A Retrospective View on the Hallmarks of Neurobiological Alcohol and Drug Abuse Research
What were the major achievements in the past in neurobiologically oriented alcohol and drug abuse research? This can only be answered by a very personal view. I would like to illustrate this in terms of the hallmarks of alcohol research. In 1940, Curt Paul Richter reported that laboratory rats voluntarily consume alcohol, although with high individual variability. This discovery marked the beginning of animal research in the study of alcohol. Furthermore, this observed variability in alcohol intake provided the basis for the generation of alcohol-preferring and nonpreferring rat and mouse lines, eight of which have been genetically selected since 1960. Thousands of studies on alcohol drinking in rodents have been conducted subsequently, permitting the deciphering of the genetic and neurochemical basis of alcohol reinforcement. Studies of alcohol self-administration in laboratory animals remain crucial to the development of medication in the field of alcohol research, and the predictive value of these models is demonstrated by the fact that all available pharmacotherapies (e.g., naltrexone and acamprosate) have been based on animal work of this nature. The same is true for any other drug of abuse—without appropriate animal models only little progress would have been made in the field of addiction research. In fact, most of the animal models (e.g., intravenous self-administration of heroin and cocaine) provide excellent face and construct validity.
In terms of construct validity, the discovery of the brain reinforcement system by James Olds in 1954 —one of the outstanding experimental psychologists of the last century—ultimately provided the key to understanding the neuroanatomical correlates underlying alcohol and drug reinforcement. Again, knowledge derived from animal work on the neuroanatomical and functional aspects of alcohol and drug reinforcement has been systematically translated to humans by means of neuroimaging techniques.
The foundation for understanding the neurochemical substrates of alcohol and drug reward was laid by the three research teams in 1973 responsible for identifying the first opioid receptors. In the hunt for the endogenous ligands, John Hughes and Hans Kosterlitz then identified the first opioids in the brain only 2 years later, and called them enkephalins. However, it took almost two decades until the molecular cloning of the first opioid receptors was achieved. These studies not only promoted opioid research in general, but also represented key discoveries for subsequent alcohol and drug abuse research. Similarly, the isolation of Δ 9 -tetrahydrocannabinol in 1964 by the group of Ralph Mechoulam marked the beginning of cannabinoid research. The brain targets of tetrahydrocannabinol remained unidentified until 1988, when a seminal paper by the group of Allyn Howlet identified a G-protein–coupled receptor as the target of natural cannabinoids. It was followed immediately by the molecular cloning of the cannabinoid receptor 1 60 and by the identification of the first endogenous ligand of the cannabinoid receptor, an arachidonic acid derivative termed anandamide. These key discoveries led to one of the most active fields of research in neurobiology. But the real surprise came from the discovery of the role of the endocannabinoid system in reward processes and in the neurobiology of addictive behavior. Both the endocannabinoids and the cannabinoid receptor appear to be crucial in opioid, alcohol, psychostimulant, and nicotine addiction and it can be foreseen that within the next 10 years we will have effective treatments on the market targeting various components of the endocannabinoid system.
In addition to endocannabinoids, endogenous opioid systems are thought to induce the pleasurable and rewarding effects of alcohol and other drugs of abuse, and thereby constitute ideal targets for treatment. The first description of opioid receptor blockade by means of naltrexone, and the resultant reduction of voluntary alcohol consumption in rats marked the starting point of the development of relapse medication in alcohol research. A decade later, the first reports on the clinical efficacy of naltrexone in alcohol-dependent individuals were published and a recent meta-analysis of 24 randomized controlled trials that included a total of 2861 subjects demonstrates that naltrexone decreased the relative risk of relapse compared to placebo by a significant 36%. A further milestone in medication development was the finding that a functional polymorphism of the μ-opioid receptor gene may predict response to naltrexone. Although this finding has been replicated recently, no final judgement on this pharmacogenetic discovery will be possible for several years. Nevertheless given that our century is dominated by the belief that personalized medicine will power further biomedical developments, the study of Oslin et al. has already marked this shift in paradigms. Despite the promise of pharmacogenetics to identify treatment responders, there have so far been very few success stories in all of medicine.
