Fig. 2.1
The isoprenoid biosynthesis pathway-based opening/closing carbon flux strategies aiming at ART overproduction in A. annua L. ABA abscisic acid; DMAPP dimethylallyl diphosphate; DXP deoxyxylulose 5-phosphate; DXR DXP reductoisomerase; DXS DXP synthase; FPP farnesyl pyrophosphate; GGPP geranylgeranyl pyrophosphate; HMG-CoA 3-hydroxy-3-lmethylglutaryl coenzyme A; HMGR HMG-CoA reductase; G3P glyceraldehyde 3-phosphate; GPP geranyl pyrophosphate; IPP isopentenyl pyrophosphate; MEP methylerythritol 4-phosphate; MVA mevalonic acid; SS squalene synthase
We introduced an antisense squalene synthase gene (asSS) into the genome of A. annua L., and observed a well correlation of low-level SS mRNA with low-content steroids and high-yield ART. We also obtained enhanced ART production in transgenic A. annua L. plants upon cold stress or storage, accounting for 3.7-fold and 5.2-fold increases of ART content, respectively (Feng et al. 2009). These studies should shed light on the exploration of accelerated and sustainable ART supply.
One of the rate-limiting steps of ART biosynthesis was identified at the final nonenzymatic reaction (Zeng et al. 2009). As a cis element responsive to oxidative stress, the promoter of ADS from A. annua L. was found to be inducible by salicylic acid (SA) and methyl jasmonate (MJ). SA/MJ-treated A. annua L. exhibits a correlation of the upregulation of ADS with the emission of 1O2, suggesting that SA/MJ induces ART overproduction through invoking 1O2 burst (Guo et al. 2010; Zeng et al. 2011). Taking together, these results are implicated in simulating a natural cellular environment to enable an accelerated accumulation of ART. Those achievements should pave a wide path toward a practical solution to currently limited ART supply.
2.2.3 The Pharmaceutical Values and Toxicological Concerns of ART
ART is a potent antimalarial agent that effectively kills chloroquine-resistant Plasmodium falciparum and other multidrug resistant malarial parasite strains. Administration of ART would allow the curation of 90 % malarial patients within 48 h although escaped parasites might recur shortly. A best way to prohibit the recurrence of malaria is to combine the rapidly but shortly effective ART with the long half-life antimalarial drug, such as mefloquine, lumefantrine, amodiaquine, piperaquine, or pyronaridine (Krudsood et al. 2010).
Except for P. falciparum, ART can also kill other helminth parasites, such as Schistosoma japonicum, Clonorchis sinensis, Theileria annulatan, and Toxoplasma gondii. The schistosomes of S. japonicum cause schistosomiasis, another prevalent parasitic infection as the second to malaria. ART and its derivatives are potent anthelmintics (Xiao 2005).
Since the last decade, on the other hand, an early-phase research wave of ART’s anticancer activity has been initiated. We and other authors previously found ART’s significant anticancer effects on human hepatoma cell lines (Chen et al. 2000; Hou et al. 2008). Additionally, ART was also observed to exhibit antiarrhythmic activity (Wang et al. 1998) and antihepatitis B virus activity (Romero et al. 2005). Besides, artemisinic acid was shown to have antibacterial activity (Roth and Acton 1989).
In the cytotoxicity assays of ART and derivatives to Ehrlich ascite tumor cells, Woerdenbag et al. (1994) estimated that the half inhibitory concentrations (IC50) of ART, artesunate, artemether, and arteether are 12.2–29.8 μM, the IC50 of artemisitene is 6.8 μM, and the IC50 of dihydroartemisinin dimmers is 1.4 μM. Later, Zheng et al. (1994) and Jung (1997) determined the cytotoxicity of ART and semisynthetic analogs on tumor cells, including L-1210, P-388, A-549, HT-29, MCF-7, and KB lines. Beekman et al. (1997, 1998) also investigated the stereochemistry-dependent cytotoxicity of ART and derived analogs.
