and Jürgen Roth2
(1)
Medical University of Vienna, Vienna, Austria
(2)
University of Zurich, Zurich, Switzerland
Architecture of the Cell Nucleus
The nucleus is the largest of the cellular compartments, housing the chromosomes with the vast majority of the cellular genome and multiple molecular machineries necessary for gene organization and expression. In recent years, contemporarily with the successful sequencing of the entire human genome, knowledge of nuclear organization has also enormously increased and exciting results provide evidence that the long-standing dogma of a spatial separation of gene transcription in the nucleus and translation in the cytoplasm cannot be maintained. Advanced methodical approaches provide insight into the three-dimensional nuclear architectures and their dynamics and several nuclear structures have been proved to be multifunctional and, although morphologically stable, highly dynamic entities.
The nuclear space is separated from the cytoplasm by the double membrane of the nuclear envelope (NE), in which the nuclear pores are embedded, forming routes for nuclear-cytoplasmic exchange (arrows in panel A, cf. Figs. 11, 12, and 13). Panel B shows the outer and inner membranes enclosing the perinuclear cistern and a connection site, where the outer membranes of the nuclear envelope are continuous with those of the rough endoplasmic reticulum (RER) and the perinuclear cistern passes into the RER lumen (asterisk).
The nucleus is compartmentalized in chromosome territories and interchromatin spaces. Genomic DNA condensed in chromosomes during mitosis forms chromosome territories in the interphase nucleus. Genetic loci shown on mitotic chromosomes are positioned in the interphase nucleus within or, presumably, on decondensed chromatin fiber loops, outside the territories.
In the electron microscope, due its intense staining, the condensed chromatin (heterochromatin, C) is clearly visible, whereas the decondensed euchromatic fibers are hardly discernible. Condensed chromatin dominates the peripheral nuclear areas corresponding to its attachment to the nuclear lamina (cf. Fig. 13). In the nucleus interior, condensed chromatin cords surround the interchromatin spaces, where numerous discrete domains related to gene expression exist. Among these, the nucleolus is the most prominent (Nu, cf. Figs. 7, 8, and 10).
Interchromatin granule clusters (IG) represent the ultrastructural equivalent of the splicing speckles or splicing factor compartments, which are enriched in RNA and protein factors engaged in mRNA splicing. It is suggested that IGs represent sites of storage and/or assembly of splicing complexes. IGs also contain multiple RNA processing factors, transcription factors, and potential structural proteins, such as lamins. Both chromatin proteins and proteins of interchromatin domains reside only temporarily on chromatin and the respective compartments, and there is a continuous exchange with the nucleoplasm.
The Cajal body (CB), first described by Ramon y Cajal a century ago, is the best characterized of the small nuclear bodies. It corresponds to the “coiled body” and was recently renamed after its discoverer. In Cajal bodies, components of transcription and RNA processing machineries are transiently localized (cf. Fig. 10).
References
Cardoso MC, Schneider K, Martin RM, Leonhardt H (2012) Structure, function and dynamics of nuclear subcompartments. Curr Opin Cell Biol 24:79
Cau P, Navarro C, Harhouri K, Roll P, Sigaudy S, Kapsi E, Perrin S, De Sandre-Giovanolli A, Lévy N (2014) Nuclear matrix, nuclear envelope and premature aging syndromes in a translational research perspective. Semin Cell Developm Biol 29:125
Cremer T, Cremer C (2001) Chromosome territories, nuclear architecture and gene regulation in mammalian cells. Nat Rev Genetics 2:292
Derenzini M, Olins AL, Olins DE (2014) Chromatin structure in situ: the contribution of DNA ultrastructural cytochemistry. Eur J Histochem 58:2307
Iborra FJ, Jackson DA, Cook PR (2001) Coupled transcription and translation within nuclei of mammalian cells. Science 293:1139
Lamond AI, Spector DL (2003) Nuclear speckles: a model for nuclear organelles. Nat Rev Mol Cell Biol 4:605
Misteli T (2008) Physiological importance of RNA and protein mobility in the cell nucleus. Histochem Cell Biol 129:5
Pederson T (2002) Dynamics and genome-centricity of inter chromatin domains in the nucleus. Nat Cell Biol 4:E287
Pederson T (2013) The persistent plausibility of protein synthesis in the nucleus. Curr Opin Cell Biol 25:520
Rapkin LM, Anchel DRP, Li R, Bazett-Jones DP (2012) A view of the chromatin landscape. Micron 43:150
Roix J, Misteli T (2002) Genomes, proteoms, and dynamic networks in the cell nucleus. Histochem Cell Biol 118:105
Rouchette J, Genoud C, Vazquez-Nin GH, Kraus B, Cremer Th, Fakan S (2009) Revealing the high-resolution three-dimensional network of chromatin and interchromatin space: A novel electron-microscopic approach to reconstructing nuclear architecture. Chromos Res 17:801


Figure 3
Magnification: ×24,000 (A), ×106,000 (B)
Variation of Nuclear Shape and Size
The shape and size of the nucleus in interphase can vary depending on the cell type and cellular activity. Nuclear shape and functional changes depend on nuclear lamins and on microtubules, while nuclear size seems to be primarily determined by the cytoplasmic volume, the karyoplasmic ratio. On the other side, the nucleoskeletal theory maintains that the genome size is a determining factor for nuclear size.