New Vistas in Neurobiological Alcohol and Drug Abuse Research
Addictive behavior is the result of cumulative responses to drug exposure, the genetic make-up of an individual, and the environmental perturbations over time. This very complex drug × gene × environment interaction, which has to be seen in a lifespan perspective, cannot be studied by a reductionistic approach. Instead, a systems-oriented perspective in which the interactions and dynamics of all endogenous and environmental factors involved are centrally integrated, will lead to further progress in alcohol and drug abuse research. My future perspective adheres to a systems biology approach such that the interaction of a drug with primary targets within the brain is fundamental to an understanding of the behavioral consequences. As a result of the interaction of a drug with these targets, alterations in gene expression and synaptic plasticity take place that either function as protective mechanisms or lead to long-lasting alteration in neuronal network activity. As a subsequent consequence, drug-seeking responses ensue that can finally lead via complex environmental interactions to an addictive behavior ( Fig. 74.1 ). This systems biology approach opens up new vistas in addiction research on the genetic (see Section “New Vistas on the Genetic Level”), molecular (see Section “New Vistas on the Molecular Level”), synaptic (see Section “New Vistas in Alcohol- and Drug-Induced Synaptic Plasticity”), neuronal network (see Section “New Vistas on Neuronal Network Activity”), and finally on the behavioral level (see Section “New Vistas on Studying Alcohol- and Drug-Related Behaviors”).

New Vistas on the Genetic Level
Genetics of Addictive Behavior
A large body of genetic epidemiological data strongly implicates genetic factors in the etiology of addictive behavior. In the following I focus mainly on smoking behavior, as progress in genetics is most pronounced in the field of nicotine addiction. The data from family, adoption, and twin studies strongly support a genetic influence on the initiation and maintenance of smoking. Two general scientific human approaches to identify candidate genes are genetic linkage analysis and genetic association studies including genome-wide association studies. Despite the success of linkage approaches in unravelling the genetic antecedents of disease, the findings with respect to smoking behavior have been disappointingly inconsistent. However, a variety of plausible candidate genes have been examined for associations with smoking behavior. Most of these studies have focused on genetic variations in relevant neurotransmitter pathways and/or nicotine-metabolizing enzymes or neuronal nicotinic receptors. Despite the large number of studies published on the association between specific candidate genes and smoking behavior, one has to conclude from the existing literature that the evidence for a contribution of a specific gene to smoking behavior is rather small.
Genome-Wide Association Studies in Addiction Research
Genome-wide association studies employing a high number (500,000 + ) of single nucleotide polymorphisms across the genome have been conducted in a variety of complex disorders and have been shown to be a successful tool in identifying underlying susceptibility genes (for all published genome-wide association studies see: www.genome.gov/26525384 ). Several genome-wide association studies have recently also been conducted on smoking behavior phenotypes. These studies have used sample sizes of up to 11,000 cases and have implicated a number of novel genes in nicotine addiction and smoking cessation, as well as known candidate genes. Especially, in conjunction with several candidate gene studies, evidence has been accumulated that genes encoding nicotinic acetylcholine receptor proteins are associated with multiple smoking phenotypes. In particular, the nicotinic acetylcholine receptor subunit genes CHRNA3 , 4 and 5, as well as CHRNB4 are associated with nicotine addiction. Although the robust association of the nicotinic acetylcholine receptor subunit genes investigated with smoking-related phenotypes is an apparent success story of genetic epidemiology, the respective variations seem to exert no relevant influence on smoking cessation probability in heavy smokers in the general population. These data suggest that the corresponding nicotinic acetylcholine receptor single nucleotide polymorphisms are relevant to the development of chronic smoking behavior but might not influence abstinence and relapse behavior. Although this is somewhat discouraging regarding the usability of genetic determinants of susceptibility to nicotine addiction as predictors of smoking cessation, it highlights the importance of taking this highly interesting phenotype explicitly into account in future studies. In conclusion, genome-wide association approaches as discussed here offer great promise for detecting candidate genes for the development of chronic smoking behavior and relapse, respectively. However, the demonstration of a causal relationship of a specific genotype with a pathological phenotype is difficult, if not impossible, to achieve in humans.
