Characteristic
NRP1
NRP2
Chromosome location
10p11.22 (human)
2q33.3 (human)
Molecular weight
130–140 kDa
130–140 kDa
Receptor isoforms
NRP1, NRP1Δ16
NRP2a17, NRP2a22
NRP2b0, NRP2b5
Soluble forms
s11NRP1, s12NRP1, sIIINRP1, sIVNRP1, s10Nrp1
s9NRP2
Cytoplasmic domain
Ends in SEA, binds PDZ-containing proteins
NRP2b does not end in SEA
Vascular localization
Arteries
Veins and lymphatic vessels
Angiogenic ligands
VEGFA, VEGFB, VEGFE, PGF, HGF
VEGFA, VEGFC, VEGFD
Inhibitory ligands
SEMA3A, 3B, 3C
SEMA3B, 3F, 3G
Knockout mouse
Lethal at E12.5-E13.5
Viable
Knockout mouse vasculature phenotype
Severely impaired blood vasculature
Fewer lymphatic capillaries, no edema
NRP1 and NRP2 are 130-kilodalton (kD) type I transmembrane glycoproteins, meaning they span the membrane in one pass and the N-terminal region of the protein is extracellular, and the C-terminal region is cytoplasmic. Approximately 80–90% of the protein is found outside the cell, leaving a small cytoplasmic region. The extracellular domains of both NRP1 and NRP2 are similar, consisting of two CUB motifs named domain a1 and a2, two domains homologous to coagulation factor V and VIII named b1 and b2, and a MAM (meprin, A5, μ) domain designated as domain c. The a and b domains are involved in ligand binding, while the c domain and transmembrane domains contain multiple conserved cysteine residues thought to be involved in adhesion or dimerization [11, 12]. The a domain is involved specifically with binding to the amino-terminal sema domain in class 3 semaphorin (SEMA3) proteins. The crystal structure of NRPs shows that the a2b1b2 domain forms a tightly packed unit, while the a1 domain is only loosely associated [13]. Intact SEMA3 ligands also make contacts with the b domains of NRP via their C-terminal basic region [14, 15]. The angiogenic vascular endothelial growth factor (VEGF) family including VEGF-A, VEGF-C, and placenta growth factor (PGF) bind to the b1b2 domain of NRP1 and NRP2 [14, 16, 17]. The domains that other NRP ligands such as hepatocyte growth factor (HGF) or transforming growth factor beta (TGFβ) interact with have not yet been identified [18, 19].
There are two transmembrane receptor NRP1 isoforms, the full-length NRP1 (923 aa) that contains all 17 exons and an alternative isoform that lacks exon 16, NRP1(ΔE16) (907 aa) [8, 20]. The NRP1(ΔE16) receptor lacks 17 amino acids (replaced by an arginine) from an extracellular region near the transmembrane domain, but this receptor still binds VEGF ligand and presumably signals similarly to the full-length NRP1 [20]. Four NRP2 transmembrane isoforms exist: NRP2a(17) (926 aa), Nrp2a(22) (931 aa, insert of GENFK), NRP2b(0) (901 aa), and NRP2b(5) (906 aa, insert of GENFK) [8, 10, 21]. NRP2a and NRP2b isoforms are generated by alternative splicing and have identical extracellular domains, meaning that they can bind the same ligands. However, the cytoplasmic domain of NRP2a more closely resembles that of NRP1 in size and sequence and both end in a 3-amino acid sequence: serine-glutamic acid-alanine (SEA). This C-terminal SEA is reported to bind to proteins containing a PDZ (PSD95, Dlg1, Zo1) domain, which is a common structural motif in scaffold proteins that anchor membrane receptors to cytoskeletal components. NRP1 (and presumably NRP2a) binds to synectin, also called NIP (neuropilin-interacting protein) or GIPC (GAIP-interacting protein, C-terminus) [22–24]. The NRP2b isoform has a shorter cytoplasmic domain that is only 11% homologous to NRP2a [21]. NRP2b lacks the SEA cytoplasmic tail and therefore does not possess the capacity to bind synectin.