The large polygonal hepatocytes contain a large, round nucleus (cf. Fig. 122), whereas cylindrical absorptive intestinal cells have an oval nucleus, and mucus-producing cells such as goblet cells have a flatter nucleus (cf. Fig. 115). Both endothelia and white adipocytes contain extremely flat nuclei (cf. Figs. 146 and 164). As a general rule, cell shape and nuclear shape appear to be related to each other. Notable exceptions are round neutrophilic and basophilic granulocytes that contain a multilobed nucleus (cf. Figs. 190 and 192). The nucleus of neutrophilic granulocytes and of lymphocytes undergoes dramatic reversible changes in shape during extravasation when the cells squeeze through the endothelium of capillaries.
Abnormal nuclear shape can be observed under various pathological conditions. Supporting the claim that nuclear shape depends on lamins, alterations of nuclear shape are commonly observed in diseases caused by lamin mutations, the laminopathies. Dramatic changes in nuclear shape and size as well as in the nucleoplasm, the nuclear matrix, and nuclear bodies are usually associated with cancer. This long-known characteristic phenomenon is of importance for histopathological diagnosis. Although the various nuclear changes mentioned below may occur in a wide range of cancer types, some are found associated with specific kinds of cancer. The electron micrographs present nuclear changes associated with a highly malignant human dendritic cell tumor. In panel A, a large lobed nucleus of a tumor cell is shown that contains enlarged nucleoli (Nu) with nucleolonema (cf. Fig. 8). Although nuclear indentations and folds are common to many cancer types, lobulation is a more restricted phenomenon. The nucleus seen in panel A is >20 μm in size and the karyoplasmic ratio is disturbed because of the larger nuclear volume. Panel B shows another cell from the same malignant tumor that appears bi-nucleated. Again, the large nuclei (>30 μm) are lobed and contain numerous enlarged nucleoli (Nu) with nucleolonema. As commonly observed in cancer cell nuclei, the condensed chromatin (heterochromatin) is dispersed, and coarse heterochromatin aggregates exist. It is now obvious that aberrant nuclear organization, in particular of the chromatin, is associated with altered gene expression in cancer.
References
Dahl KN, Ribera AJ, Limbering J (2008) Nuclear shape, mechanics, and mechanotransduction. Circ Res 102:1307
Hoffmann K et al (2002) Mutations in the gene encoding the lamin B receptor produce an altered nuclear morphology in granulocytes (Pelger-Huet anomaly). Nat Genet 31:410
Hoffmann K, Sperling K, Olins AL, Olins DE (2007) The granulocyte nucleus and lamin B receptor: avoiding the ovoid. Chromosoma 116:227
Neumann FR, Nurse P (2007) Nuclear size control in fission yeast. J Cell Biol 179:593
Scaffidi P, Misteli T (2006) Lamin A-dependent nuclear defects in human aging. Science 312:1059
Schreiber KH, Kennedy BK (2013) When lamins go bad: nuclear structure and disease. Cell 152:1365
Stein GS, Montecino M, van Wijnen AJ, Stein JL, Lian JB (2000) Nuclear structure-gene expression interrelationships: implications for aberrant gene expression in cancer. Cancer Res 60:2067
Webster M, Witkin KL, Cohen-Fix O (2009) Sizing up the nucleus: nuclear shape, size and nuclear-envelope assembly. J Cell Sci 122:1477
Zink D, Fischer AH, Nickerson JA (2004) Nuclear structure in cancer cells. Nat Rev Cancer 4:677


Figure 4
Magnification: ×1,000 (A); ×800 (B)
Cytochemical Detection of Ribonucleoproteins
Various cytochemical techniques are available to study the distribution of DNA and RNA by electron microscopy. A classic technique for the detection of nuclear RNP RNA-containing structures in ultrathin sections is the regressive contrasting procedure of Bernhard. In a first step, both DNA and RNA in Epon ultrathin sections of a tissue or cells are contrasted with uranyl acetate. Afterwards, the ultrathin sections are incubated with aqueous EDTA, which forms complexes with uranyl ions, resulting in the gradual removal of uranyl ions from DNA. The staining is of limited specificity for RNA and needs to be verified by enzyme digestion controls.
