The central nervous system (CNS) comprises neuronal networks of the brain, brainstem and spinal cord (Section 5).

The CNS integrates, processes and responds to sensory messages.

Principles of neurotransmission
Action potentials (APs) passing along axons provide instructions to other neurons or non-neuronal cells (e.g. smooth muscle cells). Instructions are transferred by the release of chemical neurotransmitters from the presynaptic endings of the neuron, which then diffuse through a small physical space, called the synaptic cleft, and stimulate the receiving (postsynaptic) cells via recognition proteins (receptors) (Fig. 4.1). The transmitters may instruct the receiving cells to increase (excite) or reduce (inhibit) their activity.
Fig. 4.1 Principles of neurotransmission at a synapse.
Basic pathways of synthesis, storage, release, action and inactivation of a typical neurotransmitter are shown, as described in the text. At many synapses co-transmission of different neurotransmitters occurs.
Neurotransmitters can be either synthesised within the presynaptic region (e.g. noradrenaline) or transported from the cell body to the synaptic region (e.g. peptides). The neurotransmitter is taken up from the cytosol using specific vesicular transporters within the nerve ending and stored within membrane vesicles. The transmitter in the vesicle may form a complex; for example, noradrenaline forms a complex with adenosine triphosphate (ATP), which reduces the free concentration of noradrenaline within the vesicle.
The release of the neurotransmitter can be ‘fine-tuned’ by axo-axonic connections and by presynaptic receptors (which are discussed below). A generalised scheme for neurotransmission is as follows (Fig. 4.1):
Neurons may release a single transmitter, but often more than one transmitter is released; there are many examples of co-transmission, which are described later in this book.
Presynaptic receptors and modulation of transmitter release
An important characteristic of neurons is the presence of presynaptic receptors (Fig. 4.1 and Table 4.1). Presynaptic receptors may increase or decrease the release of the neurotransmitter and are described as facilitatory and inhibitory, respectively. There are two main sources of ligands for presynaptic receptors:
Table 4.1
The control of transmitter release by presynaptic receptor mechanisms
Neurotransmitter | Presynaptic receptors inhibiting release | Presynaptic receptors facilitating release |
Acetylcholine (ACh) | M2, α2, D2/D3, 5-HT3 | N1, NMDA |
Dopamine | D2/D3, M2 | N1, NMDA |
γ-Aminobutyric acid (GABA) | GABAB | – |
Histamine | H3 | – |
Serotonin (5-hydroxytryptamine, 5-HT) | 5-HT1D, α2 | 5-HT3 |
Noradrenaline | α2., H3, M2, D2, opioid | β2, N1, angiotensin II |

Inhibition of transmitter release is usually achieved by limiting Ca2+ entry through voltage-gated ion channels into the neuron.
The first recognition of a clinically important presynaptic receptor came with the discovery that the antihypertensive agent clonidine lowers blood pressure via stimulation of presynaptic α2-adrenoceptors, with subsequent inhibition of the release of vasoconstricting noradrenaline. Presynaptic receptors (Table 4.1) are increasingly recognised to have important roles in the clinical effects produced by many drugs.
The peripheral autonomic nervous system
The peripheral autonomic nervous system (ANS) is an important site for drug action because:


The peripheral ANS is subdivided into two main branches (Fig. 4.2, Box 4.2):
Box 4.2 Organisation of the ANS





Fig. 4.2 Organisation of the parasympathetic and sympathetic autonomic nervous systems.
Activation of the sympathetic nervous system leads to widespread neuronal release of noradrenaline supplemented by release into the circulation of adrenaline (and noradrenaline) from the adrenal medulla; these act at α- and β-adrenoceptors (see Table 4.2). Stimulation of the parasympathetic nervous system is more localised to particular organs, mediated by acetylcholine acting at muscarinic receptors (see Table 4.3). Some tissues, such as airways, have sparse sympathetic innervation and sympathetic effects are mainly a result of circulating adrenaline. Sympathetic innervation of sweat glands is mediated, unusually, by acetylcholine. The ganglia innervating some organs are not part of the paravertebral chain but grouped together to form the coeliac, superior mesenteric or inferior mesenteric ganglia; the transmitter at all ganglia is acetylcholine acting at nicotinic type 1 receptors. ACh, acetylcholine; NA, noradrenaline; A, adrenaline; GI, gastrointestinal.


