Introduction
Current European Society of Cardiology (ESC) and American College of Cardiology (ACC)/American Heart Association (AHA) guidelines for the diagnosis and management of infective endocarditis (IE) require the association of an infective syndrome and confirmation of endocardial involvement to establish the diagnosis [ , ]. Although the “standard modified Duke criteria” are commonly used, these have a lower diagnostic yield in patients with prosthetic heart valves, implantable electronic devices, or nondiagnostic echocardiography [ ]. Modification of these criteria by the ESC in 2015 incorporated additional imaging modalities to improve diagnosis in these situations ( Table 5.1 ), including (1) incorporation of paravalvular lesions detected by computed tomography (CT) (major criterion), (2) abnormal 18 F-FDG positron emission tomography (PET)/CT at the site of a prosthetic heart valve >3 months following implantation (major criterion), and (3) detection of recent embolic events using imaging alone (minor criterion).
Major criteria |
|
Minor criteria |
|
|
|
|
|
a HACEK = Haemophilus parainfluenza/aphrophilus/paraphilus/influenza, Actinobacillus actinomycetemcomitans, Cardiobacterium hominis, Eikenella corrodens, Kingella kingae/denitrificans .
These additions were not intended to diminish the relevance of echocardiography in the setting of IE but to enhance overall diagnostic certainty in patients with suspected IE and limited echocardiographic information. Transthoracic echocardiography (TTE) retained a Class I indication as the first-line imaging modality, while transesophageal echocardiography (TEE) received a Class I recommendation for all patients with a clinical suspicion of IE and a negative or nondiagnostic TTE, and in patients with a prosthetic heart valve or intracardiac device (reflecting reduced sensitivity of TTE to detect vegetations on prosthetic valves vs. native valves [50% vs. 76%] and improved sensitivity of TEE in both settings [native valves 96%, prosthetic heart valves 92%]) [ ].
While the ESC guidelines include CT and PET imaging within the modified Duke diagnostic criteria, the ACC/AHA guidelines highlight the value of these imaging modalities in the detection of extracardiac complications of IE [ ]. Notwithstanding this subtle difference, both bodies acknowledge the ability of novel imaging techniques to provide supplementary diagnostic information beyond the anatomical and hemodynamic findings of echocardiography [ ].
Computed tomography—angiography
Although cardiac CT initially focused on coronary and vascular imaging, multiple studies have shown increasing utility in suspected IE. Technological advances have led to significant improvement in scanner spatial resolution to <0.5 mm, enhancing the capability to visualize valve morphology (vegetations/perforations) and perivalvular complications (abscesses/pseudoaneurysms) [ , ].
Diagnostic performance
Native-valve endocarditis
Given the high diagnostic accuracy of TEE in the detection of IE-related lesions in native valves, there are minimal data concerning the diagnostic performance of alternative imaging methods in this setting. Multidetector CT angiography has shown comparable diagnostic performance compared to TEE as the gold standard for IE imaging, particularly in detection of valvular and paravalvular complications. In a study of 37 patients with clinically suspected IE, cardiac CT showed a diagnostic accuracy of 96% (sensitivity 97%, specificity 88%) with regard to intraoperative findings, and high levels of agreement with TEE with respect to vegetation size and mobility (but not leaflet perforation) [ ]. In a further study of 19 patients with aortic valve IE requiring surgery, CT was highly accurate in the detection of aortic valve pseudoaneurysms (sensitivity 100%, specificity 87.5%) or perivalvular extension (sensitivity 100%, specificity 100%). Although all vegetations >10 mm were accurately detected, CT failed to recognize smaller lesions. As a result, diagnostic performance was poorer in overall vegetation recognition (sensitivity 71.4%, specificity 100%) [ ]. In a later series of 71 affected valves (70% native), CT imaging yielded a sensitivity and specificity of 81% and 90% for the detection of abscesses and pseudoaneurysms. Conversely, TEE was superior to CT in the detection of vegetations and leaflet perforation. The combination of both modalities allowed detection of all abscesses and pseudoaneurysms confirmed at surgery compared to echocardiography or CT alone [ ].
