Limbs begin as protrusions of proliferating cells, the limb buds, along the lateral edge of the mesoderm of the human embryo in the fourth week of development. The location of each limb bud along the anterior-posterior axis of the embryo (head-to-tail axis) is associated with the expression of a specific transcription factor at each location, Tbx4 for the hindlimbs and Tbx5 for the forelimbs, whose expression is induced by various combinations of fibroblast growth factor ligands. Thus the primarily proliferative process of limb bud outgrowth is activated by growth factors and transcription factors.
The limb bud grows primarily in an outward, lateral expansion of the proximal-distal axis of the limb (see Fig. 14-18B). Whereas proximal-distal expansion of the limb is the most obvious process, the two other axes are established soon after the onset of limb bud outgrowth. The anterior-posterior axis is set up soon after limb bud outgrowth, with the thumb considered to be an anterior structure, because it is on the edge of the limb facing the upper body. The fifth finger is a posterior structure because it is on the side of the limb bud oriented toward the lower part of the body. During limb formation, the morphogen SHH is expressed in the posterior aspect of the developing limb bud, and its expression level forms a gradient that is primarily responsible for setting up the anterior-posterior axis in the developing limb (see Fig. 14-18B). Defects in anterior-posterior patterning in the limb cause excessive digit patterning, manifested as polydactyly, or failure of complete separation of developing digits, manifested as syndactyly. The dorsal-ventral axis is also established, resulting in a palm or sole on the ventral side of the hand and foot, respectively.
One can now begin to understand the mechanisms underlying birth defect syndromes by applying knowledge from molecular developmental biology to human disorders. For example, mutations in the GLI3 transcription factor gene cause two pleiotropic developmental anomaly syndromes, the Greig cephalopolysyndactyly syndrome (GCPS) and the Pallister-Hall syndrome (see Fig. 14-1). These two syndromes comprise distinct combinations of limb, central nervous system, craniofacial, airway, and genitourinary anomalies that are caused by perturbed balance in the production of two variant forms of GLI3, referred to as GLI3 and GLI3R, as shown in Figure 14-24. GLI3 is part of the SHH signaling pathway. SHH signals, in part, through a cell surface receptor encoded by a gene called PTCH1, which is concentrated in the cilium of cells during development. Mutations in PTCH1 cause the nevoid basal cell carcinoma syndrome. Also known as Gorlin syndrome, this syndrome comprises craniofacial anomalies and occasional polydactyly that are similar to those seen in GCPS, but in addition, Gorlin syndrome manifests dental cysts and susceptibility to basal cell carcinoma. By considering Gorlin syndrome and GCPS, one can appreciate that the two disorders share phenotypic manifestations precisely because the genes that are mutated in the two disorders have overlapping effects in the same developmental genetic pathway. A third protein in the SHH signaling pathway, the CREB-binding protein, or CBP, is a transcriptional coactivator of the GLI3 transcription factor. Mutations in CBP cause the Rubinstein-Taybi syndrome (see Fig. 14-5), which also shares phenotypic manifestations with GCPS and Gorlin syndrome.