An example of the regressive RNA contrasting procedure is given in the micrograph. It shows an Epon ultrathin section from glutaraldehyde-fixed rat liver. In the nucleus of hepatocytes, the EDTA differential staining preferentially contrasts ribonucleoprotein-containing nuclear constituents. Perichromatin fibrils (arrowheads) are now visible in such a specimen, whose contrast after a conventional staining of sections would be too close to that of dispersed chromatin fibers. Arrows point to some perichromatin granules considered to be nuclear storage and/or the transport form of pre-mRNA particles. The DNA-containing peripheral and nucleolus-associated condensed chromatin (C) exhibits only light gray contrast.
IG: interchromatin granules; Nu: nucleolus
References
Bernhard W (1969) A new staining procedure for electron microscopical cytology. J Ultrastruct Res 27:250
Gautier A (1976) Ultrastructural localization of DNA in ultrathin tissue sections. Int Rev Cytol 44:113
Moyne G (1980) Methods in ultrastructural cytochemistry of the cell nucleus. Progr Histochem Cytochem 13:1


Figure 5
Magnification: ×24,500
Detection of Sites of DNA Replication and of Interphase Chromosome Domains
DNA can be detected with high precision and specificity by immunoelectron microscopy. For this purpose, antibodies raised experimentally or present in sera from patients who have autoimmune diseases and antibodies raised against nucleotide analogs have been applied. Formaldehyde or formaldehyde/glutaraldehyde fixation and embedding in Lowicryl K4M or LR white resins provides high detection sensitivity. High spatial resolution is obtained by the use of gold-labeled secondary antibodies. With these techniques, DNA has been observed consistently in the dispersed and condensed chromatin of interphase nuclei, chromosomes of mitotic cells, and mitochondria.
In panels A and B, DNA has been revealed by the incubation of living V79 hamster cells with the thymidine analog bromodeoxyuridine (BrdU). The BrdU, which becomes incorporated into nuclear DNA during its replication, can be detected subsequently in ultrathin sections by a monoclonal anti-BrdU antibody and gold-labeled secondary antibody.
Panel A shows part of the nucleus of a cell that was incubated with BrdU for as short a period as 2 min. Sites of nascent DNA as detected with anti-BrdU antibody are indicated by the presence of gold particles. In addition, DNA in the Lowicryl K4M thin section was histochemically revealed by a Feulgen-type reaction using osmium amine. Immunogold labeling showed DNA replication sites in association with individual dispersed chromatin fibers (arrowheads) on the periphery of a condensed chromatin area (arrow). In other experiments, these regions were shown to contain DNA polymerase alpha. Together with results from 5 min BrdU pulse, alone or followed by a chase, it could be concluded that the sites of DNA replication corresponded essentially to perichromatin regions and that the newly replicated DNA moved rapidly from the replication sites towards the interior of condensed chromatin areas.
In panel B, V79 hamster cells were incubated with BrdU for 9 h and then allowed to grow for an additional 62 h. This time period allowed for about five cell divisions, during which the labeled chromosomes became segregated. Nuclei of these cells contained only two to three labeled chromosomes and were highly amenable to analyzing the presence of chromosome territories and subchromosomal domains. Such analysis indicated that chromosome domains were either separated from one another by interchromatin space or were in close contact with no or little intermingling of their DNA. Therefore, although chromosomes formed discrete territories, chromatin of adjacent chromosomes seemed to be in contact in limited regions, implying chromosome-chromosome interactions. Such an in vivo labeling approach allowed the analysis of the structural features of interphase chromosome domains and of their relations with the immediate neighborhood. Arrows indicate RNP fibrils in perichromatin and interchromatin regions.
NE: nuclear envelope.