Soluble NRP (sNRP) proteins are truncated NRP isoforms with molecular weights ranging from 60 to 90 kD [10]. sNRP1 was first detected from a 2.2-kilobase (kb) mRNA species found after Northern blotting mRNA from human PC3 prostate carcinoma cells with radioactive probes to the a or b domain of NRP1 [25]. Subsequent cloning of this band resulted in the identification of a novel 644-amino acid (aa) isoform resulting from a read-through into intron 12 and a premature stop codon. As more isoforms began to be identified, this first isoform was later named s12NRP1, which contained the entire a and b domains of NRP1 and a unique 28-base-pair (bp) sequence at its carboxy terminus, yet lacked the c domain, transmembrane domain, and cytoplasmic domain [25]. Three other soluble isoforms of human NRP1 were later identified: s11NRP1 reads into intron 11 and is 704 aa, sIIINRP1 lacks exon 10–11 but includes intron 12 and consists of 551 aa, and sIVNRP1 skips exon 11 but reads into intron 12 and consists of 609 aa [8, 26]. An additional sNrp1 isoform, s10Nrp1, was cloned in the mouse and is 588 aa [27]. So far, the only human sNRP2 identified is s9NRP2, a 555 aa isoform containing the a domains, b1 domain, and part of the b2 domain [8]. Full-length NRP1 and sNRP1 share a common promoter, yet the expression and regulation of each isoform can be cell-type specific. For instance, in the liver, hepatocytes strongly express sNrp1, while sinusoidal endothelial cells express Nrp1 [25, 27, 28].
The sNRP1 isoform can bind and sequester VEGF-A [25, 29] or HGF [27], and sNRP2 can bind and sequester VEGF-A [14], VEGF-C [30], or semaphorin 3F (SEMA3F) [14]. Although sNRPs bind ligands, they do not form co-receptor complexes with other transmembrane receptors and do not transmit signals into the cytoplasm [25, 31]. sNRP1 was found to inhibit invasion of lung cancer cells in vitro [32], and a mutated sNRP2 which preferably binds VEGFA over SEMA3F [14] was shown to inhibit human melanoma growth in nude mice [31]. Therefore, sNRPs behave functionally in the opposite manner to full-length NRP receptors and can act in a stimulatory or inhibitory manner depending on the ligand interaction that they are antagonizing. sNRPs contain only a few novel amino acids that are unique and not found in NRP, and therefore no specific antibodies to any of the sNRPs are commercially available. Specific detection of sNRP requires either in situ hybridization with intron-specific probes in tissue sections or Western blotting of tissue lysates using N-terminal NRP-specific antibodies [25, 27]. The results of immunohistochemistry, which is routinely performed to detect NRP levels in tumor biopsies, must be scrutinized carefully since antibodies to the N terminus of NRPs will detect both membranous and soluble forms. C-terminal-specific antibodies are preferred to detect the full-length receptors with the distinction between NRP2a and NRP2b.
Neuropilin Ligands
Neuropilins were discovered in the neuronal system, and the first ligands identified for these receptors were the class 3 semaphorin (SEMA3) family of axonal guidance proteins [21, 33, 34]. Later NRPs were shown to be a unique class of receptors for the VEGF family of angiogenic molecules [35]. This link between the neuronal and vascular systems opened up an entirely new field comparing the molecular similarities in these two distinct yet similarly patterned systems [36]. Today it is now appreciated that NRPs can bind and mediate signals through many ligands including platelet-derived growth factor (PDGF), HGF, and TGFβ (reviewed by [37–39]). We will summarize each ligand family and its relation to NRP function below with particular interest in those processes involved in cancer progression such as angiogenesis, migration, invasion, and metastasis.
VEGF Family
VEGF is fundamental for the development and survival of vascular supply, and therefore VEGF signaling is pivotal for physiological functions and homeostasis by maintaining a functional network of blood vessels [40]. VEGF also plays an essential role in inducing vascular permeability, which is important for angiogenesis associated with tumors and wound healing [41]. NRPs serve as receptors for growth factors in the VEGF family with differential binding affinities for each ligand. The VEGF binding sites reside in the b1 and b2 domains on the extracellular component of the NRP receptors [13, 14, 16, 42, 43]. Therefore, both membranous and secreted NRPs can bind VEGF, as they all contain intact b1/b2 domains.
The human VEGF-A gene encodes multiple isoforms that arise due to alternative splicing. The VEGF-A isoforms differ in their inclusion of exons 6 and 7, which are the domains responsible for heparin binding [44]. VEGF-A121 is a slightly acidic form that cannot bind heparin, while VEGF-A165, VEGF-A189, and VEGF-A206 all contain exon 7 and bind heparin. NRPs were initially described as isoform-specific receptors for only heparin-binding isoforms of VEGF-A, in particular, VEGF-A165 [35, 45]. Later, crystal structures of NRPs indicated a VEGF-A exon 8a binding site [13, 46, 47]. Furthermore, a newly identified VEGF-A variant called VEGF-A165b, which lacks exon 8a but includes exons 7 and 8b, did not bind to NRP1 [48]. Taken together, these data suggest that the b1 domain in NRP1 or NRP2 can bind VEGF-A via regions encoded by exon 7 and 8 [49]. The VEGF-A165 ligand can bind to both NRP1 or NRP2, but recent evidence shows that binding is 50 times stronger to NRP1 than NRP2 [49]—this may explain why the initial expression cloning of the putative VEGF-A165 receptor (now known as NRP) yielded six clones of NRP1 and only one clone for NRP2 [35].