Forward Genetics in Preclinical Addiction Research
Animal Models as the Basis for Forward Genetic Approaches
Animal studies using intravenous self-administration in rodents represent a powerful method to functionally validate candidate genes deriving either from human genome-wide association study approaches or from gene expression profiling studies in animals. Intravenous self-administration is commonly used as an animal model for studying nicotine intake as it offers face validity from various perspectives; for example, there is good concordance between the nicotine concentrations in plasma of human cigarette smokers and of rats in intravenous self-administration studies. Furthermore, genetic differences have been reported because various strains of rats show different latencies to acquire nicotine self-administration behavior. Thus studies on the acquisition of intravenous self-administration will help to elucidate the genetic vulnerability of the development of chronic smoking behavior in humans.
Major progresses in animal models for addictive behavior also allow now the study of compulsive nicotine-seeking behavior and relapse. The most common procedure to study nicotine-seeking behavior—which can be considered as the motivational component of nicotine craving and relapse—is the reinstatement model. In this model, intravenous self-administration of nicotine paired with conditioned cues has to be acquired at first. This is followed by a nicotine-free period where the animals undergo extinction (i.e., allowing the animal to perform the operant response without programmed consequences). Finally, in response to previously conditioned cues reinstatement can be tested. If instead of extinction training protracted abstinence is applied, responsiveness to conditioned cues progressively increases over the first weeks of abstinence (i.e., the animals remain in their home cage for at least 1 month without any further conditioning)—a phenomenon called incubation. In summary, reinstatement of drug-seeking behavior as well as the incubation of this behavior following protracted abstinence has become the gold standard to measure craving and relapse in animals.
Alterations in Gene Expression in Drug-Exposed Animals
Microarray studies have revealed that chronic exposure of nicotine increases expression of genes involved in regulation of food intake and energy expenditure as well as it coregulates multiple neurotransmitter systems and pathways involved in protein modification/degradation in rat brain. Our knowledge on how these changes in gene expression contribute toward nicotine addiction is substantially limited because nicotine was administered passively in these studies, which do not mimic the situation observed in smokers. No previous study has identified the effects of nicotine in active self-administering animals on brain regions and performed gene expression profiles of such regions. However, we know from a key publication by Jacobs et al. that active drug consumption during intravenous self-administration is a crucial psychological factor directing long-term genomic responses in the brain, especially in the nucleus accumbens shell. Therefore, it will be crucial to establish gene expression profiles in a triad design from animals that actively self-administer nicotine in direct comparison to yoked nicotine and saline control animals. These kinds of studies usually result in a huge database on gene expression profiles in animals and it will be essential to combine this genomic information with datasets deriving from human genetic studies by a convergent translational genomics approach.
Convergent Translational Genomics Approach for Addictive Behavior
Convergent translational genomics approaches integrate genomic information (e.g., from microarray analysis) from animal models with a candidate gene or genome-wide association study approach in humans. Especially the explanatory power of genetic findings is enhanced by such a convergent approach and as a result a priority gene list for functional validation is obtained. Recently a convergent translational genomics approach was successfully applied to alcohol addiction. In this study, genome-wide association study data from a huge case-control sample for alcoholism were combined with massive information from gene expression profiling in alcohol addicted rats. The genome-wide association studies produced approximately 100 single nucleotide polymorphisms with nominal p < 10 −4 . These, together with 20 additional single nucleotide polymorphisms from genes showing differential expression in rats, were genotyped in a large replication sample. Fifteen single nucleotide polymorphisms showed significant association (two single nucleotide polymorphisms met genome-wide significance) with the same allele as in the genome-wide association studies. Eight of the 15 genes derived from the animal data, demonstrating that relevant genes would have been lost by a mere genome-wide association study approach. In response to this study, the Integrative Neuroscience Initiative on Alcoholism has announced that one of their major aims is to integrate animal data into data obtained from studies in humans with alcoholism ( www.scripps.edu/cnad/inia/structure.html ). In summary, this novel translational tool results in a priority gene list that has to be functionally validated.