Anatomically, in both branches of the ANS, the efferent neurons innervating effector organs are linked to neurons in the CNS via ganglia. The distribution and neuronal interconnections differ between the two branches (Fig. 4.2).


Many organs are innervated by both the parasympathetic and sympathetic nervous systems, which act in concert and may have opposite effects on the organ function. Physiological functions therefore often require inverse coordination of sympathetic and parasympathetic activities; for example, urination is brought about by a decreased sympathetic activity on the sphincter muscle and increased parasympathetic activity on the detrusor muscle (see urinary bladder, Tables 4.2 and 4.3, and Ch. 15).
Table 4.2
Effects of sympathetic nervous system activity via adrenoceptor subtypes in major tissues
aRespond to circulating adrenaline; little noradrenergic innervation.
Table 4.3
Effect of stimulation of parasympathetic nerves (via muscarinic M receptor subtypes) in major tissues
Tissue | Effect | Main receptor type |
Heart rate | Decrease | M2 |
Contractility of atria | Decrease | M2 |
Atrioventricular conduction velocity | Decrease | M2 |
Vascular endothelium | Dilates blood vessel: NO release | M1, M3 |
Bronchial smooth muscle | Constriction | M2, M3 |
Gut motility | Contraction, relaxation | M2, M3 |
Gut sphincter tone | Increased | M3 |
Gut secretions | Increased | M3 |
Bladder detrusor | Contraction | M3 |
Bladder sphincter | Relaxation | M3 |
Penis | Erection | M3 |
Eye pupil circular muscle | Contraction (miosis) | M3 |
Ciliary muscle | Contraction (accommodates for near vision) | M3 |
Pancreatic insulin secretion | Increased | M1, M3 |
Salivary glands | Secretion | M1, M3 |
Emesis | Increased | M3 |
The concept of opposing actions, although imperfect, can be useful in remembering the effects that each part of the nervous system has on tissue function. Tables 4.2 and 4.3 show the effects that stimulation of the sympathetic or parasympathetic nervous systems have on major tissues and the primary receptors that are involved. Under resting conditions, the predominant drive to many organs is from the parasympathetic nervous system.
The sympathetic nervous system and noradrenergic transmission
Noradrenaline and adrenaline are members of a group of amine transmitters called catecholamines (a catechol is a benzene ring with two adjacent hydroxyl groups; Fig. 4.3A). Both the catechol and amino groups are important for receptor binding. The receptors that noradrenaline and adrenaline stimulate are described as adrenoceptors and the effects of noradrenaline and adrenaline at these receptors are described as noradrenergic and adrenergic effects.
Fig. 4.3 The structure of the main physiological catecholamines (A) and their synthesis from amino acid precursors (B).
The catecholamine synthetic pathway is described in the text.