ART is generally well tolerated at the dose used for malarial patients. The side effects of ART are nausea, vomiting, anorexia, and dizziness. A rare but serious adverse effect is allergic reaction (Taylor and White 2004). The drugs other than ART used in ACTs are believed to contribute to some adverse effects. It was also noted that adverse effects in malarial patients tend to be higher when treated with ART derivatives (Price et al. 1999).
Although the neurotoxicity of ART occurs at high doses in experimental animals, no significant clinic toxicity appears in patients when administered in a therapeutic dosage. A high dose of ART was shown to induce fatal resorption in animals, but no mutagenic and teratogenic roles of ART were found in malaria-infected pregnant women.
2.2.4 The Plausible Mechanisms of ART’s Actions
Currently used ART derivatives are actually predrugs of dihydroartemisinin, a biologically active metabolite of ART, which is effective when parasites reside inside red blood cells. Although there is no consensus regarding the mechanism by which ART kills the malarial parasite, several lines of evidence have indicated that ART may exert antimalarial effects by perturbing the redox homeostasis in the parasite. After infection into a red blood cell, malarial parasites consume hemoglobin in their digestive vacuoles, a process that generates oxidative stress (Ginsburg and Atamna 1994). The activity of malarial cysteine protease in digestive vacuoles can be inhibited by ART (Pandey et al. 1999), and the membranes of digestive vacuoles can be damaged soon after parasites are exposed to ART (del Pilar Crespo et al. 2008). The digestive vacuole is already re-established by a mid-ring stage of the parasite’s blood cycle, a stage sensitive to ART derivatives but not to other antimalarial drugs (Abu Bakar et al. 2010).
A study to investigate the action mode of ART using a yeast model has demonstrated that ART targets mitochondria, in which electron transport chains can activate ART, generate ROS, and depolarize mitochondrial membranes (Li et al. 2005). Subsequent studies have confirmed that ART can attack the mitochondria of parasites but not mammalian cells. Different from atovaquone, ART acting on mitochondria does not inhibit the electron transport during respiration. Therefore, an action specificity of ART derivatives might arise from its own activation (Wang et al. 2010).
It is also thought that when the peroxide lactone of ART comes into contact with a high titer of iron commonly seen in cancerous cells, ART might become unstable and release ROS. Recent pharmacological evidence has demonstrated that dihydroartemisinin targets human metastatic melanoma cells and induces the mitochondrial apoptosis (Cabello et al. 2011).
2.2.5 Conclusions
ART, a sesquiterpene lactone with a unique endoperoxide bridge, is extracted from the medicinal plant species A. annua L. Although ART was originally discovered as a malaria-killing drug, it actually exhibits a wide range of therapeutic potentials, especially in antitumor. However, ART’s applications are often restricted by its availability and cost. Fortunately, ART biosynthetic genes have been cloned and ART precursors are produced in engineered yeast. The re-established or modified ART biosynthetic pathway in either plants or microbes could eventually lead to the large-scale industrial production of ART and derivatives.
2.3 Interactions of Heme with NO and ART
GC is a major receptor for NO binding, by which the enzyme is activated to catalyze the generation of cGMP, thereby playing a pivotal role in cellular function and metabolic regulation (Hobbs and Stasch 2010). On the other hand, NO behaves as a reversible inhibitor of COX to exert a signaling effect (Boveris et al. 2010). Both GC and COX are hemoproteins, whose prosthetic heme moieties are targeted by NO. In theory, all kinds of hemoproteins can interact with NO through the heme-NO interaction albeit resulting in either activation or inhibition.
Based on their functions, hemoproteins can be classified into four subtypes: (1) O2 transport: hemoglobin, myoglobin, neuroglobin, cytoglobin, and leghemoglobin; (2) electron transfer: cytochrome a, cytochrome b, and cytochrome c; (3) catalysis: COX, NOS, CAT, POX, cytochrome P450s, ligninase, and tryptophan oxygenase; (4) sensory: GC, FixL (an O2 sensor), and CooA (a carbon oxide sensor).