Reverse Genetic Approaches for the Functional Validation of Candidate Genes
Reverse genetics are used to assess the role of a candidate gene in a specific behavior, for example, in nicotine self-administration. The most common reverse genetic approach is the generation of a conventional mouse knockout model and its subsequent behavioral analysis. However, the generation of a conventional knockout model is time consuming, cost-intensive, and has no tissue specificity, and because the gene is ablated early in development, numerous compensatory mechanisms may ensue. Through more advanced techniques such as Cre/loxP and tetracycline-inducible systems, a gene of interest can be expressed or inactivated in a tissue-specific and time-controlled manner. Although those conditioned knockout models are of very high value for the neuroscience community they still do not provide a good rational for large-scale functional validation of candidate genes because there is still an enormous effort to generate those model. As an alternative, the use of viral vectors for gene delivery offers many advantages for rapid functional validation studies. In particular, the advent of adeno-associated virus vectors carrying cDNA for—or short hairpin RNA against—specific genes allows for the first time the rapid bidirectional manipulation of gene function.
New Vistas on the Molecular Level
Hallmarks in drug abuse research were the discoveries of endogenous primary target systems. Thus drugs of abuse act on specific receptors, channels, or transporters within the brain to produce their psychoactive and reinforcing effects: for example, opiates act mainly on μ-opioid receptors, cannabis products such as Δ 9 -tetrahydrocannabinol act mainly on cannabinoid receptor 1 receptors, nicotine acts on the nicotinic acetylcholine receptor, psychostimulants such as cocaine act mainly via monoaminergic transporters, and hallucinogens such as lysergic acid diethylamide act via the serotonin-2A receptor —to name the primary targets of the most important drug classes. However, how does alcohol affect the functions of the central nervous system—are there any primary sites of action?
Primary Targets of Alcohol in the Central Nervous System
It is only recently that a shift from the so-called lipid theory (i.e., the primary targets of ethanol are membrane lipids) to the protein theory (i.e., the primary targets of ethanol are membrane proteins, especially receptors) took place. Into the 1990s, different lipid theories postulated that alcohol acts via some perturbation of the membrane lipids of central nervous system neurons. In particular, effects on membrane fluidity and disordering of the bulk lipid phase of membranes was originally an attractive hypothesis of alcohol action because it provided a possible mechanism by which alcohol could affect membrane proteins, such as ion channels, via an action on membrane lipids.
There are clear limitations to the lipid theory. First, effects of alcohol on membrane disorder are generally measurable only at alcohol levels well above the pharmacological range (>500 mg/dL blood alcohol levels); these levels are close to the LD 50 of ethanol in humans. Significant effects of membrane disordering on protein function are even more difficult to envision at pharmacologically relevant alcohol concentrations. For example, at very high intoxicating blood alcohol levels associated with loss of consciousness (∼300 mg/dL), there would be only one alcohol molecule per approximately 200 lipid molecules. Second, membrane effects induced by alcohol concentrations exceeding the pharmacological range can be mimicked by an increase in temperature of just a few tenths of a degree Celsius, which clearly does not produce behavioral signs of alcohol intoxication or appreciably alter the function of membrane proteins such as neurotransmitter-gated ion channels. Therefore, the reported effects of alcohol on membrane fluidity and organization seem to be a purely biophysical phenomenon without any relevance for the pharmacological central nervous system effects of alcohol. Taking even more refinements of the lipid theory into consideration it remains unlikely that membrane lipids are the primary targets of alcohol.
The protein theory predicts that alcohol acts specifically on membrane proteins such as receptors and ion channels. The main reason for a shift toward the protein theory comes from findings that alcohol—at concentrations in the 10–20 mM range—directly interferes with the function of several ion channels and receptors. In a key publication, David Lovinger and colleagues showed that N -methyl- d -aspartate function was inhibited by ethanol in a concentration-dependent manner over the range of 5–50 mM, a range that also produces intoxication. The amplitude of the N -methyl- d -aspartate–activated current was reduced 61% by 50 mM ethanol. What is more, the potency for inhibition of the N -methyl- d -aspartate–activated current by several alcohols is linearly related to their intoxicating potency. This suggests that ethanol-induced inhibition of responses to N -methyl- d -aspartate receptor activation may contribute to the neural and cognitive impairments associated with intoxication. But how can ethanol directly interfere with N -methyl- d -aspartate receptor function?