VEGF-A isoforms bind to the canonical tyrosine kinase VEGF receptors, VEGFR1 or VEGFR2, via regions encoded by exon 4 (reviewed by [50]). Thereby, the VEGF-A165 protein can “bridge” between NRP receptors (via exon 7–8) and VEGFRs (via exon 4) at the same time forming a complex that enhances the output of the receptor tyrosine kinase (RTK) [35, 45]. As described above, the NRP cytoplasmic domain is small (40 aa) and does not contain kinase activity; therefore, NRPs are often referred to as “co-receptors” since the business end of the signaling is performed through VEGF RTKs. Both VEGFR1 and VEGFR2 have been shown to complex with NRP1 and NRP2 in the presence of VEGF-A [45, 51].
Nearly all members of the greater VEGF family, which share NH2-terminal cystine knot domains, have been shown to interact with either NRP1 or NRP2. Specifically, two family members that most closely resemble VEGF-A in sequence and structure are VEGF-B and PGF. VEGF-B167 and PGF2 both contain basic COOH-terminal domains and sequences homologous to exon 7 of VEGF-A, and both have been reported to bind NRP1 [16, 52, 53] and signal through VEGFR1 [54].
Alternately, the lymphangiogenic members of the VEGF family, namely, VEGF-C and VEGF-D, primarily bind to NRP2 via its b1b2 domain (although they can also bind to NRP1) and transmit their signal through the VEGFR3 RTK [17, 55, 56]. The VEGF-C proprotein (uncleaved version) binds better to NRP2 than does the amino-cleaved version of VEGF-C. VEGF-C can bind to NRP2 in the absence of heparin, but only interacts with NRP1 in the presence of heparin [17]. VEGF-D protein requires heparin to bind to either NRP [17]. The presence of NRP2 enhances the phosphorylation of VEGFR2 in the presence of VEGF-A or VEGF-C [55].
Other ligands that are related to the VEGF superfamily include PDGF and TGFβ. Both of these proteins are capable of binding heparin and have cystine knot and beta-strand topology [57]. Although the precise binding sites on these ligands and NRP domains have not been mapped, it is clear that NRPs can form co-receptor complexes with the PDGF receptors (PDGFR) and TGFβ receptors (TGFβR). Knockdown of NRP1 in mesenchymal stem cells or vascular smooth muscle cells attenuated PDGFRα phosphorylation by PDGF-AA and/or reduced PDGFRβ activation by PDGF-BB [58, 59]. Additionally, NRP1 O-linked glycosylation at Ser612 regulated PDGF-induced smooth muscle cell migration [59].
NRP1 and NRP2 are able to bind to both the active and latent forms of TGFβ1 [19, 60]. TGFβ1 can compete with VEGF-A binding for NRP, suggesting that TGFβ also binds to the b1b2 domain of NRP. Moreover, Nrp1 expression can activate latent TGFβ1 [19]. NRP1/2 were shown to form co-receptor complexes with TGFβRI and RII. TGFβ is also a major inducer of the epithelial-to-mesenchymal (EMT) phenotype in cancer cells, and TGFβ has been shown to induce NRP2 expression in hepatocellular carcinoma cells [61].
HGF
NRP1 can bind to another heparan sulfate binding protein called hepatocyte growth factor (HGF) or scatter factor [62]. Active HGF is a heterodimeric, disulfide-linked protein composed of an alpha chain and a smaller beta chain [63]. HGF is secreted as a single-chain inactive protein and then cleaved by serine proteases to the active form. The N-terminal hairpin loop region of the HGF protein structure is strikingly similar to the heparin-binding C-terminal region of VEGF-A [64]. HGF binds and signals through the c-Met receptor tyrosine kinase. Classically, HGF stimulates mitosis in hepatocytes, but HGF also has potent angiogenic and lymphangiogenic properties in endothelial cells [65–67].
