8 William M. Caudle and Gary W. Miller Within this chapter we will provide: The human nervous system is exquisitely complex, composed of billions of neurons and an even greater number of connections among these neurons, all with the fundamental goal of carrying out many basic functions such as the ability to walk, enjoy the smell of a flower, or carry on a conversation with friends. However, the complexity of these finely orchestrated interactions between neurons within different parts of the brain that are imperative for normal neural functioning also makes the human nervous system extremely vulnerable to the effects of different environmental toxicants, especially during stages of neurodevelopment. Neurodevelopment is composed of several different stages, each critical to the proper generation and connection of cells in the nervous system. These critical periods of growth are exquisitely prescribed cellular and molecular sequence of events that must be allowed to occur for the proper placement of anatomical components and connections to be made that will define the subsequent normal function of the CNS. Adding a layer of complexity to this process is the fact that the developing nervous system is tremendously sensitive to exposure to toxic compounds, which can alter multiple processes of neurodevelopment and have deleterious effects on subsequent neurological function and behavior. Therefore, understanding how the CNS develops is essential not only to appreciate the complex anatomy of the mature brain but also to comprehend how traumatic events or exposure to neurotoxic compounds can impact the development and function of the CNS. All neural tissue and cellular components of the CNS (neurons and glia) arise from the neural tube in a process called neural induction. Whether these cells become neurons, astrocytes, or oligodendrocytes is determined by their interaction with specific molecular cues that act on receptors located on the neural precursor cells. Interaction with these receptors modulates the expression of a series of genes involved in differentiating these cells into neurons or glia. Disruption of these initial processes can result in significant nervous system malformations, including spina bifida and anencephaly. Occurring in parallel with the induction of cellular diversity is the formation of the neural tube into the basic anatomical structures of the brain and spinal cord. As seen in neural induction, the activation of particular signaling pathways helps to guide the generation of rudimentary structures that will eventually give rise to recognizable regions of the nervous system. Similarly, altered development of brain regions can have significant effects on neurodevelopment and function. Neurons generated in the CNS must move from the site of their initial genesis to a distant region, where they continue to differentiate into mature neural circuits. This migration of neurons to their particular regions in the nervous system is a tightly orchestrated series of events involving the exposure of the cells to specific chemical cues that help to attract them to the proper spot, while at the same time repelling them from areas they do not belong. Once they have reached their specific regions, neurons extend their processes and form synaptic connections with multiple other neurons in order to establish the complex circuitry necessary to carry out normal day-to-day neural processes. With these various intricacies of the brain it can be appreciated that alteration or damage to even a single aspect of this system can have significant repercussions to the normal function of the brain and the behaviors it is charged with overseeing. Thus, in order to fully comprehend and appraise the effects of particular toxins and toxicants on the nervous system, a comprehensive understanding of the nervous system is essential. The nervous system is separated into two anatomically distinct parts: the CNS and the peripheral nervous system (PNS). Although their structure and location may be different, these two aspects of the nervous system are not functionally delineated. Rather, their functions are highly dependent upon the other and cooperate in an integrated fashion to elicit neurobehavioral responses. With this in mind, we will focus this section on describing the general structures and physiology of the major parts of each system and provide a brief appraisal of the interaction between the two when possible. The major components of the CNS are the brain and spinal cord. The spinal cord sends, receives, and then processes sensory information from skin, muscles, and joints to produce movements in response to these inputs. These functions are integrated and facilitated by the brain via the mass of nerve fibers that course throughout the entire length of the spinal cord providing a conduit through which the brain can elicit a coordinated response to the peripheral information it is constantly receiving. Like the CNS, the brain can be subdivided into several anatomically distinct, yet functionally integrated regions (Figure 8.1). Moving up from the spinal cord, the first part that connects the spinal cord with the brain is the brainstem. The brainstem is involved in general aspects of arousal as well as in acting as a conduit through which nerve fibers between the spinal cord and the rest of the brain travel and communicate. The brainstem can further be divided into