juice. They are classical columnar epithelial cells, comparable to those lining the intestine itself, whose passive permeability is restricted by well-developed intercellular tight junctions. When stimulated, these cells transport bicarbonate ions into the pancreatic juice as it passes along the duct, with water following paracellularly in response to the resulting transepithelial osmotic gradient. Thus, the effect of the duct cells is to dilute the pancreatic juice and to render it alkaline. Quantitatively, the pancreas plays the major role in supplying the bicarbonate necessary to neutralize gastric acid so that appropriate digestion can take place in the small intestine.
The relative roles of acinar and ductular cells in contributing to the pancreatic juice can be demonstrated in animals fed a diet that is deficient in copper while also receiving the drug penicillamine. Among other effects, this treatment leads to atrophy of the pancreatic acini but has no effect on the ducts. Following a meal, such animals are unable to secrete pancreatic enzymes, but remain capable of increasing the volume of pancreatic juice due to the residual duct activity. In fact, the activity of the ductular cells is likely critical to “wash” the pancreatic enzymes out into the small intestine. Later in this chapter, we will consider the effects of cystic fibrosis, a disease state where ductular function is abnormal, on pancreatic secretory function.
Regulation of Pancreatic Secretion
As we saw for gastric secretion, pancreatic secretory activity related to meal ingestion occurs in phases. In humans, the majority of the secretory response (approximately 60–70%) occurs during the intestinal phase, but there are also significant contributions from the cephalic (20–25%) and gastric (10%) phases. Pancreatic secretion is activated by a combination of neural and hormonal effectors. During the cephalic and gastric phases, secretions are low in volume with high concentrations of digestive enzymes, reflecting stimulation primarily of acinar cells. This stimulation arises from cholinergic vagal input during the cephalic phase, and vago-vagal reflexes activated by gastric distension during the gastric phase. During the intestinal phase, on the other hand, ductular secretion is strongly activated, resulting in the production of high volumes of pancreatic juice with decreased concentrations of protein, although the total quantity of enzymes secreted during this phase is actually also markedly increased. Ductular secretion during this phase is driven primarily by the endocrine action of secretin on receptors localized to the basolateral pole of duct epithelial cells. The inputs to the acinar cells during the intestinal phase include CCK and 5-HT from the intestine as well as neurotransmitters including acetylcholine (ACh) and GRP. The large magnitude of the intestinal phase is also attributable to amplification by so-called enteropancreatic reflexes transmitted via the enteric nervous system. The mechanisms regulating CCK and secretin release during the intestinal phase will be addressed in the following sections.
CCK can be considered a master regulator of the duodenal cluster unit, of which the pancreas is an important component (Figure 4–2). CCK is a potent stimulus of acinar secretion, acting predominantly via CCK1 receptor-dependent stimulation of vagal afferents close to its site of release in the duodenum, thereby evoking vago-vagal reflexes that stimulate acinar cell secretion via cholinergic and noncholinergic neurotransmitters (the latter including both GRP and VIP). There are also CCK1 receptors on the basolateral pole of acinar cells, but it now seems likely that these are only activated if circulating concentrations of CCK rise to supraphysiologic levels. In addition to its effects on the pancreas, CCK coordinates the activity of other GI segments and draining organs, including by contracting the gallbladder (the physiologic function for which this hormone was named), relaxing the sphincter of Oddi, and slowing gastric motility to retard gastric emptying and thereby control the rate of delivery of partially digested nutrients to more distal segments of the gut. The latter activity serves to match luminal nutrient availability to the digestive and absorptive capacity of the small intestine. Finally, CCK can modulate the activity of other neurohumoral regulators in a synergistic fashion. Notably, while CCK is a weak agonist of pancreatic ductular secretion of bicarbonate by itself, it markedly potentiates the effect of secretin on this transport mechanism. During the integrated response to a meal, therefore, it is likely that the ability of secretin to evoke pancreatic bicarbonate secretion is amplified by occurring against the background of a CCK “tone.”