The approved European names of noradrenaline and adrenaline when they are formulated and used therapeutically as medicines are norepinephrine and epinephrine, respectively; however, when their physiological actions are being described, the terms noradrenaline and adrenaline are retained. Most preparations of adrenaline and noradrenaline in Europe are dual-labelled with both terms. In the USA, the terms epinephrine and norepinephrine are used for both therapeutic and physiological descriptions.
Synthesis and storage of catecholamines: noradrenaline, adrenaline and dopamine
Catecholamine neurotransmitters are synthesised from inactive precursors (Fig. 4.3B). The basic carbon skeleton of catecholamines is derived from phenylalanine or tyrosine, which are aromatic amino acids used predominantly in protein synthesis. The sequence of synthesis of adrenaline (via dopamine and noradrenaline) is shown in Figure 4.3B. The oxidation of tyrosine to levodopa by tyrosine hydroxylase, which occurs within the neuron, commits the molecule to become a neurotransmitter. This step is subject to negative feedback by the catecholamines that are subsequently produced, thereby regulating supply. Conversion of levodopa to dopamine is catalysed by a cytosolic enzyme, aromatic L-amino acid decarboxylase (also known as dopa decarboxylase). The amine product, dopamine, is taken up by vesicles via a specific transporter termed the vesicular monoamine transporter 2 (VMAT2). In neurons that use dopamine as their primary transmitter this is the end of the synthetic pathway. Dopamine is a vital neurotransmitter in some parts of the peripheral nervous system and also widely in the CNS (Chs 7, 21 and 24).
The vesicles present in noradrenergic neurons contain dopamine-β-hydroxylase. This enzyme is largely present in the membranes of the vesicles, but on exocytosis some is lost into the synapse, after which it diffuses into the bloodstream and is slowly cleared; its levels in the blood can therefore be used as a marker of peripheral noradrenaline release. In noradrenergic neurons this is the end of the synthetic pathway. Noradrenaline and its precursor dopamine are stored in the vesicles complexed with ATP and proteoglycans.
The chromaffin cells of the adrenal medulla contain an additional cytosolic enzyme (phenylethanolamine-N-methyl transferase), which converts noradrenaline to adrenaline by the addition of a methyl group (Fig. 4.3B). Adrenaline is then transferred into chromaffin cell granules by vesicular monoamine transporter 1 (VMAT1), where it is stored ready for release.
Noradrenaline release
Release in response to a nerve impulse occurs following Ca2+ influx and Ca2+-mediated fusion of the noradrenaline-containing vesicle with the cytoplasmic membrane.
Noradrenaline in the presynaptic neuron may also be released by so-called indirectly acting sympathomimetic amines, which are low-molecular-weight basic compounds; for example, food constituents (such as tyramine), therapeutic drugs (such as ephedrine) and some drugs of abuse (such as amfetamine and metamfetamine). These compounds are taken into the presynaptic cytosol and into the vesicles, from which they displace noradrenaline. Increased noradrenaline release into the synapse is responsible for the biological effects produced by ingestion of compounds like tyramine, ephedrine and amfetamine.