The N -methyl- d -aspartate receptor is a ligand-gated ion channel with a heteromeric assembly of NR1, NR2 (A-D), and NR3 subunits. The NR1 subunit is crucial for channel function, the NR2 subunits contain the glutamate binding site, and the NR3 subunits have some modulatory function on channel activity, especially under pathological conditions. Electrophysiological studies show that ethanol interacts with domains that influence channel activity, suggesting that residues within transmembrane domains may be involved. In the search for a possible binding site of alcohol at the N -methyl- d -aspartate receptor, several site-directed mutagenesis studies were performed and putative binding sites in the transmembrane 3 and 4 of the NR1 and NR2A subunit, respectively, were identified.
It is not yet possible to directly measure the binding of an ethanol molecule to the N -methyl- d -aspartate receptor by means of physical methods because ethanol is a small molecule with low binding energy being efficient only in the mid-millimolar range. These pharmacological characteristics preclude a direct assessment of an ethanol protein binding site. However, with the discovery of the LUSH protein in the fruit fly Drosophila melanogaster it became possible to model how transmembrane residues can form a specific protein-binding pocket for ethanol. The high-resolution crystal structures of LUSH in complex with a series of short-chain alcohols were obtained by the team of David Jones in 2003. The structure of LUSH reveals a specific alcohol-binding site. LUSH exists in a partially molten globule state. The presence of ethanol at pharmacologically relevant concentrations <50mM shifts the conformational equilibrium to a more compact state, demonstrating that ethanol induces a conformational change of the binding protein—an important requirement for a functional binding site. A group of amino acids form a network of concerted hydrogen bonds between the protein and the ethanol molecules provide a structural motif to increase alcohol-binding affinity at this site. This motif seems to be conserved in a number of mammalian ligand-gated ion channels, and it is therefore suggested that the alcohol-binding site in LUSH represents a general model for putative alcohol binding sites in proteins such as the N -methyl- d -aspartate receptors.
Taken together it has been demonstrated over the last 20 years that ethanol acts directly on the N -methyl- d -aspartate receptor. However, direct interactions have been also described with γ-aminobutyric acid A receptor, serotonin-3, glycine and nicotinic acetylcholine receptors, as well as with several ion channels such as L-type Ca 2+ channels, where concentrations as low as 1 mM produce alterations in the function of these receptors and ion channels. This modern view on selective primary targets of alcohol in the central nervous system has so far not been implemented well into the general knowledge of drug abuse researchers and neuroscientists. Actually, most researchers still consider alcohol as a “dirty drug” with an undefined mode of action. In the future it will therefore be important to better define the putative binding sites for ethanol in the central nervous system. In particular, the findings on LUSH have to be translated to the mammalian brain.
Agonist-Directed Trafficking of Receptor Stimulus—A Key for Understanding Drug Action
A gain of knowledge in structural biology will not only be essential to define the molecular mode of action of the ethanol molecule but also for other drugs of abuse. This is best exemplified by the mode of action of lysergic acid diethylamide on the serotonin-2A receptor. The demonstration that lysergic acid diethylamide and other hallucinogenic compounds elicit their psychoactive effects via serotonin-2A receptor activation has generated a fundamental paradox in a way that not all serotonin-2A receptor agonists exhibit hallucinogenic activity. Indeed, nonhallucinogenic compounds such as lisuride and ergotamine share significant structural similarities and comparable agonist activities at this receptor, but they lack psychoactive properties. This pharmacological paradox has been resolved recently by demonstrating that hallucinogenic versus . nonhallucinogenic compounds, although acting at the same binding site, elicit different patterns of signaling that are responsible for their different behavioral activities. But how can such a divergent effect been explained in view of the standard pharmacological model of G-protein–coupled receptor activation. The ternary complex model postulates a conformational change from an inactive to an active state following agonistic activation. However, both theory and experimental evidence suggest that G-protein–coupled receptors adopt multiple conformations when activated by different agonists. Thus an advanced model of agonist-directed trafficking of receptor stimulus has recently been proposed by Kenakin. This expanded version of the ternary complex model posits that different receptor agonists stabilize distinct conformations that preferentially recruit and activate specific signaling pathways. The fact that serotonin-2A agonists can activate different signaling pathways is consistent with such an expanded version of the ternary complex model because it explains how distinct cellular responses could be produced by agonists acting at the same binding sites. Paradoxical effects on the receptor level have been also observed with other drugs of abuse (e.g., opioids); progress in structural biology and the application of the new concept of agonist-directed trafficking of receptor stimulus will not only be helpful for a better understanding of the molecular action of alcohol and drugs of abuse but will be also important for gaining better insight into the molecular processes underlying drug-induced synaptic plasticity.
