chapter 6 Non-catalytic proteins: providers of structure and functionality







In Chapter 5 the organisation of chains of amino acids into secondary and tertiary structures was described. These structural elements can be arranged in a large number of combinations to produce a structurally diverse series of proteins. However, the majority of these combinations can be classified into two main groups; globular and fibrous proteins.
Globular proteins
The haem group
The role played by the haem group depends on the environment created by folding the protein around it, and includes catalysis (acceleration of chemical reactions), electron transfer, nitric oxide transport and oxygen transport and storage. The most abundant haem proteins in humans and other mammals are myoglobin and haemoglobin, both of which use their haem groups to reversibly bind oxygen. Haem has a planar ring structure with a central ferrous iron (Fe2+) held in place by bonds to four nitrogens in the ring (Fig 6-1). The planar nature of the ring allows the iron to form two further bonds, one above the ring and one below it. In myoglobin and haemoglobin one of these bonds is to the side chain of a histidine residue and the other allows a reversible interaction with oxygen.
Myoglobin
Myoglobin functions as an oxygen carrier and oxygen reservoir in cardiac and skeletal muscle. It consists of a single polypeptide that is tightly folded with about 80% of it in the form of α-helices (Fig 6-2). Each stretch of α-helix is terminated by the presence of a proline residue or by a bend or loop stabilised by ionic or hydrogen bonding. The interior of the molecule is mainly composed of tightly-packed nonpolar amino acids to form a hydrophobic core. In contrast the charged residues are located on the surface, allowing them to hydrogen bond with each other and with water. The haem group is located in a cleft largely made from hydrophobic amino acids. However, there are two histidine residues included in the cleft which are involved in the binding of oxygen to the ferrous iron in the haem group. This process is called oxygenation which is different from oxidation (the loss of electrons).
Haemoglobin
Haemoglobin is only found in erythrocytes, with its main function being to collect oxygen from the lungs and deliver it to the tissues. The main form of haemoglobin in adults (HbA) consists of four polypeptide chains held together by non-covalent bonding to generate a tetrameric quaternary structure (Fig 6-3). There are two types of peptides involved, α-globin and β-globin. Each globin polypeptide folds to generate a structure similar to myoglobin, with stretches of α-helix and hydrophobic residues buried in a central hydrophobic core. However, some hydrophobic residues are exposed on the surface to allow an α-globin to interact with a β-globin by hydrophobic interactions to form a dimeric structure. Each globin also has a hydrophobic haem-binding cleft similar to that in myoglobin.
The relaxed (R) form—the binding of oxygen to the T form disrupts some of the ionic and hydrogen bonds between the two dimers allowing the peptides more freedom of movement. This results in a higher affinity for oxygen resulting in the oxygenated state. The binding of oxygen to one haem group increases the affinity for oxygen of the others. This ‘communication’ between the subunits results in the phenomenon referred to as cooperative binding, where the binding of a molecule affects the subsequent binding of other molecules (Fig 6-4). Haemoglobin must bind oxygen efficiently in the lungs and release oxygen in other body tissues. Haemoglobin in the R state has a high affinity for oxygen, which is useful when in the lungs but less so in body tissues in which oxygen needs to be released. Haemoglobin in the T state has a low affinity for oxygen, allowing for efficient release in body tissues but inefficient uptake in the lungs. This problem is solved by haemoglobin making a transition from a low affinity T state to a high affinity R state as more oxygen molecules are bound.
Fetal haemoglobin (HbF) is the main oxygen transport protein in the fetus during the last seven months of development in the uterus but is nearly completely replaced by HbA by approximately 12 weeks after birth. The structure of HbF is similar to that of HbA in that it is made from four subunits, each of which carries a haem group. However, whereas HbA consists of two α- and two β-subunits, the β-subunits are replaced by γ-subunits in HbF. These γ-subunits have a higher affinity for oxygen and thus make HbF better able to bind oxygen, giving the developing fetus better access to oxygen from the placental blood supply.