Uptake and metabolism of released noradrenaline
The principal mechanism for the removal of noradrenaline from the synapse is uptake (approximately 70–90%) into the presynaptic neuron via a specific high-affinity carrier called uptake 1 (or the norepinephrine transporter, NET); it also takes up dopamine but not adrenaline (Fig. 4.4). Both noradrenaline and dopamine can then be transferred from the cytosol into the vesicles by VMAT2. Some of the noradrenaline remaining in the synapse is taken up into non-neuronal tissues by a low-affinity carrier called uptake 2, which can also transport adrenaline; some of the remaining noradrenaline and the majority of any adrenaline released into the circulation as a co-transmitter is metabolised. Separate transporters exist for reuptake of serotonin (5-hydroxytryptamine, 5-HT) and dopamine from their respective neurons, termed the serotonin transporter (SERT) and dopamine transporter (DAT), respectively. Therapeutic agents that block NET or SERT increase the amount of neurotransmitter in the synaptic cleft and are used in treating depression (Ch. 22). The uptake of noradrenaline by NET is also blocked by cocaine and amfetamine.
Fig. 4.4 A noradrenergic nerve terminal (varicosity) showing the synthesis and sites of action of noradrenaline and adrenaline.
Dashed lines show the ways that the actions of the catecholamines are curtailed. COMT, catechol-O-methyltransferase; MAO, monoamine oxidase; NA, noradrenaline; NET, norepinephrine transporter (uptake 1).
There are two main enzymes involved in the initial steps in the catabolism of noradrenaline: monoamine oxidase (MAO) and catechol-O-methyltransferase (COMT).
Monoamine oxidase
MAO is present on the surface of the mitochondria of the presynaptic neuron, where it oxidises free cytoplasmic noradrenaline. It is also present in many other sites such as the gastrointestinal epithelium and liver. Oxidative removal of the amino group on noradrenaline via MAO is the major pathway of catabolism of noradrenaline and other aminergic neurotransmitters. Loss of the amino group prevents binding to the postsynaptic receptor, and therefore is an inactivation process. In the periphery, metabolism results in the formation of vanillylmandelic acid, which is the main urinary metabolite. In the CNS, the metabolites are conjugated with sulphate before being excreted in the urine. There are two main MAO isoenzymes, MAO-A and MAO-B, which differ in their organ distribution and substrate affinities (Table 4.4). The use of inhibitors of MAO-A and/or MAO-B isoenzymes in depression and Parkinson’s disease is discussed in Chapters 22 and 24.
Table 4.4
Monoamine oxidase (MAO) and its inhibitors
aBoth isoenzymes are present, but in humans the amount of MAO-B exceeds that of MAO-A.
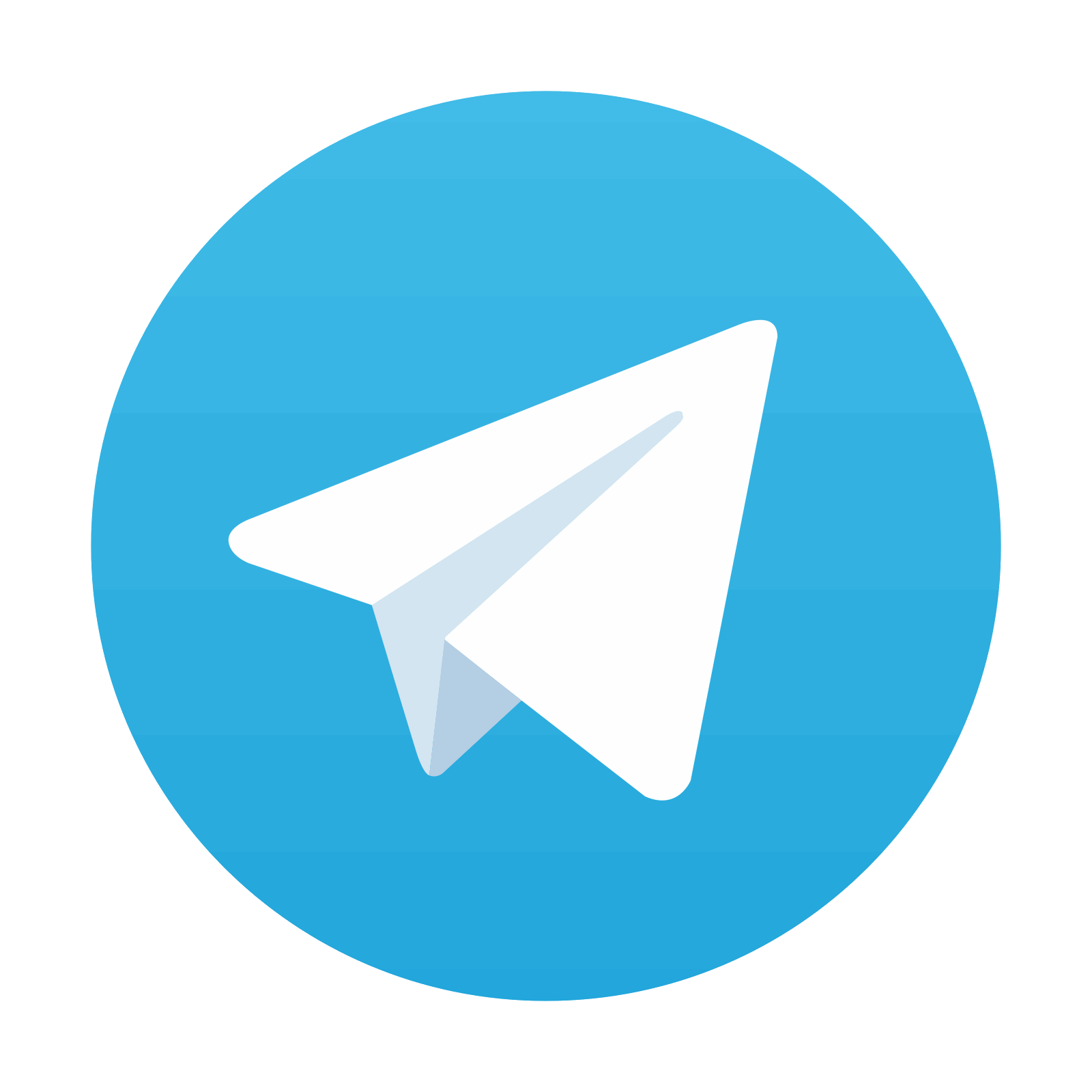
Stay updated, free articles. Join our Telegram channel

Full access? Get Clinical Tree
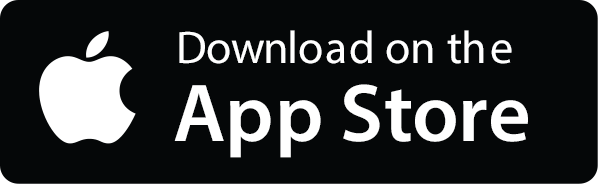
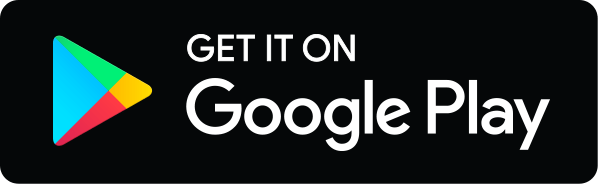