specialized regions called the medulla, pons, and midbrain. The medulla and pons participate in the regulation of blood pressure and respiration, while the midbrain plays an important role in controlling the movement of the eyes and body. The brainstem is also important as it is the point from which most of the cranial nerves, which are part of the PNS, emanate. The functions of the cranial nerves will be discussed in greater detail later. Given their role in regulating important aspects of respiration, damage to the medulla and pons could have dire consequences. Indeed, benzodiazepines, such as valium, are CNS depressants, acting on the respiratory centers of the brainstem and suppressing their function, which can result in death. Figure 8.1 Superficial structures of the human brain. Picture provided courtesy of the University of Washington School of Medicine, Department of Pathology. Continuing further up from the brainstem is the cerebellum. The cerebellum is connected to the brainstem through an intricate series of nerve fibers that arise from the spinal cord and brainstem, as well as other parts of the brain. It receives and integrates information from each of these regions and uses it to regulate vital aspects of posture and balance as we move or stand. Damage to this region can result in a lack of coordination. Thus, it makes sense that many of the uncoordinated movements that are seen following ingestion of ethanol can be attributed to its effect on the cerebellum. The diencephalon consists of two major regions: the thalamus and the hypothalamus. The thalamus acts as a complex relay station for sensory information that travels from the spinal cord and cranial nerves as well as other parts of the brain and is routed to the appropriate regions of the brain that will eventually integrate this information and facilitate a motor response. Just below the thalamus is the hypothalamus. The hypothalamus regulates eating, drinking, sleeping, and sexual behavior. In addition, it also works in conjunction with the autonomic nervous system (discussed later) to modulate its function as well as control endocrine processes mediated through the pituitary gland. The cerebral hemispheres are the most structurally complex and developed regions of the CNS. The cerebral hemispheres consist of four main components: the cerebral cortex, the hippocampal formation, the basal ganglia, and the amygdala. Together, these structures mediate the most sophisticated human behaviors, such as perception, cognition, movement, and emotion. Again, it should be noted that although their anatomical location and structure may be different, each of these regions is intimately integrated, transmitting and receiving information from each other in order to obtain a comprehensive neural image of a stimuli and to fine-tune the response. The most prominent and visually accessible structure of the cerebral hemispheres is the cerebral cortex. The cerebral cortex is most easily identified by its convoluted surface formed by ridges (gyri) and grooves (sulci). This appearance is a result of an evolutionary adaptation that allowed for a greater surface area of the brain to fit within a confined space of the skull. The cerebral cortex can be further divided into four distinct yet integrated lobes. The frontal lobe is essential for motor behavior, cognition, and emotion. In addition, the frontal lobe houses our faculties that allow us to make decisions about what are right or wrong, safe or dangerous. The parietal lobe is concerned with our perception of bodily senses, such as touch and pain, as well as with providing a sense of where our limbs exist in space. In this regard, the parietal lobe works closely with other brain regions involved with motor movement to assist us when we reach for a cup of coffee or hammer a nail. The temporal lobe is involved in the perception of language and sound, in addition to functioning in memory. Finally, the occipital lobe controls the perception and integration of all visual stimuli. As with all other lobes, the occipital lobe is in close reciprocal communication with other regions of the brain in order to refine the sensory information it is receiving as well as the information it transmits to other regions. Located beneath the lobes of the cerebral cortex are three major components (Figure 8.2). The hippocampus is the part of the brain primarily responsible for learning and memory. Damage to this region can not only affect the ability to recall memories from the past (retrograde amnesia) but also impair the ability to form new memories or learn new tasks (anterograde amnesia). For instance, some algae blooms produce a potent neurotoxicant that targets the hippocampus and destroys vital areas responsible for memory formation and learning. The amygdala mediates our emotions while coordinating our physiological response to stressful or threatening situations. It should be no surprise that the amygdala and the frontal cortex are heavily connected. The amygdala and hippocampus are also tightly integrated in terms of remembering situations or specific events that may have elicited a stressful or threatening response in the past. Damage to the amygdala can result in the inability to appropriately assess a situation as being dangerous or threatening. Finally, the basal ganglia are one of many structures that are involved with modulating movement. Its role is to integrate information