Chapter 9 Discovering new lead compounds in pharmaceutical research and development
Traditional remedies invariably involve crude plant extracts containing multiple chemical constituents, which vary in potency from highly active (e.g. Digitalis leaf) to very weak (e.g. cinnamon bark). By contrast, orthodox medicine relies heavily on single (or a very small number of) chemically well-characterized active ingredients exhibiting selective activities at, in many cases, well-characterized biological targets. These medicines are generally very potent and many exhibit fairly narrow windows between an effective and a toxic dose. Orthodox medicines are formulated into doses that are carefully standardized for bioavailability.
BIOLOGICAL ASSAYS AND HIGH-THROUGHPUT SCREENING
An example of a biological assay that has the characteristics needed in a good screen is the squalene synthase enzyme assay, which wasdeveloped to look for inhibitors of squalene synthesis, a potential target for the identification of novel cholesterol lowering agents (Tait, 1992). Using either [1-14C] isopentenyl diphosphate as a precursor for squalene or [2-14C] farnesyl diphosphate as a direct substrate of squalene synthase, the production of radiolabelled squalene is determined after adsorption of assay mixtures onto silica gel thin-layer chromatography sheets and selective elution of the diphosphate precursors into a solution of sodium dodecyl sulfate at alkaline pH. The use of [2-14C] farnesyl diphosphate, and of an endogenous oxygen consumption system (ascorbate/ascorbate oxidase) to prevent further metabolism of squalene, allows the method to be applied as a dedicated assay for squalene synthase activity. The assay can be readily operated in microtitre plate format, which allows 96 or 384 samples to be screened per plate. It can be deployed either in a quantitative, low-throughput mode or in a qualitative, high-throughput mode, which has proved to be resistant to interference by compounds other than selective inhibitors.
The endogenous neuropeptide bradykinin (BK) is implicated in the mediation of various types of pain in the mammalian CNS. Antagonism of bradykinin to its receptors is a potential target for the development of new analgesic agents. An assay has been devised to detect compounds that antagonize binding of radiolabelled bradykinin to BKII receptors expressed in Chinese hamster ovary (CHO) cells (Sampson et al., 2000). Compounds under test are added to the wells of microtitre plates to which CHO cells have adhered. After incubation with radiolabelled bradykinin, the excess labelled ligand is removed by washing. The plates are then counted in a scintillation counter so as to assess binding of labelled bradykinin to the receptors expressed on the surfaces of the cells. This particular screen suffers interference from compounds that possess cytotoxicity through a variety of mechanisms. It is therefore essential to run follow-up control assays against other cell types to distinguish false positives.
In the infectious disease arena, it is still common to run high-throughput, whole-cell antifungal or antibacterial assays to detect samples that inhibit growth of the designated strain, e.g. Candida albicans, Staphylococcus aureus. Optical density or colour changes using a redox indicator are the most frequently used assay technologies. Assays in this therapeutic area may be mechanism based. For example, a C. albicans cell-free translation system using polyurethane as a synthetic template, has been established to search for compounds that inhibit fungal protein synthesis (Kinsman et al., 1998).
Screening plant extracts for antitumour activity involves assays against a wide variety of cancer cell lines and mechanism-based in vitro targets, which have been documented extensively (Pezzuto, 1997). Among the most frequently used mechanism-based assays are those assessing activity against the biological targets of existing antitumour drugs, such as topoisomerases I and II, collagenase, tubulin binding and stabilization, endocrine hormone synthesis and androgen and oestrogen receptor binding. Mechanism-based assays often require sophisticated or expensive reagents: an assay for activity of DNA ligase I involves incubating plant extracts with recombinant human DNA ligase I cDNA and its radiolabelled substrate, and measuring uptake 5′-32P labelled phosphomonoesters into alkaline-phosphatase-resistant diesters (Tan et al., 1996).