Immunoglobulins
Immunoglobulins (abbreviated to Ig and also known as antibodies) are large (∼150kDa) globular proteins that are found in the blood and body fluids of vertebrates, or attached to the surface of specialised immune cells. They are used by the immune system to recognise and then neutralise foreign material such as bacteria and viruses. Immunoglobulins exist as a family of proteins made from a basic structural unit of two large heavy chains and two small light chains. This basic unit can then be used to form monomers, dimers or pentamers. They are made by a specialised type of white blood cell called B lymphocytes or B cells. There are five different kinds (isotypes or classes) of antibodies which are based on the use of different types of heavy chain (Table 6-1
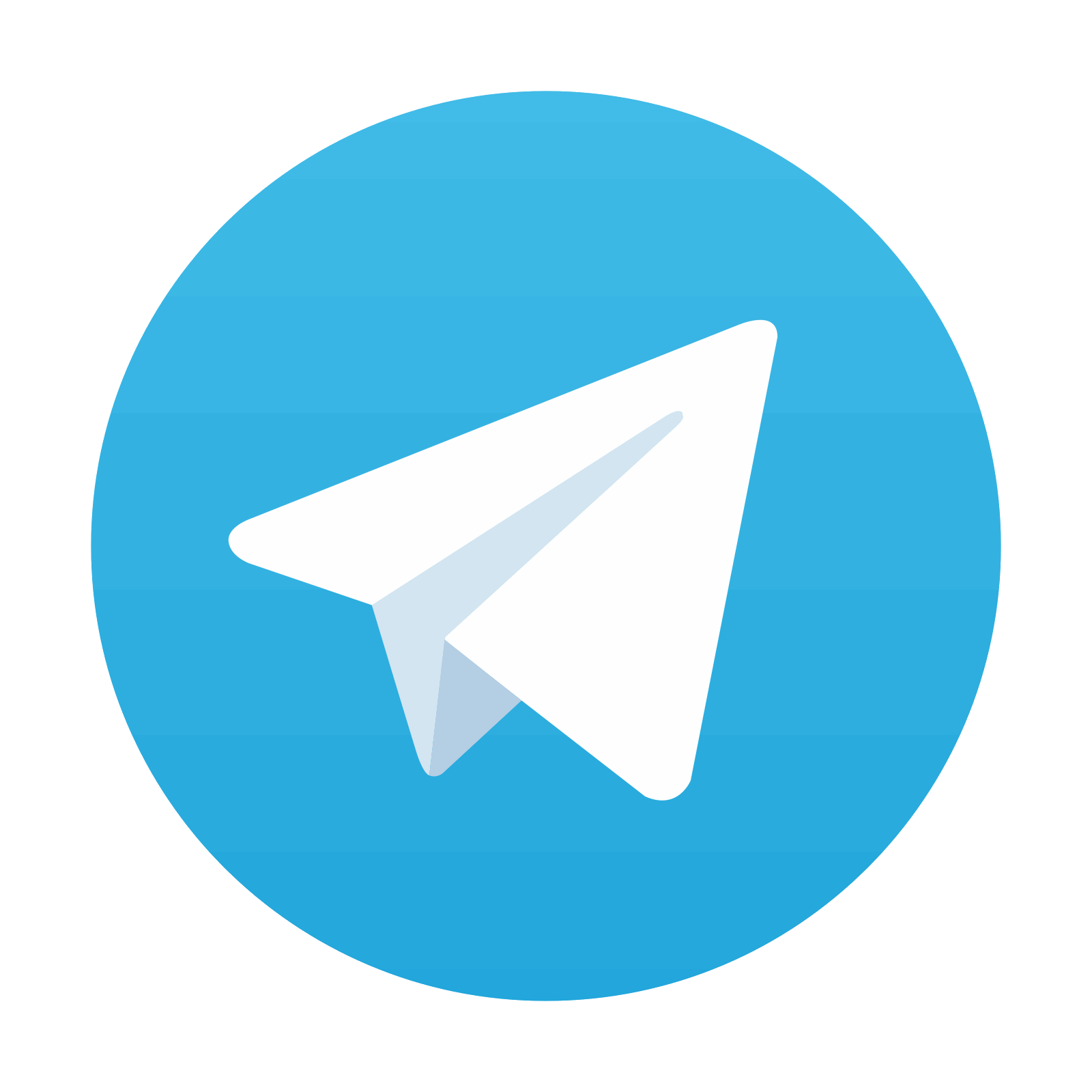
Stay updated, free articles. Join our Telegram channel

Full access? Get Clinical Tree
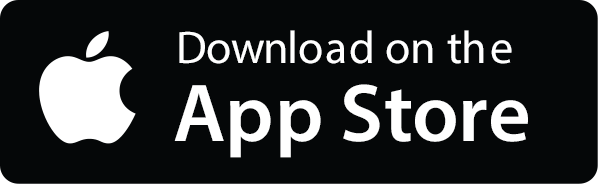
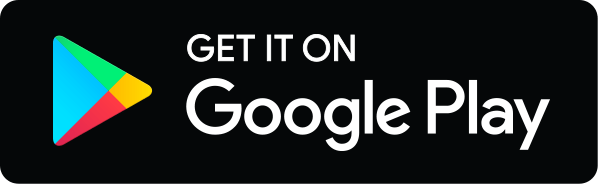