NRP1 and NRP2 both bind HGF, and HGF can compete VEGF-A binding to NRPs suggesting that HGF may bind to the b1b2 domain on NRPs [18]. NRP1 has been shown to act as a co-receptor for HGF with c-Met, and HGF likely binds to these two receptors independently or as a bridge via different domains since knockdown of NRP1 or NRP2 could not inhibit all signaling through c-Met [18]. sNRP1 also binds and sequesters HGF [27]. Since NRP1 is highly expressed in sinusoidal endothelial cells in the liver and the liver hepatocytes are a prime source of HGF, one may speculate that NRP1 in the liver is primarily an HGF receptor rather than a VEGF receptor. After hepatectomy, sNrp1 levels plummet in the liver and only increase after regeneration suggesting that this endogenous soluble receptor may regulate the bioavailability of ligands during the healing process [27].
SEMA3 Family
The semaphorin (SEMA) family is a large group of proteins (more than 20 vertebrate members) which share a common structure at the amino terminus called the “sema” domain that is folded into a β-propeller structure with seven blades homologous to alpha integrins (reviewed by [68]). The SEMA family is divided into eight classes (numbered 1–7, and a viral group) containing proteins that span the plasma membrane with the exception of the vertebrate class 3 SEMA (SEMA3) proteins that are secreted. There are seven different SEMA3 secreted proteins labeled A to G that share a cysteine-rich PSI (plexin-semaphorin-integrin) domain, an immunoglobulin domain, and a C-terminal basic-charged domain (in addition to the sema domain) (reviewed by [69]). SEMA3s were originally named “collapsins” for their ability to collapse axonal growth cones [70, 71]. Subsequently, NRPs were found to be high-affinity receptors for these secreted SEMA3 mediators of neuronal guidance [21, 33, 34]. All members of the SEMA3 subfamily bind to NRPs except SEMA3E, which binds directly to Plexin D1 [72, 73]. Although NRP1 and NRP2 extracellular domains are quite homologous, there is specificity within the SEMA3 family for binding to either receptor. SEMA3A binds specifically to NRP1 [33, 34], and full-length SEMA3G binds specifically to NRP2 [74], whereas SEMA3B, C, D, and F can bind to either NRP (reviewed by [75]). In the case of SEMA3F, its affinity to NRP2 is tenfold higher than to NRP1 [21], and SEMA3F appears to only function through its interaction with NRP2 [76], suggesting that NRP1 may be a lower-affinity decoy for SEMA3F.
The functions of SEMA3 proteins are regulated by proprotein convertases (PPC)—similar to that of other proproteins like MMP, VEGF-C, and TGFβ. All SEMA3 proteins contain conserved PPC recognition sites (KRRXRR) between the sema and Ig domains and in the basic C-terminal domain. The SEMA3 proteins are secreted as 100 kD proproteins that are initially cleaved at their C-terminus to 95 kD proteins and then subsequently cleaved upstream to create two protein fragments of ~65 kD and ~30 kD (Table 14.2). The second cleavage is important for function because some SEMA3 proteins bind to NRPs as dimers, making disulfide bridges at conserved cysteines, while others function as monomers. As an example, the affinity of the SEMA3A dimer (95 kD) is 10,000 times greater for NRP1 than the cleaved monomer (65 kD) [77], and dimerization is necessary for collapsing activity of SEMA3A [78].
Table 14.2
Structure to function relationship in SEMA3 proteins
SEMA3 | Full-length protein | Protein cleavage products | |
---|---|---|---|
p100/p95 | p65 | p30 | |
SEMA3A | p95 active dimer, binds Nrp1, repulsion/collapse | p65, monomer, inactive, does not bind Nrp1 | p30, inactive, does not bind Nrp1 |
SEMA3B | p95a active, binds Nrp1 and Nrp2, EC repulsion | ||
SEMA3C | p95a active, binds Nrp2, LEC repulsion | p65 active, Nrp dependency unknown, promotes survival in lung cancer | |
SEMA3E | Mutant p95, binds Plexin D1, Inhibits EC survival and migration | p61 active, binds Plexin D1, promotes lung metastasis | |
SEMA3F | p95 active, binds NRP2, competes with VEGFA, repels EC and LEC | c-furSema active, binds NRP1, blocks VEGF binding | |
SEMA3G | p100 binds NRP2 weakly p95 binds NRP2 strongly, binds NRP1 weakly | Inactive |
Full-length SEMA3G (p100) binds to NRP2, processed SEMA3G (p95) binds strongly to NRP2 and weakly to NRP1, and cleaved SEMA3G (p65) does not bind to either receptor [79]. SEMA3B and SEMA3C (either endogenous or recombinant) found in the conditioned media from tumor cells are cleaved and inactive [80, 81], likely due to the high levels of PPC found in tumor cells [82]. However full-length uncleavable SEMA3B protein engineered with a mutant PPC recognition site binds NRP1 or NRP2 and induces endothelial cell repulsion [80]. Similarly, full-length mutant uncleavable SEMA3C binds NRP2 in lymphatic endothelial cells and induces repulsion [81]. Interestingly, the cleaved SEMA3C protein (65 kD) promoted the survival of lung cancer cells, although whether this action is NRP-dependent remains unclear [81]. SEMA3E proteins have dual functions depending on their PPC cleavage status [83]. In its naturally cleaved form, SEMA3E (p61) binds Plexin D1 and associates with ErbB2 to promote lung metastasis and growth [73, 84]. However, when engineered to an uncleavable form, mutant SEMA3E (p95) activated Plexin D1 and inhibited EC migration and survival [73].