with the cerebral cortex and use this to refine or fine-tune our movement. The basal ganglia is the primary structure that is damaged in Parkinson’s disease (PD) and is caused by loss of neurons in this region and a reduction in the neurotransmitter dopamine. Interestingly, several neurotoxic compounds, including 1-methyl-4-phenyl-1,2,3,6-tetrahydropyridine (MPTP), carbon monoxide, and carbon disulfide, target the basal ganglia and produce deficits in movement. Further effects of these compounds will be discussed later. Figure 8.2 Coronal aspect of the human brain. Picture provided courtesy of the University of Washington School of Medicine, Department of Pathology. The PNS is composed of the nerves that arise from the brain (cranial nerves) and spinal cord (spinal nerves) and are ultimately integrated and modulated by the CNS. Both the cranial and spinal nerves of the PNS can be functionally divided into the somatic and autonomic nervous systems. The somatic division carries motor information to the skeletal muscles and joints as well as transmits sensory information from sensory receptors of the body to the CNS. These functions are usually considered as “voluntary,” which is in contrast to the “involuntary” functions of the autonomic division. The somatic nerves are exquisitely sensitive to damage by several classes of neurotoxic compounds, including gamma-diketones, which can specifically damage these nerves and disrupt the ability to sense touch and pain and conduct motor movements. The autonomic division contains nerve fibers that are responsible for the motor and sensory aspects of internal organs, such as controlling cardiovascular, respiratory, and gastrointestinal functions. The autonomic nervous system can be further divided into the sympathetic and parasympathetic divisions. These divisions maintain an opposing yet complementary function with each other. While the sympathetic division can be considered to be involved in the “fight or flight” physiological response, which serves to enhance blood flow to the heart and muscles, increase respiration, and shunt blood from nonessential biological processes, the parasympathetic division promotes a “rest and digest” response, which lowers blood pressure and breathing, and increases blood flow to organs such as the gastrointestinal tract in order to facilitate these functions. The two major cellular components of the nervous system are neurons and glia. These cells work in conjunction with each other to maintain the overall structure and function of the nervous system. We will consider the structure and function of neurons first. Although several different types of neurons exist, they are all composed of the same four structures, which define their morphology and function as neurons. Dendrites form chemical connections with and receive information from other neurons. This information is passed on via the interaction of chemical neurotransmitters with their specific receptors, which are located on the dendrites. The cell body contains the nucleus, ribosomes, and other basic cellular structures necessary for viability of the neuron. Because other parts of the neurons do not produce many of these necessary proteins and other molecules, they must be transported from the cell body. The most reliable method is via axonal transport, which utilizes specialized cytoskeletal proteins used to provide stabilization of the neuron to move this cargo wherever it is needed. In addition to facilitating axonal transport, the major function of the axon is to transmit information in the form of electrical impulses called action potentials, which are the fundamental signals that carry information from one place to another in the nervous system. These electrical signals are initiated by fluxes in concentrations of potassium and sodium that lie on either side of the neuronal membrane creating an electrical potential, called the resting membrane potential. In general, potassium exists in a greater concentration inside the neuron while sodium has a larger concentration outside the neuron. The action potential is produced through the sequential opening and closing of specific sodium and potassium ion channels that are integrated in the axonal membrane, which allows for a change in ion concentration as well as a change in the electrical potential across the membrane (Figure 8.3). The opening and closing of these channels occurs all the way down the axon as the electrical signal is propagated. There are numerous toxic substances found in nature, such as the poison from a jellyfish sting or poison dart frog secretions that specifically target these ion channels and disrupt their functions. Figure 8.3 Diagram of components involved in the neuronal action potential. The speed at which these signals travel is facilitated by the wrapping of myelin around the axon at defined intervals along the entire axon. In the CNS this is done by oligodendrocytes, while Schwann cells are responsible for myelin production in the PNS. Like an electrical wire, the myelin serves as insulation for the axon, which allows the electrical signal to travel down the axon more efficiently. In between each myelinated segment of the axon is a space called the node of Ranvier. Within these intervals where myelin is not present are sodium channels that serve to regenerate the action potential and help in the propagation of the signal down the length of the axon.