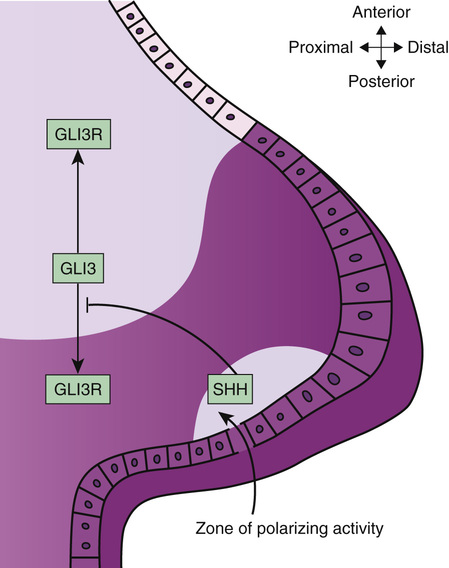
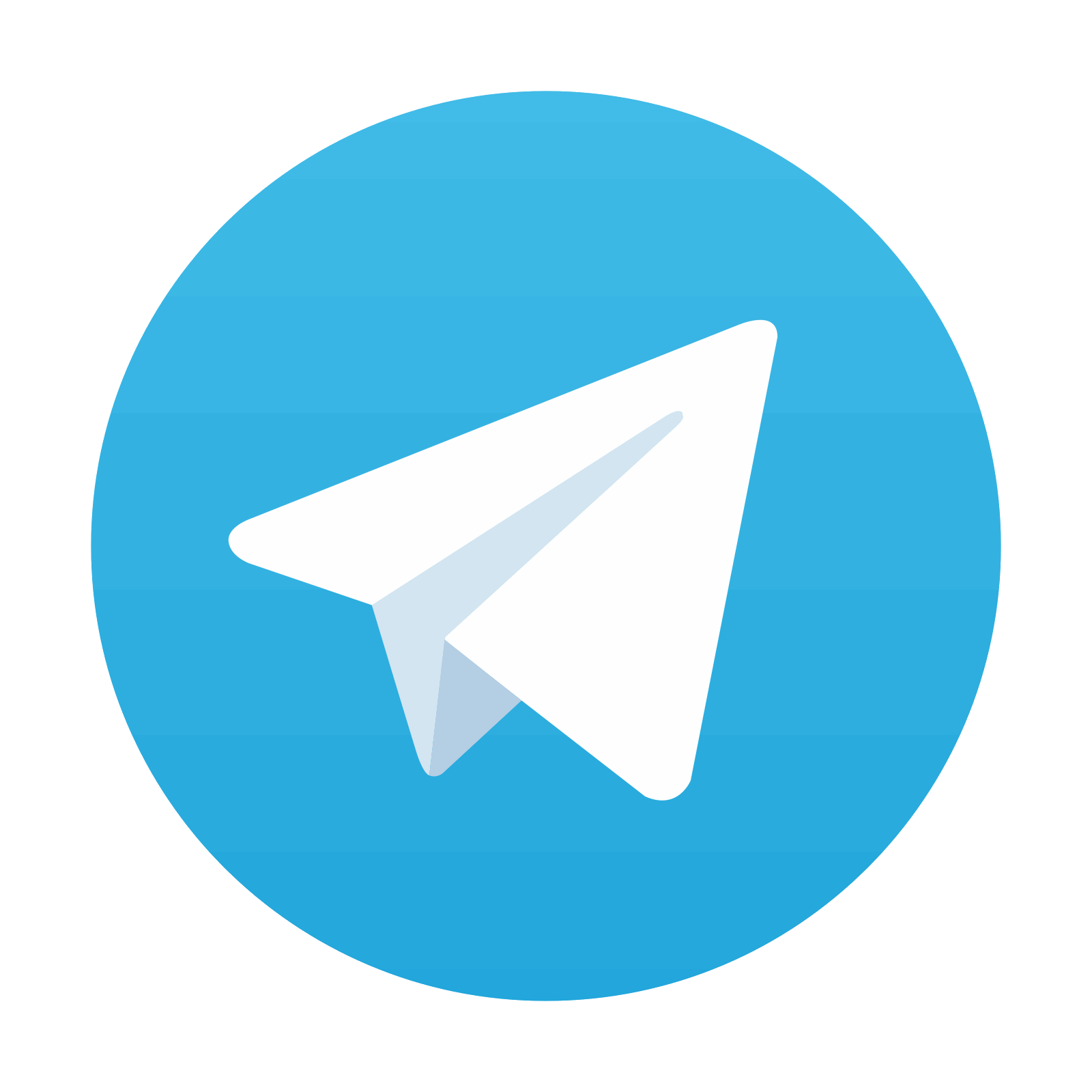
Stay updated, free articles. Join our Telegram channel

Full access? Get Clinical Tree
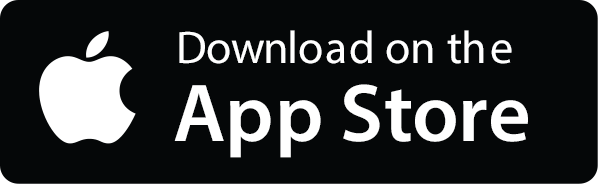
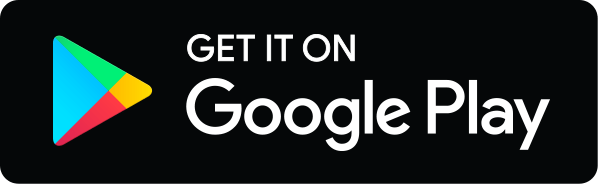