2.3.1 Activation of GC by the NO-Heme Interaction
The hemoprotein GC is a heterodimer composed of one α subunit and one heme-binding β subunit. The mammalian GC contains one heme per heterodimer. NO binding to heme results in the activation of a C-terminal catalytic domain and the production of cGMP. NO leads to at least 200-fold increase of GC activity (Wolin et al. 1982), in which NO displaces the axial histidine (His105) by direct competition at the proximal side of heme (Lawson et al. 2000). However, the exact mechanism by which NO-mediated changes in the heme coordination resulting in the activation of GC has not yet been established.
Under oxidative stress conditions, GC can be oxidized and lose its heme. A heme-free enzyme no longer responds to NO, but it can still respond to other activators because they can bind to the empty pocket of heme in a similar manner with NO. In addition, GC contains an allosteric site to which some stimulators can bind. These allosteric agents can potentate the NO-GC signaling, thereby allowing even a suboptimal NO level also maximally activating GC.
2.3.2 Inhibition of COX by the NO-Heme Interaction
The hemoprotein COX is a large transmembrane protein complex found in bacteria and mitochondria in eukaryotes. It is a downstream enzyme complex on the respiratory chain. It receives an electron from each of four cytochrome c, and transfers them to O2. COX accepts four protons from the inner aqueous phase, hence converting one molecule of O2 to two molecules of H2O. Additionally, COX translocates four protons across the mitochondrial membrane to establish a cross-membrane electrochemical potential that allows the production of adenosine triphosphate (ATP) via catalysis by the proton-driven ATP synthase (H+-ATPase).
In mammals, COX composes 14 protein subunits, in which 7 subunits are encoded by the nuclear genome, and 3 subunits are originated from the mitochondrial genome (Balsa et al. 2012). This complex contains one cytochrome a, one cytochrome a 3 , and two copper centers, Cu A and Cu B (Tsukihara et al. 1995). Cytochrome a 3 and Cu B form a binuclear center for O2 reduction. Cytochrome c can be reduced by a preceding component on the respiratory chain, cytochrome bc1 complex (complex III). The ferrous cytochrome c (Fe2+) passes an electron to the Cu A binuclear center and is oxidized to the ferric cytochrome c (Fe3+). An electron from the reduced Cu A binuclear center is first passed to cytochrome a, and then to the cytochrome a 3–Cu B binuclear center.
NO inhibits COX by excluding O2 binding in the range of 80–200 nM (Cleeter et al. 1994; Brown and Cooper 1994) although NO is a multisite inhibitor of mitochondrial complexes IV, III, and I. At a lower O2 tension, NO interacts predominantly with the fully reduced (ferrous/cuprous) center and competes with O2. As the O2 tension is raised, a reaction with the oxidized COX becomes increasingly important. There is no requirement for NO to bind to the singly reduced binuclear center, but it interacts with either the ferrous heme iron or oxidized copper (Mason et al. 2006).
2.3.3 Conjugation of ART with Heme
This was first reported by Meshnick et al. (1991, 1994), who identified the ART-heme adduct by mass spectrometry. The in vitro reaction of ART with heme in the presence of red cell membranes was shown to cause the oxidation of protein thiols (Meshnick et al. 1993). ART was also known to alkylate the heme model molecule at α, ß, and δ carbon atoms (Cazelles et al. 2001) (Fig. 2.2).