On the basis of these observations, cardiac CT has a primary role in native-valve IE as an adjunctive imaging test where perivalvular complications are suspected but cannot be adequately excluded by echocardiography alone.
Prosthetic-valve endocarditis
Echocardiographic imaging is often limited in the setting of prosthetic heart valves where acoustic shadowing limits visualization of leaflets and periannular structures. In a study involving 27 patients with suspected prosthetic valve (16 of whom underwent surgery), CT imaging agreed closely with TEE findings in patients with aortic wall thickening, but less closely in those with abscess or valve dehiscence (and only moderately in detecting vegetations) [ ]. In a small study of 28 patients with suspected prosthetic-valve IE, use of CT as adjunct imaging beyond TTE and TEE demonstrated additional findings (predominantly mycotic aneurysms) resulting in a recommendation for surgery rather than conservative treatment in 21% of patients [ ]. In a further study of 67 patients with aortic prosthetic-valve IE, perivalvular abscesses providing an indication for surgery were detected on CT but not on TEE in 14% of the cohort ( Figs. 5.1 and 5.2 ) . Conversely, lesions providing an indication for surgery were detected by TEE but missed by CT in 19% of patients (with complete agreement in 85% of cases) [ ]. Diagnostic performance of CT was superior with respect to perivalvular complications and inferior with respect to vegetations, valve perforation, and perivalvular leaks, as confirmed in multiple subsequent studies [ ].


These findings suggest that CT should be a complementary investigation to TEE for the assessment of suspected prosthetic-valve IE. Combined imaging leads to higher rates of detection of IE-related pathology and allows comprehensive evaluation of potential complications and alternative diagnoses [ ].
Additional benefits of computed tomography—angiography and key limitations
Cardiac CT has a well-defined role in surgical procedural planning in patients who have undergone previous cardiac surgery ( Fig. 5.3 ) and is also the preferred technique for noninvasive evaluation of the coronary arteries in patients with aortic valve IE and aortic root pathology to mitigate the risk of catheter-induced aortic trauma and systemic embolism of aortic valve vegetations [ , , ]. CT is also commonly recommended for the detection of embolic complications of IE or the exclusion of other occult sources of infection when cardiac imaging is normal ( Fig. 5.4A and B ) [ ].



Key limitations of cardiac CT are the use of ionizing radiation, need for iodinated contrast, and the related risk of acute kidney injury and allergic reactions. Furthermore, imaging of the coronary arteries and heart valves requires slow and stable heart rates (owing to its limited temporal resolution) which may be problematic since intravenous or oral beta blockade is relatively contraindicated in patients with congestive cardiac failure or severe valvular incompetence. Prosthetic heart valves and cardiac implantable electronic devices (CIEDs) can also create substantial hard beam artifacts that limit evaluation of key structures [ ].
Molecular imaging
Whereas TTE and TEE provide excellent visualization of native heart valves, they are limited in the presence of prosthetic heart valves and CIEDs. In these instances, molecular nuclear imaging techniques provide complementary information to localize potential sources of infection when vegetations are not clearly seen [ , ].
General principles of molecular imaging—pros and cons
The key principle of PET is the ability of radionuclide-labeled agents to localize in areas of inflammation and infection. In clinical practice, 11 In- or 99 mTc-labeled autologous white blood cells (WBCs) and 18 F-fluorodeoxyglucose (18 F -FDG) have been used to visualize foci of infection and inflammation, respectively [ ]. To achieve better anatomical correlation, both methods utilize superimposed, hybrid whole body PET gamma cameras to assess radiolabeled agent uptake (molecular imaging) and CT imaging for anatomical orientation [ ]. Hybrid imaging with PET complemented by ECG-gated cardiac CT angiography has been proposed as an alternative approach, combining the benefits of molecular imaging and high spatial and temporal resolution of CT angiography for optimal, comprehensive anatomical imaging [ ].