Nevertheless, CCK predominantly affects acinar cell secretion. Thus, during the initial response to a meal (i.e., the cephalic and gastric phases), pancreatic secretions are low in volume with a high concentration of enzymes and enzyme precursors. This situation should be contrasted with secretory flows occurring in the intestinal phase, where 5-HT and secretin also play a role. The effects of secretin are mediated predominantly at the level of the ducts. However, 5-HT, released from intestinal enterochromaffin cells in response to nutrients, activates a vago-vagal reflex that mirrors and augments that of CCK itself. It has been calculated that CCK and 5-HT are each responsible for about 50% of pancreatic enzyme secretion during the intestinal phase.
CCK is synthesized and stored by endocrine cells located predominantly in the duodenum, labeled in some sources as “I” cells (Figure 4–3). Control of CCK release from these cells is carefully regulated to match the body’s needs for CCK bioactivity. In part, this is accomplished by the activity of two luminally active CCK releasing factors, which are small peptides. One of these peptides is derived from cells in the duodenum, and called CCK-releasing peptide (CCK-RP). It is likely released into the lumen in response to specific nutrients, including fatty acids and hydrophobic amino acids. The other luminal peptide that controls CCK secretion is monitor peptide, which is a product of pancreatic acinar cells. Release of monitor peptide can be neurally mediated, including by the release of ACh and GRP in the vicinity of pancreatic acinar cells during the cephalic phase, and mediated by subsequent vago-vagal reflexes during the gastric and intestinal phases of the response to a meal. Likewise, once CCK release has been stimulated by CCK-RP, it too can cause monitor peptide release via the mechanisms outlined for acinar cell stimulation discussed earlier.
The significance of having peptide factors that regulate CCK release lies in their ability to match pancreatic secretion of proteolytic enzymes to the need for these enzymes in the small intestinal lumen. When meal proteins and oligopeptides are present in the lumen in large quantities, they compete for the action of trypsin and other proteolytic enzymes, meaning that CCK-RP and monitor peptide are degraded only slowly. Thus, CCK release is sustained, causing further secretion of proteases and other components of the pancreatic juice. On the other hand, once the meal has been fully digested and absorbed, CCK-RP and monitor peptide will be degraded by the pancreatic proteases. This then leads to the termination of CCK release, and thus a marked reduction in the secretion of pancreatic enzymes. This feedback mechanism for the control of CCK release, and in turn, pancreatic secretion, can be demonstrated in animals in which pancreatic juices have been diverted away from the intestinal lumen. In such experiments, CCK release in response to fatty acids or amino acids is potentiated and prolonged, presumably reflecting the persistence of CCK-RP.
The other major regulator of pancreatic secretion is secretin, which is released from S cells in the duodenal mucosa. When the meal enters the small intestine from the stomach, the volume of pancreatic secretions increases rapidly, shifting from a low-volume, protein-rich fluid to a high volume secretion in which enzymes are present at lower concentrations (although in greater absolute amounts, reflecting the effect of CCK and neural effectors on acinar cell secretion). As the secretory rate rises, the pH and bicarbonate concentration in the pancreatic juice rises, with a reciprocal fall in the concentration of chloride ions (Figure 4–4). These latter effects on the composition of the pancreatic juice are mediated predominantly by the endocrine mediator, secretin. The postprandial bicarbonate secretory response can largely be reproduced by intravenous administration of secretin, particularly if given with a low dose of CCK that potentiates ductular secretion, as discussed earlier.