Fig. 2.2
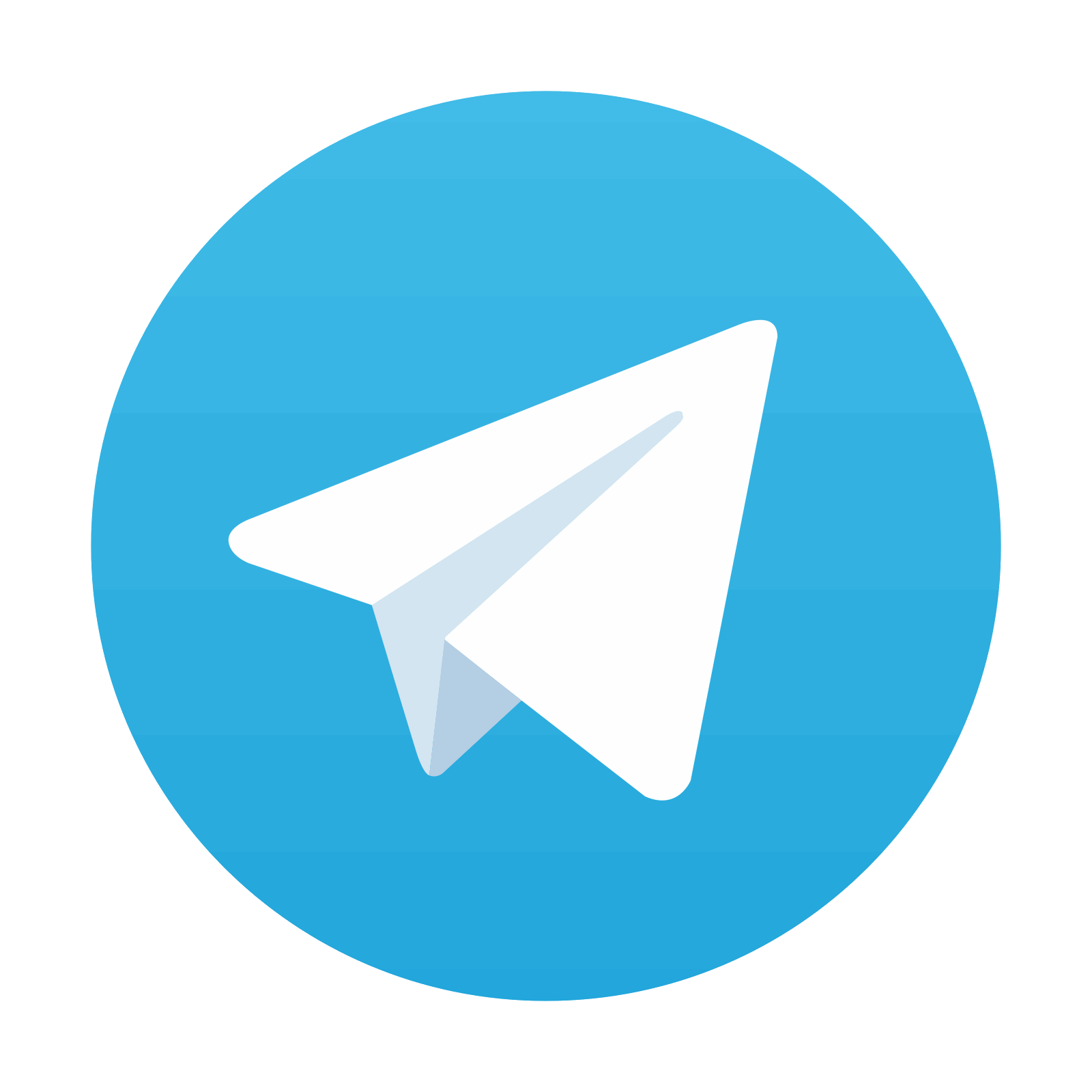
Alkylation of a heme model (dimethyl ester of heme) by a primary carbon-centered radical derived from activated ART. An ART-heme adduct from the β carbon atom is shown, but other adducts are also obtained from α and δ carbon atoms