Compared to echocardiography, both methods bear the advantage of full body acquisition that allows accurate detection of extracardiac embolic sequelae of IE [ ]. In addition, both methods allow recognition of potential sources of infection, both as the origin of IE and as a differential in septic patients ( Fig. 5.5 ) [ ]. Being based on the principles of molecular imaging, they have proved useful in assessing the early stages of inflammation and infection, even before the cascade of IE results in anatomically recognizable changes [ , ]. Finally, both methods have been successful in detecting CIED infection extending from the generator pocket to the lead tips. In a similar fashion, both modalities appear to be sensitive in the detection of prosthetic-valve endocarditis and have been used in the assessment of suspected left ventricular assist device (LVAD) infection.

Both techniques require ionizing radiation dose exposure in the range of 3–8 mSv from the tracer emission and about 5 mSv from the CT scan required for attenuation correction [ ]. This has necessitated a rationed approach in clinical practice in conjunction with echocardiography as the first-line imaging test. Despite similarities of acquisition, WBC and FDG PET imaging are fundamentally different in terms of patient preparation, radiolabeling, and imaging targets, thus yielding dissimilar results in the assessment of patients with suspected IE.
Radiolabeled white blood cell PET imaging
Basic principles
In order to perform a WBC PET study, the patient’s WBCs are collected from a blood sample (usually 40 mL) and radiolabeling performed in vitro. Radiolabeling is a complex, time-consuming, and expensive process with an additional risk of pathogen contamination. Once the leukocytes are labeled ex vivo (most frequently with 99 mTc-hexamethyl propylene amine oxime, 99 mTc-HMPAO), they are reinfused to the patient before image acquisition 5 and 21 h later (together with low-dose CT scanning to allow anatomical correlation of uptake). There is no specific requirement for patient preparation.
Uptake is influenced by a number of factors, such as quality of radiolabeling, prior antibiotic treatment (and its duration), chemotactic properties of infected tissues, and the type and amount of microbial pathogens [ ]. A notable advantage of 99 mTc-HMPAO-labeled WBC single-photon emission computed tomography/computed tomography (SPECT/CT) compared to other molecular diagnostic techniques is the ability to visualize infectious foci in the brain (which is not possible with 18 F-FDG owing to innate physiologic tracer uptake by the brain regardless of patient preparation).
Diagnostic performance
In a series of 63 consecutive patients with suspected CIED-related IE, 99 mTc-HMPAO-WBC SPECT/CT yielded a sensitivity of 94% for the detection and localization of infective foci on the CIED. None of the patients with a negative scan developed IE over 12-month follow-up, with an estimated negative predictive value of 95% [ ].
Compared to TTE, 99 mTc-HMPAO-WBC SPECT/CT has superior ability to detect “true” IE, even in patients with lesions considered diagnostic. In a series of 40 patients with suspected IE, a diagnosis was clinically confirmed in 14 patients. Both radiolabeled leukocyte PET and TTE were equally sensitive (93%) in detecting lesions associated with a clinical diagnosis of IE at follow-up. However, false-positive rates of IE were much lower with 99 mTc-HMPAO-WBC SPECT/CT compared to TTE, resulting in a higher specificity of 88% versus 42% [ ].
Incorporating the use of 99 mTc-HMPAO-WBC SPECT/CT into diagnostic algorithms can lead to reclassification of patients from possible to definite IE according to the modified Duke criteria. In a further study of 131 consecutive patients, a clinical diagnosis of IE was established in 51 subjects at 12-month follow-up. 99 mTc-HMPAO-WBC SPECT/CT was truly positive in 46 patients with no false-positive cases. The detection of pathologic peripheral uptake allowed reclassification of 11 patients from possible to definite IE according to modified Duke criteria [ ].
With a reported negative predictive value exceeding 93%, 99 mTc-HMPAO-WBC SPECT/CT is particularly useful for the exclusion of CIED-related infection [ , ]. In a series of 103 consecutive patients, 49.5% had possible CIED infection according to modified Duke criteria, while exclusion of infection by 99 mTc-HMPAO-WBC SPECT/CT allowed a 12.5% reduction in the number of patients with possible IE category [ ].