SELECTING SAMPLES FOR SCREENING
Both strategies are likely to play a role in a pharmaceutical company’s methodology. Diversity screening may yield an unexpected interaction between a compound and a biological target, although the question of what constitutes ‘representative’ chemical diversity in the vast area of potential chemical space remains unanswered. Focused screening requires a large amount of prior information about a target, and this might not always be available. A combination of both approaches may be adopted. Computational methodologies for hit identification are continuously being developed. For example, compound databases enabling three-dimensional chemical structure searching are often used. If there are known ligands for a target, these can be used to construct a pharmacophore, which can then be utilized to search further chemical databases and select molecules with desired features. Chemical clustering can be used to derive sets of the required size. In the case of combinatorial chemistry-derived libraries, targeted sets can be generated with desired chemical properties, by using appropriately selected chemical building blocks. Natural products offer a potentially infinite source of chemical diversity unmatched by synthetic or combinatorially derived compound collections (Strohl, 2000), thus making them a desirable tool for diversity based screening. If a focused strategy is adopted, however, it is necessary to develop different techniques for natural product sample selection in order that the most appropriate samples are accessed for relevant targets.
The United Nations Convention on Biological Diversity (CBD) of 1992 has, to date, 191 parties, including 168 signatories (see its website http://www.cbd.int and the references therein). The key objectives of the Convention are to ensure the conservation of biological diversity, the sustainable use of natural resources and to implement fair and equitable sharing of benefits. Within the framework of the Convention are the concepts of the sovereignty of states over genetic resources and their obligation to facilitate access. The contracting parties are expected to establish measures for benefit sharing in the event of commercial utilization. This involves collaboration between the collector, the source country and the commercial partner. It is now normal practice to draw up a legal agreement to cover these issues and many companies have issued policy statements relating to this area.
As an example, a statement on GlaxoSmithKline’s website (http://www.gsk.com) describes how a pharmaceutical company addresses such issues. The policy recognizes the importance of matters considered at Rio and subsequent meetings of the Congress of the Parties and goes on to state that GlaxoSmithKline will collaborate only with organizations that can demonstrate both the expertise and the authority to supply natural materials. Only relatively small quantities of plant material are collected, from sustainable sources. GlaxoSmithKline supports the CBD’s role in providing a framework for the conservation of biological diversity and the sustainable use of its components and the CBD objective ‘to provide fair and equitable sharing of the benefits arising from the use of genetic resources’. GlaxoSmithKline further supports the approach laid down in the CBD and in the Bonn Guidelines of leaving it to national governments to determine the conditions under which access to genetic resources should be given and for the parties concerned mutually to agree on the benefits to be shared. Agreements will cover such matters as the permitted use of the resources and the nature and timing of any benefits that are to be shared. This approach allows national governments the flexibility to determine what rules will best serve their national interests and allows the stakeholders involved to reach agreement appropriate to each particular case.
STRATEGIES FOR THE SELECTION OF PLANT MATERIAL FOR HIGH-THROUGHPUT SCREENING
Some companies have access to large and diverse natural materials collections. Such collections are likely to include samples acquired to add diversity to the potential collection (and inherent in the desire for taxonomic diversity is the assumption that this will be reflected in chemical diversity of extracts subsequently generated). Most collections will also include samples particularly selected for various reasons, e.g. a microbial producer of a given compound or a plant used ethnomedically for a given condition. These large collections probably still only reflect a fraction of the world’s potential biodiversity. It has been estimated that only around 70 000 fungal species are known, out of an estimated 1 500 000 (see the UN CBD website http://www.cbd.int and the references therein). Further, it has been estimated that only about 1% of microbial biodiversity actually comprises ‘culturable’ organisms (Amman et al., 1995). Thus, a huge number of strains may not be amenable to conventional isolation and cultivation methodologies, and many groups are now working on applying cloning techniques to harness the potential chemical diversity of these organisms.