References
Cremer T, Cremer M (2010) Chromosome territories. Cold Spring Harb Perspect Biol 2:a003889
Fakan S (2004) The functional architecture of the nucleus as analyzed by ultrastructural cytochemistry. Histochem Cell Biol 122:83
Ferai C et al (2010) Gene positioning. Cold Spring Harb Perspect Biol 2:a000588
Francastel C, Schubeler D, Martin DI, Groudine M (2000) Nuclear compartmentalization and gene activity. Nat Rev Mol Cell Biol 1:137
Gratzner HG (1982) Monoclonal antibody to 5-bromo- and 5-iododeoxyuridine: a new reagent for detection of DNA replication. Science 218:474
Jaunin F, Visser AE, Cmarko D, Aten JA, Fakan S (2000) Fine structural in situ analysis of nascent DNA movement following DNA replication. Exp Cell Res 260:313
Liu DF, el-Alfy M, Leblond CP (1995) DNA changes involved in the formation of metaphase chromosomes, as observed in mouse duodenal crypt cells stained by osmium-ammine. II. Tracing nascent DNA by bromodeoxyuridine into structures arising during the S phase. Anat Rec 242:449
Tamatani R, Taniguchi Y, Kawarai Y (1995) Ultrastructural study of proliferating cells with an improved immunocytochemical detection of DNA-incorporated bromodeoxyuridine. J Histochem Cytochem 43:21
Thiry M (1999) Ultrastructural methods for nucleic acid detection by immunocytology. Progr Histochem Cytochem 34:93


Figure 6
Magnification: ×66,000 (A); ×63,000 (B)
Nucleolus
Nucleoli are the most prominent of the nuclear bodies. They are formed around the nucleolus organizer regions (NORs), which are localized to the secondary constrictions of acrocentric chromosomes and bear numerous copies of genes coding for the pre-ribosomal RNA (pre-rRNA). Production of ribosome precursors is the main task of nucleoli, although it is neither a privilege, nor is it the sole task, inasmuch as pre-ribosomal particles may also be built in the nucleus outside of nucleoli and, during the past decade, various additional functions have been ascribed to nucleoli.
In human cells, NORs are contained in five chromosomes. Hence in diploid cells, theoretically ten nucleoli could be organized, but nucleoli tend to fuse during interphase and mammalian cells show mostly one to four nucleoli.
The morphological appearance of nucleoli as shown electron microscopically in this figure and illustrated in the insert at the left lower corner reflects their main function in ribosome biogenesis. The structure of nucleoli is a result of the processes connected with transcription and processing of pre-rRNA and assembly of precursors of the small and large ribosome subunits. The nucleolar structure changes concomitantly with cell differentiation (cf. Fig. 8) and disappears with onset of mitosis, at which time transcription and processing of pre-rRNA are suppressed.
Nucleoli are composed of three different components, the dense fibrillar component (dfc), the granular component (gc), and the fibrillar center (fc), and they are accompanied by masses of condensed chromatin (C), which mainly correspond to chromosome territories of NOR-containing chromosomes (cf. Fig. 3). The fibrillar center, which is assumed to be a protein storage site, appears as a distinct spherical body encircled by the dense fibrillar and granular components. These compartments each contain growing pre-ribosomal particles, the dense fibrillar component in the early state and the granular component at late states of formation. The dense fibrillar component is enriched in newly synthesized pre-rRNA and a palette of proteins, the granular component consists of pre-ribosomal particles, which are nearly completed. Transcription is proposed to take place in the dense fibrillar component or at the border between the fibrillar center and the dense fibrillar component. In another model, the fibrillar center is suggested to be the main site of transcription.
The relation between nucleoli and Cajal bodies is close (cf. Figs. 3 and 10, CBs). CBs are important for the biogenesis and function of small nucleolar ribonucleoproteins. Several proteins, such as fibrillarin and Nopp40, are localized in both the nucleolus and the CB. There is also evidence that proteins move between the two bodies. CBs are found in direct contact with nucleoli and they are highly dynamic structures that have been shown to travel toward and away from nucleoli. Because CBs also have been shown to move from the nucleolar periphery into the nucleolus, it is possible that transfer of the material takes place by direct contact between CBs and nucleoli.
In recent years, additional nucleolar functions not related to the assembly of pre-ribosomes have been assumed. These include tRNA maturation, sequestration of regulatory molecules, roles in viral infection, export of viral RNAs, and control of aging. It has been proposed that, for some of the nonconventional activities, an immobile platform is necessary and thereby advantage is taken of the nucleolus.