These findings demonstrate that 99 mTc-HMPAO-WBC SPECT/CT is highly accurate in excluding IE in the presence of suspicious findings and enables diagnostic confirmation and correct classification. Caution is required in nonbacterial IE (e.g., marantic or fungal endocarditis) since 99 mTc-HMPAO-labeled leukocyte SPECT/CT may be falsely negative due to reduced leukocyte chemotaxis [ ]. Some bacterial microorganisms, such as Staphylococcus epidermidis or strains of Enterococcus spp., may elude host immune system detection by developing a biofilm resulting in reduced leukocyte recruitment at areas of infection [ ]. Prior antibiotic treatment may also result in reduced uptake, thereby reducing sensitivity and specificity [ ].
18 F-fluorodeoxyglucose PET imaging
Basic principles
The fundamental principle underlying 18 F-FDG PET imaging is that areas of inflammation attract glucose as a consequence of bacterial and immune cell metabolism. In order to remove any background metabolic uptake, patients have to fast for 6–12 h or follow a low-carbohydrate, high-fat diet for at least 12–24 h prior to investigation, with administration of heparin immediately prior to the injection of the radiolabeled tracer and image acquisition [ , ]. This process suppresses physiological myocardial glucose metabolism, thereby nulling the myocardial signal in the absence of inflammation. Physiological uptake persists in the brain (and partially in the liver and intestine), as well as in the urinary tract through which the tracer is excreted. In addition, neoplastic tissue exhibits increased, nonsuppressed uptake as described in patients with presumed IE who are eventually diagnosed with a malignancy [ ].
Qualitative and semiquantitative image interpretation can be performed by measurement of signal intensity in areas of uptake to calculate standardized uptake values (SUVs), these being influenced by a series of factors, including local protocols, the timing of image acquisition, scanner properties, patient body habitus, and glucose levels, as well as anatomy of the imaged tissue [ , ]. A focal SUV that is twice the value measured at the liver parenchyma has been proposed as a discriminator of true inflammation, but this has not been validated in large prospective cohorts [ ].
A key consideration for imaging analysis is that 18 F-FDG PET visualizes glucose metabolism per se, and that this does not necessarily reflect infection. Thus, increased uptake may be observed early after valve surgery in the absence of infection ( Fig. 5.6 ) as a consequence of aseptic inflammation [ ]. Signal intensity measured by SUV may be less in inflammation compared to infection but cut-off values vary depending on patient preparation, acquisition technique, and timing of scanning [ ]. The utility of 18 F-FDG PET in the detection of early IE following valve surgery may therefore be limited since “physiological” uptake may persist up to 12 months postsurgery [ , ], and rare cases of persistent uptake up to 13 years have been described [ ]. Current guidelines suggest that 18 F-FDG PET may be performed in patients with a high likelihood of IE at least 3 months following prosthetic-valve implantation [ ]. However, patients should not be automatically excluded from investigation, even in cases of early suspected IE, since differentiation between true infection and aseptic inflammation may be feasible on the basis of the morphological distribution of uptake (being more homogeneous and localized at areas of surgical manipulation) [ ].

