© Springer International Publishing Switzerland 2015
Josef Miller, Colleen G. Le Prell and Leonard Rybak (eds.)Free Radicals in ENT PathologyOxidative Stress in Applied Basic Research and Clinical Practice10.1007/978-3-319-13473-4_2525. Overarching Challenges of Free Radicals in ENT Pathology
Colleen G. Le Prell1 , Leonard P. Rybak2, Richard A. Altschuler3, 4 , Thomas Lenarz5 and Josef Miller6
(1)
Department of Speech, Language, and Hearing Sciences, University of Florida, 100174, Gainesville, FL 32610, USA
(2)
Division of Otolaryngology-Head and Neck Surgery, Department of Surgery, Southern Illinois School of Medicine, 19649, Springfield, IL 62794, USA
(3)
Department of Otolaryngology-Head and Neck Surgery, Kresge Hearing Research Institute, 5443 Medical Science Building 1, Ann Arbor, MI 48109, USA
(4)
Department of Cell and Developmental Biology, University of Michigan, Ann Arbor, MI 48109, USA
(5)
Department of Otorhinolaryngology, Medical University of Hannover, Carl-Neuberg-Str. 1, 30625 Hannover, Germany
(6)
Department of Otolaryngology-Head and Neck Surgery, Kresge Hearing Research Institute, 5428 Medical Science Building 1, Ann Arbor, MI 48109, USA
Keywords
ChallengesMultiple mechanismsAnimal modelsMetricsFocusResultsEpidemiological studiesGenomic studiesHuman trialsTargets of opportunity25.1 Challenge One: Potential for Multiple Mechanisms
One of the overarching challenges in the prevention of acquired hearing loss is the fact that while many insults converge on free radical-based mechanisms of injury or disease, oxidative stress is not the only factor contributing to the pathological process. Free radicals are clearly important, but they are not the whole story; insults can induce pathological changes and dysfunction by other mechanisms as well. Clearly, there are also a variety of factors that can lead to an upregulation of excessive free radical formation, and this excess may lead to pathology and cell death via a number of downstream pathways; however, many of these factors may induce pathology via parallel pathways. While inflammation and apoptosis follow oxidative stress, it is unclear under what conditions these pathological processes proceed independently from oxidative stress. Associated immune reaction during stress and during the healing process initiates biochemical processes, some of which include free radical formation, which influence the degree of pathology induced. The extent of pathology can reflect a variety of exogenous and endogenous factors each differentially associated with each of these processes. Thus, it is not surprising that a focused antioxidant treatment that reduces oxidative stress may not provide complete prevention, particularly in the case of more robust insult to the ear or other head and neck tissues. While this should not delay translational studies, a better understanding of biochemical pathways involved in free radical-induced pathology in various cell types of the inner ear and how they interact, including hair cells, supporting cells, and cells in the lateral wall, as well as cell types of other structures of the head and neck affected by oxidative stress, will surely facilitate human trials allowing a more informed selection of putative interventions and more relevant measure of efficacy. A more nuanced understanding of the pathways to pathology, their interaction, and their hierarchy will guide the development of new “cocktails” of intervention, e.g., antioxidant, anti-inflammatory, and antiapoptotic agents targeting specific genes and signaling molecules, optimizing treatment of different types and levels of insult and disease.
25.2 Challenge Two: Choice of Animal Models and Rigor of Design
Current animal models may not be adequate to represent the human condition. Animal models are frequently good for a particular study of an insult and intervention; they are sometimes not good enough to provide needed generalization of result to a more complex human condition. Clearly we need tests across different animal models with different stresses, interventions, and dose response data on stress and intervention. Thus, a key issue is the selection of species, which may vary as a function of the disease and injury, the intervention, and the insult/injury. For example, the literature on otoprotection (against noise) has limited utility for comparisons across agents because the type and extent of the injury and the animal models vary considerably across the different assessed agents. With respect to hearing loss induced by drugs such as the aminoglycoside antibiotics and cisplatin, the injury induced in animal models often exceeds the injury in drug-treated patients. With respect to trauma induced by electrode insertion, it is difficult to precisely control mechanical injury in either animal models or human patients given that this varies with both surgical skill and patient anatomy. Moreover, the various factors, including the interplay of genetic and environmental factors, contributing to long-term slowly progressive pathology (evidenced as increasing hearing loss) are unclear. These questions notwithstanding, this is a significant issue given that the majority of patients with residual hearing lose some or all postimplantation and with that lose some benefits.
In considering the utility of animal model data for design of human studies, it is increasingly clear that an “overarching” concern must include far greater rigor in animal investigations. Rigorous application of scientific methodology, including power analysis, blinding, carefully selected and time-distributed controls, objective and relevant outcome measures, careful adherence to statistical analysis including the identification in advance of primary measures for analysis, replication of results, and other factors must all be performed with care and thoughtful consideration (Landis et al. 2012; Katz et al. 2012a, b).