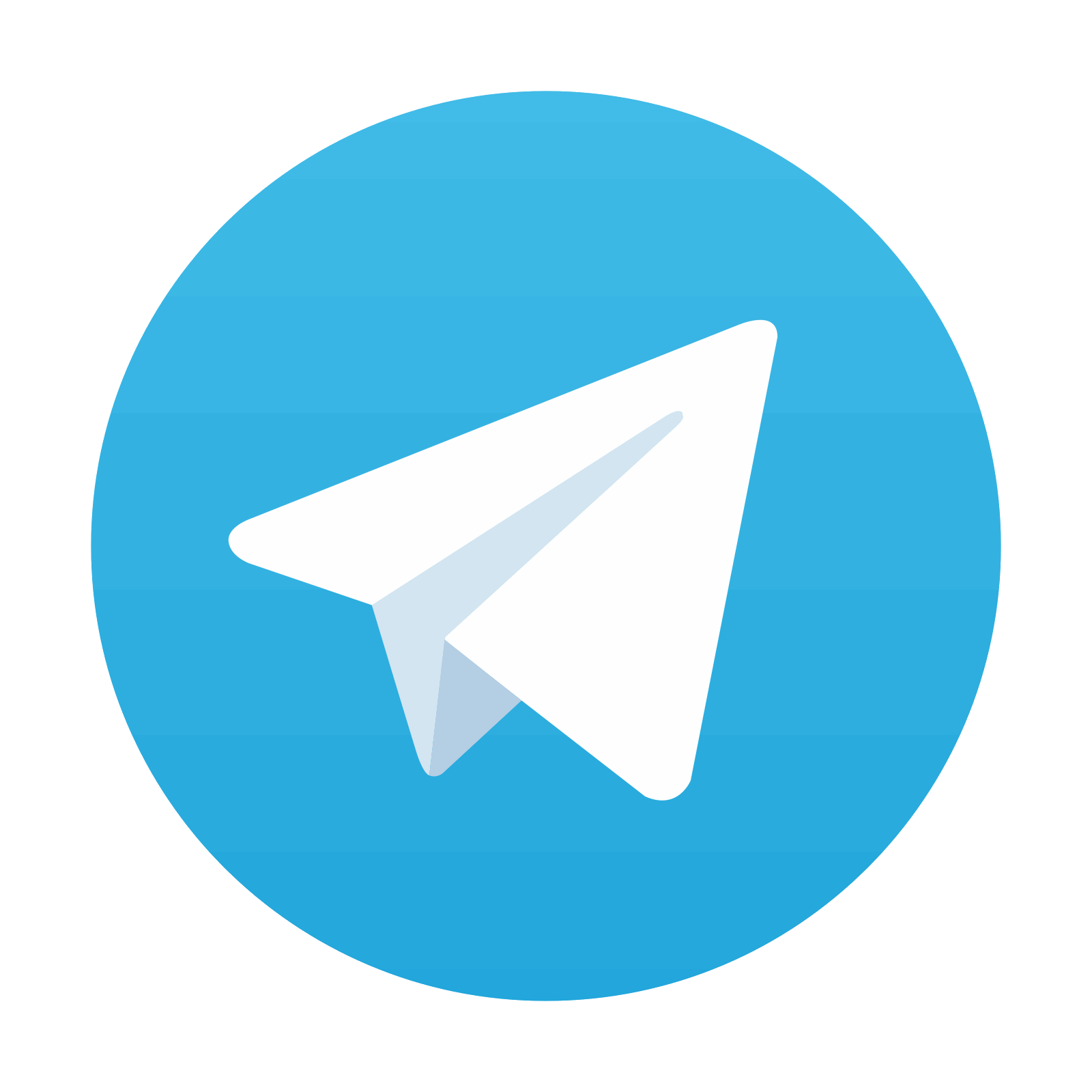
Stay updated, free articles. Join our Telegram channel

Full access? Get Clinical Tree
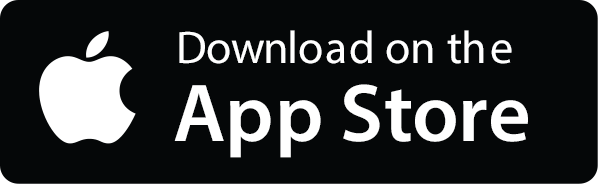
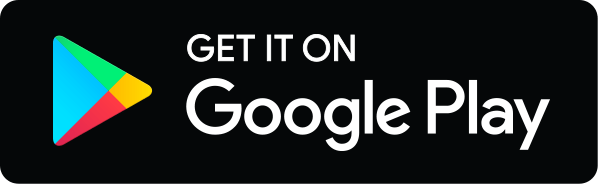