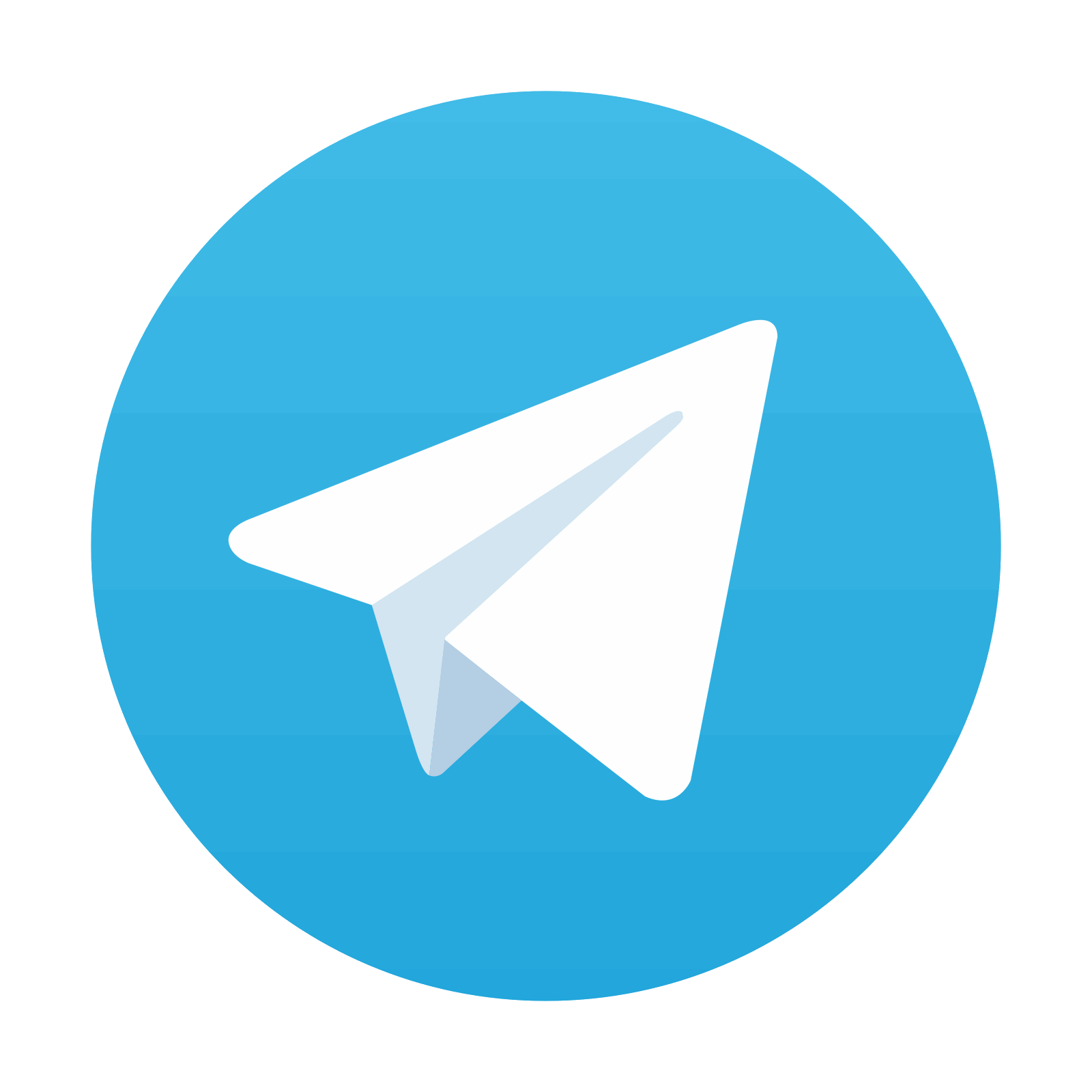
Stay updated, free articles. Join our Telegram channel

Full access? Get Clinical Tree
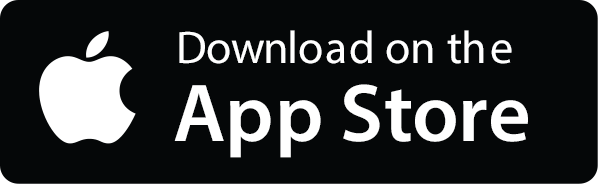
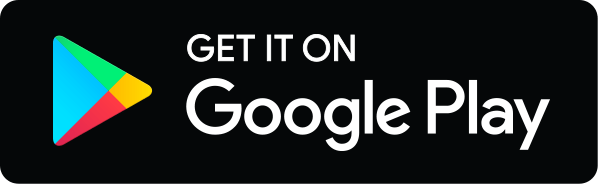