25.3 Challenge Three: Need for Relevant Metrics
Many of our current metrics do not span the animal model—human gap. As a field, there is a pressing need for metrics with general comparability between people and animals. There is a uniform agreement that conventional pure tone air conduction thresholds are a prerequisite starting point in humans, and auditory brainstem response thresholds are the typical correlate in animal subjects. However, the most common patient complaint is difficulty understanding speech in noisy or reverberant environments, and we need metrics in animals that can model these more complex perceptual deficits in humans. Two possibilities available are operant psychophysical tasks in which trained animals report discrimination of sounds with noise maskers in the background and collection of multiunit neural response data from inferior colliculus. While these measures are sufficiently time consuming and specialized that it is unlikely they can be incorporated broadly, selective use could bring great clarity to many important issues. One design paradigm that may be much more readily implemented within a broad variety of laboratories is the startle-based gap detection task, which can be a sensitive, albeit somewhat variable, measure of temporal processing, but which does not require animals be trained to perform an operant task. Frequency following response may provide a metric that could be adopted as a test for central auditory function. There is a broad agreement that human clinical studies should employ a test battery that includes a words-in-noise test; the National Institutes for Health (NIH) toolbox offers one possibility for a test that is optimized for speed and ease of use.
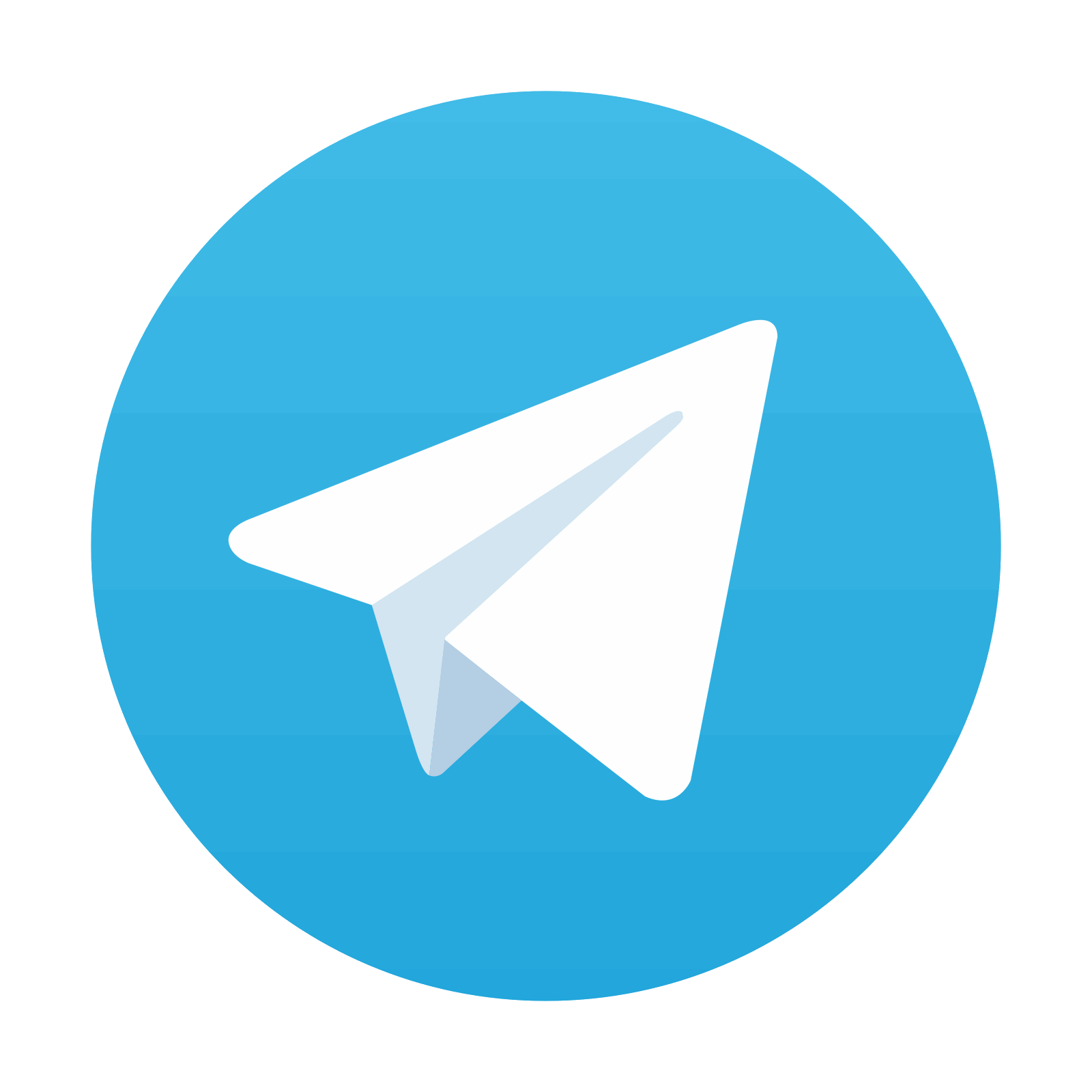
Stay updated, free articles. Join our Telegram channel

Full access? Get Clinical Tree
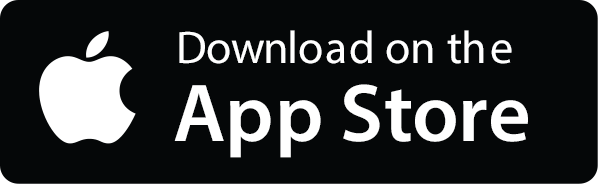
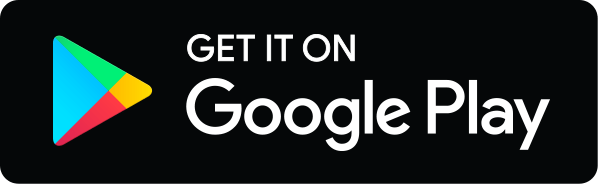