References
Filipowicz W, Pogacic V (2002) Biogenesis of small nucleolar ribonucleoproteins. Curr Opin Cell Biol 14:319
Gall JG (2003) The centennial of the Cajal body. Nat Rev Mol Cell Biol 4:975
Hozak P (1995) Catching RNA polymerase I in flagranti: Ribosomal genes are transcribed in the dense fibrillar component of the nucleolus. Exp Cell Res 216:285
Olson MOJ, Hingorani K, Szeneni A (2002) Conventional and nonconventional roles of the nucleolus. Int Rev Cytol 219:199
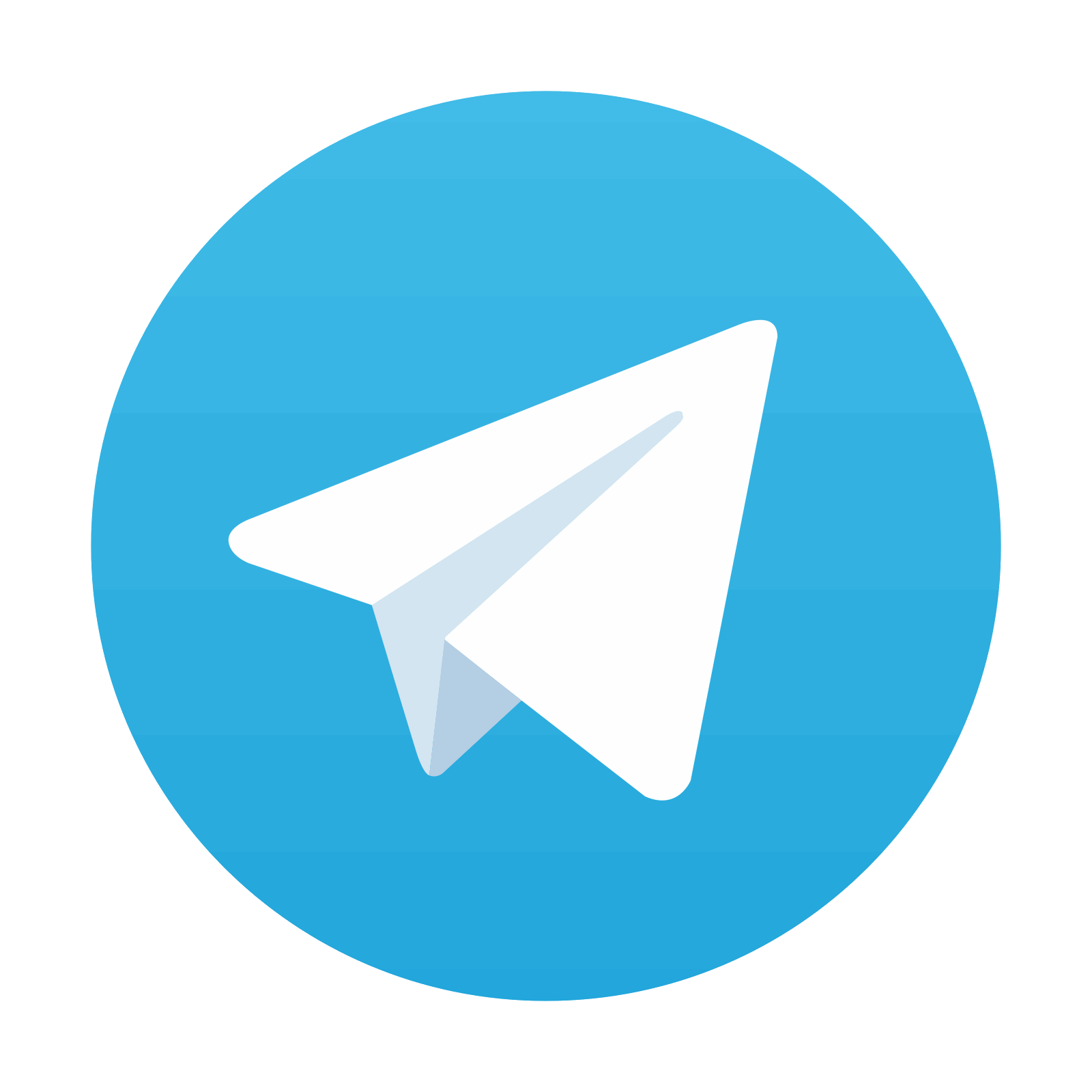
Stay updated, free articles. Join our Telegram channel

Full access? Get Clinical Tree
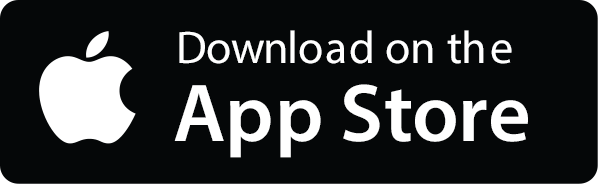
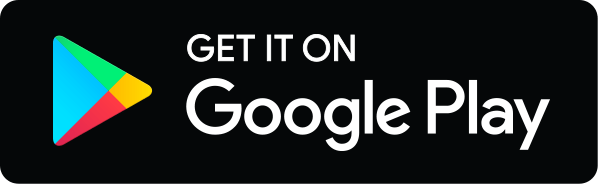