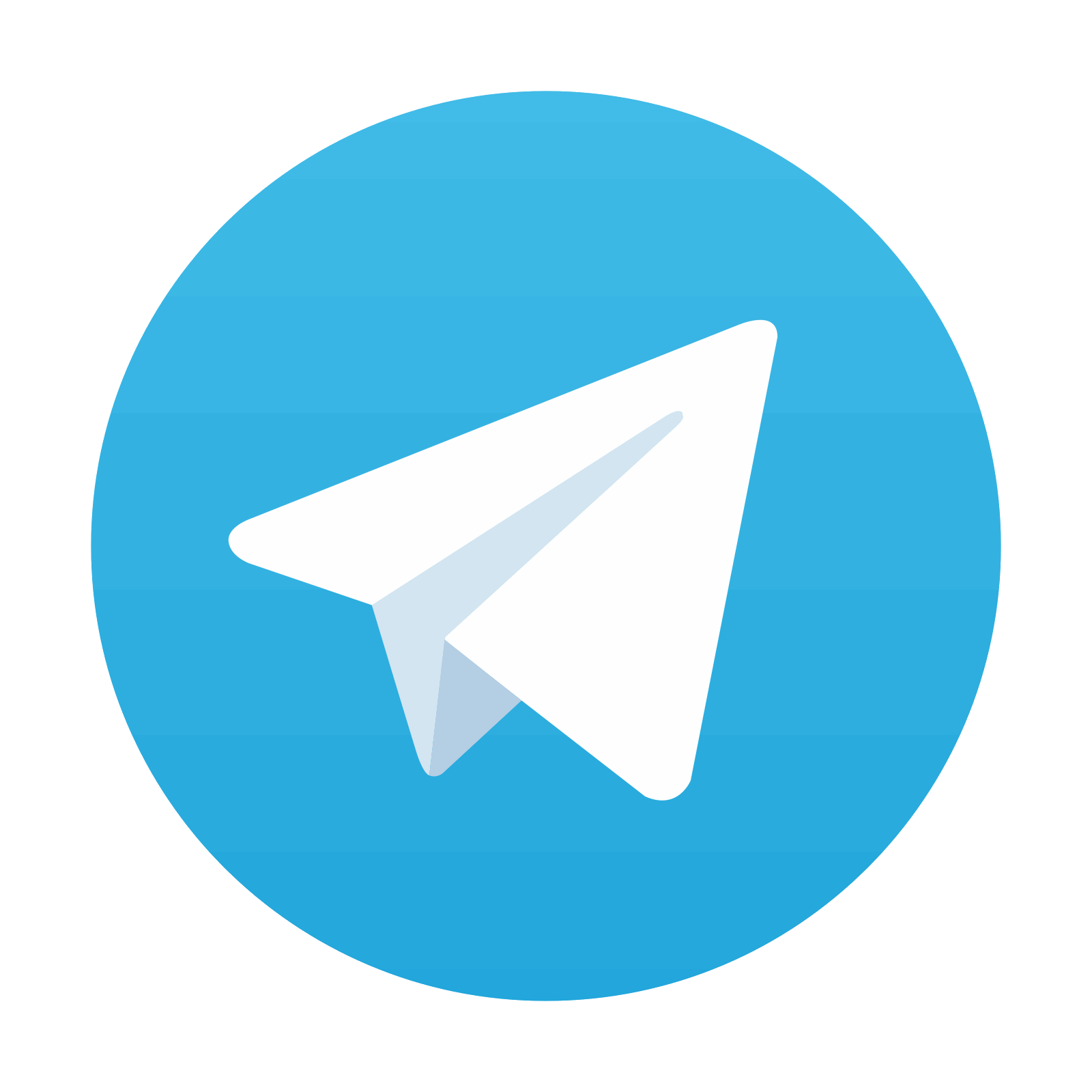
Stay updated, free articles. Join our Telegram channel

Full access? Get Clinical Tree
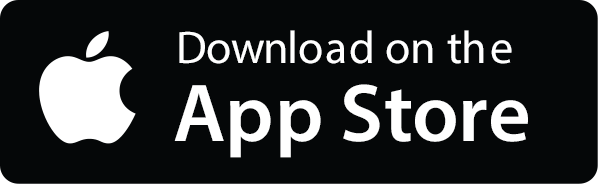
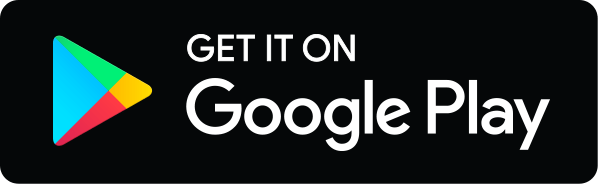