New Vistas in Alcohol- and Drug-Induced Synaptic Plasticity
A ubiquitous property of all synapses is their ability to undergo activity-dependent changes in synaptic plasticity that can be studied most effectively using electrophysiological methods in brain slices. Because these slices only remain viable for several hours, the cellular mechanisms underlying the first few hours of long-term potentiation and long-term depression are the best understood. It has been suggested that synaptic plasticity within the mesolimbic dopaminergic system and associated limbic structures, including the extended amygdala, becomes manifest following drug exposure. Some key publications on drug-induced adaptations in the mesolimbic system have revealed that glutamatergic synapses on dopamine neurons in the ventral tegmental area in particular undergo plastic changes following administration of drugs of abuse including ethanol.
By increasing synaptic strength, facilitating long-term potentiation, or blocking long-term depression, drugs of abuse augment the responsiveness of dopamine neurons to glutamate and ultimately promote enhanced dopamine release in brain areas such as the nucleus accumbens and the prefrontal cortex. Drug-induced synaptic strengthening in dopamine neurons in the ventral tegmental area is associated with changes in alpha-amino-3-hydroxy-5-methylisoxazole-4-propionic acid (AMPA) receptor subunit composition. Incorporation of the AMPA receptor subunit GluR1 promotes drug-induced synaptic strengthening, probably through the formation of highly conductive, Ca 2+ permeable GluR1 homomeric AMPA receptors, whereas insertion of GluR2-containing receptors reverts it. Synaptic recruitment of GluR1 subunits and the resultant synaptic potentiation require the activation of N -methyl- d -aspartate receptors. These synaptic changes in dopamine neurons are thought to be related to the development of drug-induced reinforcement processes (see also Engblom et al. ).
In conclusion, drug-induced synaptic plasticity has been found in the ventral tegmental area—nucleus accumbens projection as well as in other brain areas of the extended amygdala. However, the generally held view that these cellular adaptations underlie drug reinforcement is based on purely associative findings. Direct experimental evidence for the behavioral significance of these drug-induced synaptic changes involving glutamate receptors is still lacking. Only in vivo electrophysiology in conditional mouse models that selectively lack, for example, N -methyl- d -aspartate receptors in dopaminergic neurons will provide a clear answer as to whether AMPA/ N -methyl- d -aspartate receptor–induced synaptic strengthening of dopamine neurons within the ventral tegmental area serves as a cellular model for the induction of drug reinforcement. It will be important also to know whether these drug-induced synaptic changes modulate neuronal network activity. For answering this question, multielectrode recording and ultra-high-field imaging in rodents will be useful.