< div class='tao-gold-member'>
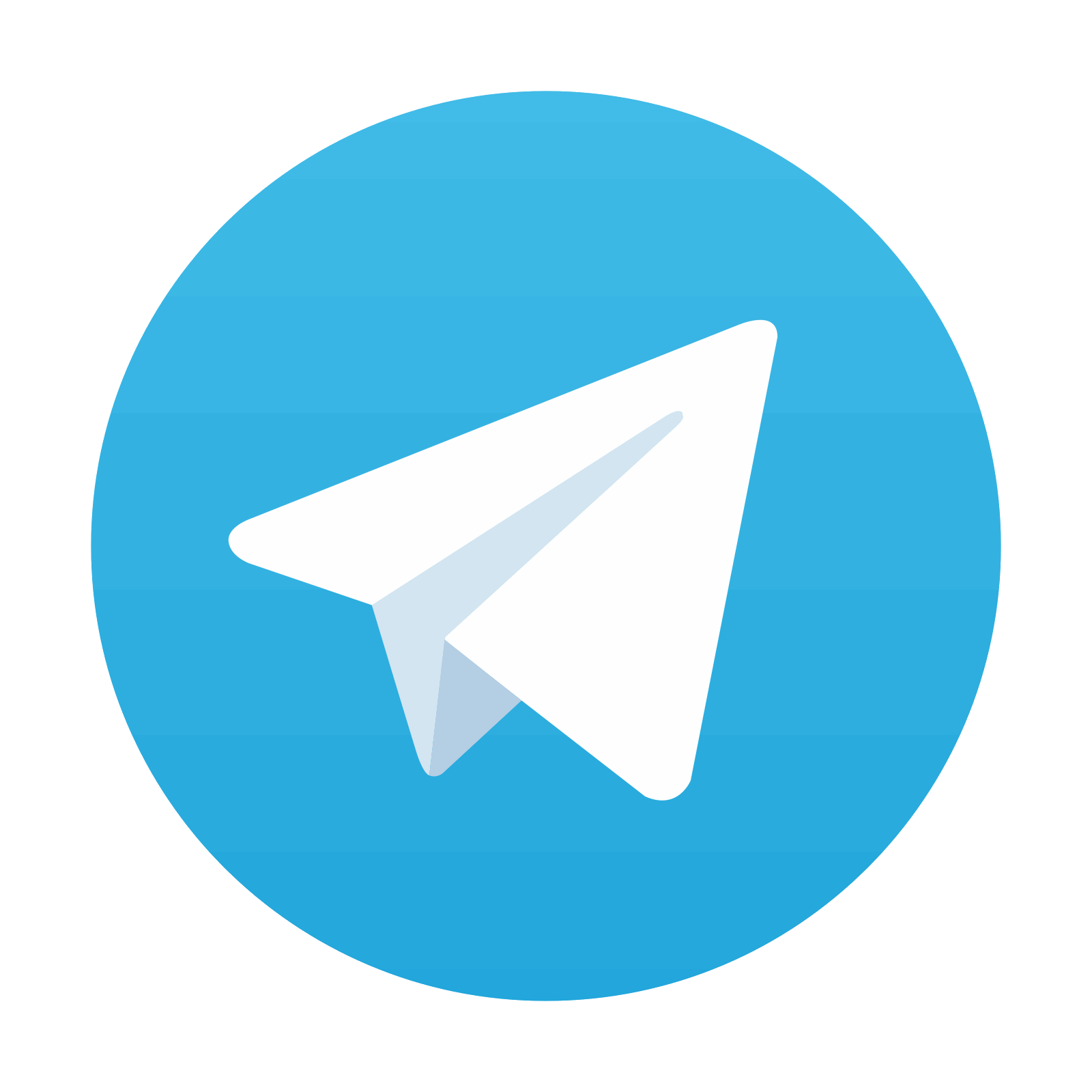
Stay updated, free articles. Join our Telegram channel

Full access? Get Clinical Tree
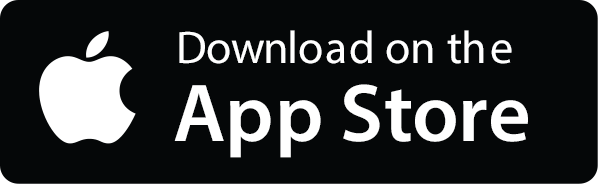
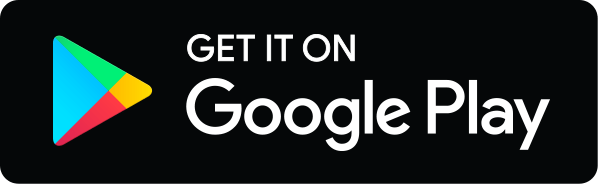