NEUROTOXICITY: TOXIC EFFECTS ON THE NERVOUS SYSTEM
8.1 Nervous System
Central Nervous System
Peripheral Nervous System
Neurons
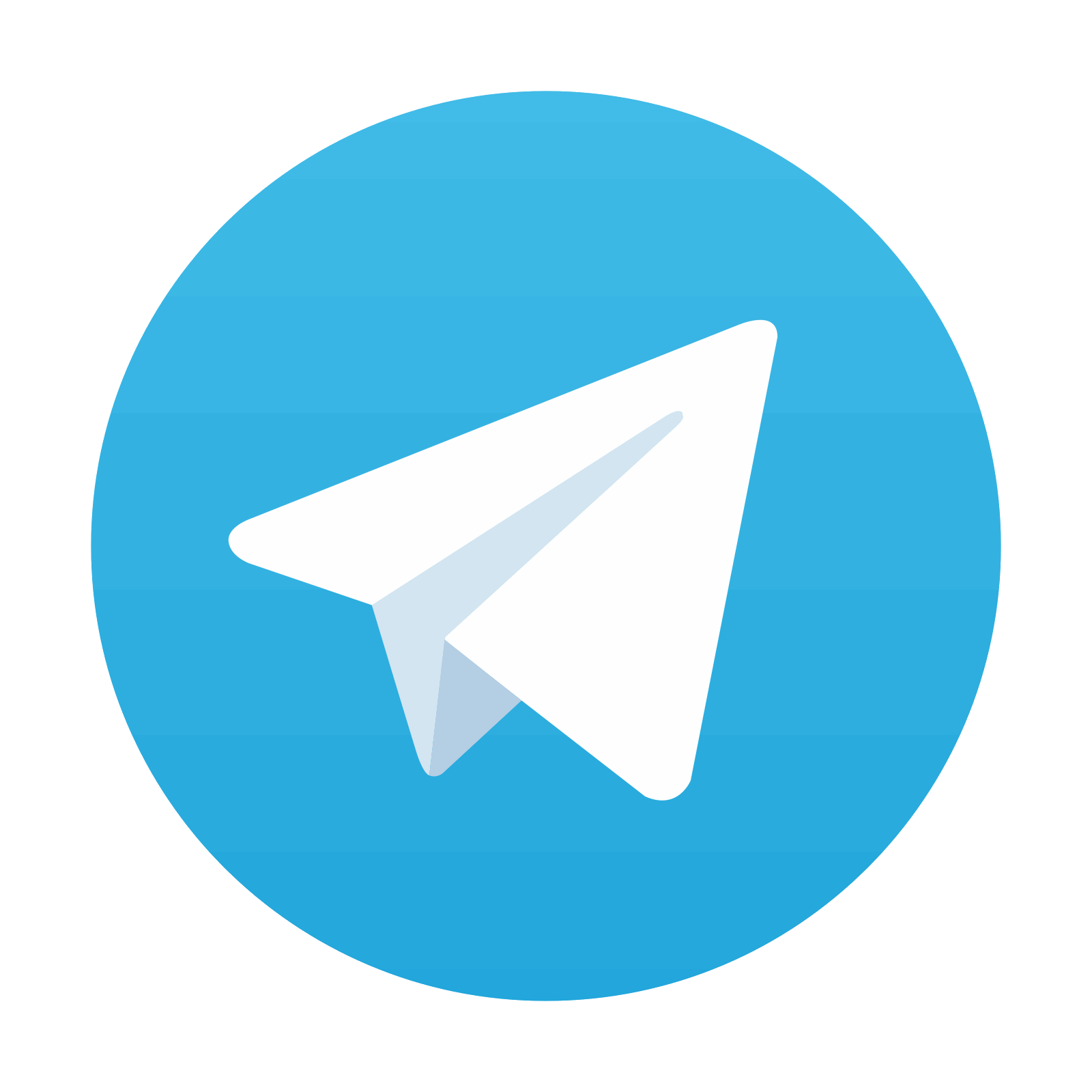
Stay updated, free articles. Join our Telegram channel

Full access? Get Clinical Tree
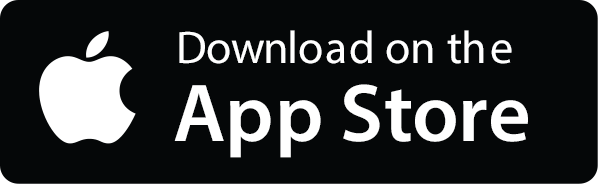
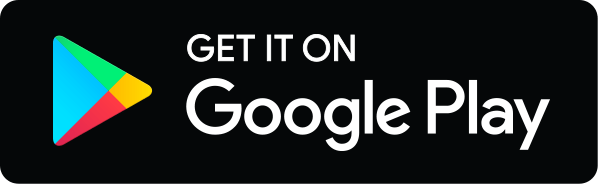