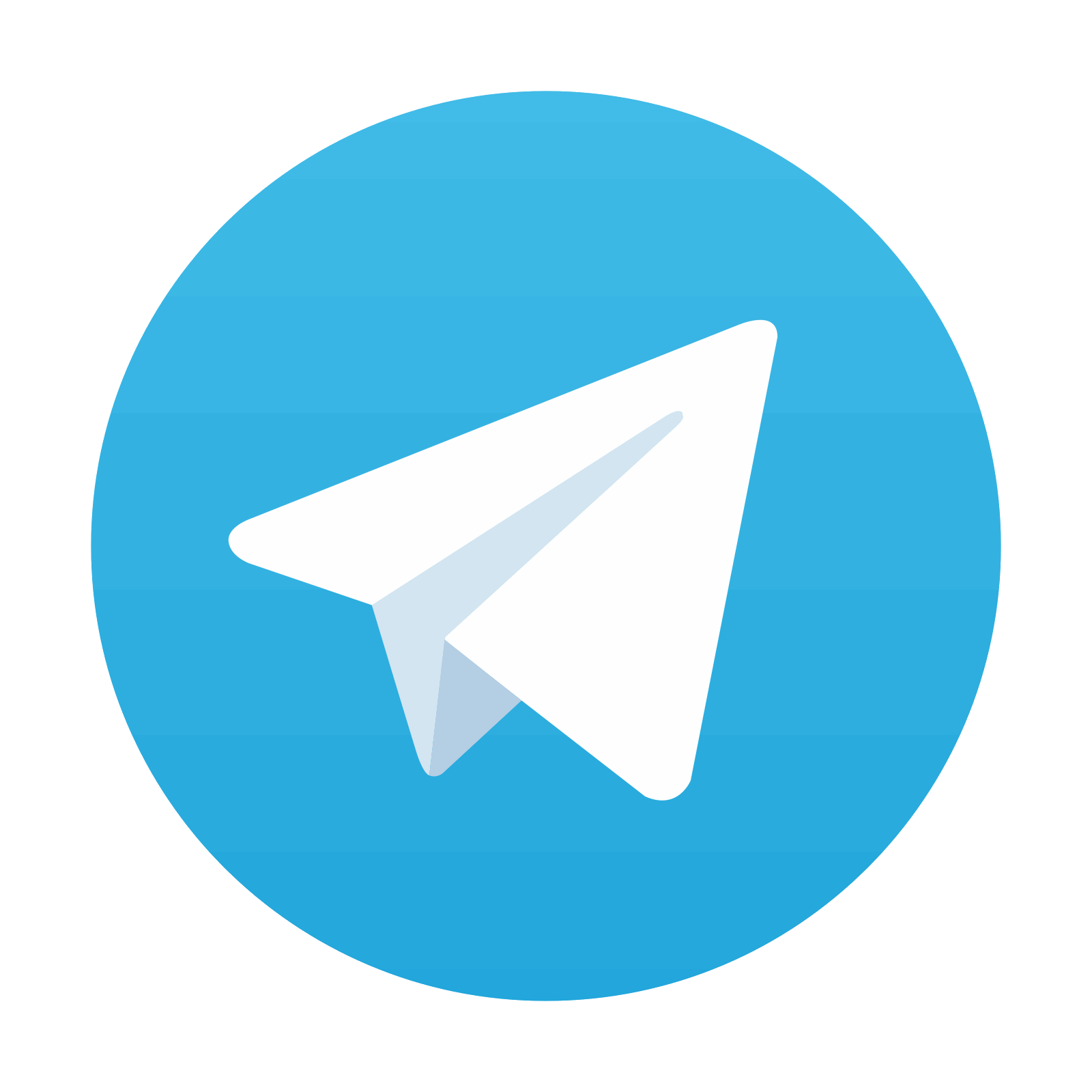
Stay updated, free articles. Join our Telegram channel

Full access? Get Clinical Tree
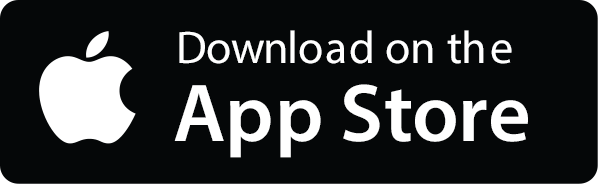
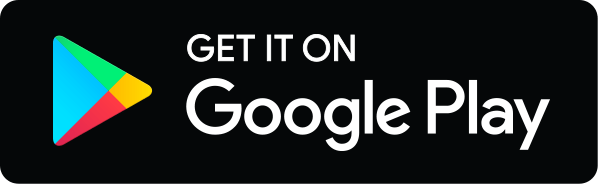