The three-dimensional structure of the SEMA3F protein is important for its proper binding and function. The “sema” domain in SEMA3F binds to the a domain of NRP2, while the basic domain of SEMA3F binds to the b domain of NRP2 [85]; therefore, it may come as no surprise that SEMA3 proteins can compete with VEGF-A and VEGF-C binding to NRPs [14, 55, 86]. Additionally, furin cleavage in the C-terminal basic region of SEMA3F creates a fragment protein called c-furSema that can bind to both NRP1 and NRP2 and inhibit VEGFA binding to these receptors [87, 88]. It is currently unclear whether SEMA3 proteins can also compete with binding of PDGF, TGFβ, or HGF to NRP, but it would seem reasonable. Since growth factor signaling is generally stimulatory for proliferation, survival, or migration and SEMA3 proteins compete with the binding of these factors, then it is understandable why SEMA3 proteins are often termed “inhibitory” proteins.
Independent of their ability to compete with VEGF family members, SEMA3 proteins trigger a chemorepulsive signal through the interaction of NRPs and large transmembrane receptors called plexins (reviewed by [89, 90]). NRPs are the SEMA3 ligand-binding part of the complex, and plexins are the signal-transducing part of the complex. For instance, the activation of Plexin A1 by SEMA3A/NRP1 or SEMA3F/NRP2 leads to the inhibition of the RhoA pathway and the subsequent depolymerization of F-actin filaments [91, 92]. This “collapsing” phenotype thus inhibits cell motility, migration, and invasion.
Neuropilin Localization
Although the two NRP receptors were first found in neurons, their tissue expression pattern is now appreciated to be much more widely distributed [4, 5, 38, 93]. In the embryonic neuronal system, neural crest cells express both Nrps in a non-overlapping pattern such that Nrp1-expressing cells give rise to sensory and sympathetic ganglia, while Nrp2-expressing cells primarily give rise to sensory neurons [85, 94]. Melanocytes (and melanoma cells), which are derived from neural crest cells, express high levels of NRP2 [95]. In the embryonic vascular system, NRP1 is expressed in arteries, while NRP2 is found in veins and lymphatic vessels [96–98]. NRP receptors are also involved in the guidance and patterning of blood vessels. Thus, it is not surprising that numerous studies using transgenic and mutant mice have established that any of the following can result in abnormal vascular patterning during development: overexpression of Nrp1 [99], constitutive knockout of Nrp1 [100, 101], heterozygous knockout of Nrp1 and Nrp2 [102], or deletion of the cytoplasmic domain of Nrp1 (Nrp1 cyto Δ/Δ) [103]. NRP expression in endothelial cells is plastic and can be affected by hemodynamic flow and ischemia/hypoxic conditions [9, 104, 105]. However, NRPs are dramatically downregulated in endothelial cells after birth when patterning is completed and homeostasis has been established [6]. That being said, both NRP1 and NRP2 can be upregulated in activated adult capillaries during phases of remodeling or angiogenesis [106–109].
Although most published reports on NRPs have focused on their roles in blood vessels or neurons, NRPs are much more strongly expressed in epithelial cells and smooth muscle cells than in endothelial cells or neurons (see Fig. 14.1– Nrp2 +/lacz embyro) [5, 29, 110, 111]. Nearly all epithelial cells express NRP1 including the epidermis (skin) [111, 112], mammary gland [25, 113], prostate gland, intestine, pancreas, and podocytes of the kidney [114]. Therefore, it should come as no surprise that the majority of all carcinomas, which are derived from epithelial cells, express NRP1 (discussed in more detail below). Interestingly, NRP1 expression in epithelial cells facilitates Epstein-Barr virus entry and promotes infection [115].


Fig. 14.1
Neuropilin 2 expression in a Nrp2 +/lacz mouse. Cryosections of wild-type Nrp2 +/+ mouse (a) and heterozygous Nrp2 +/lacz mouse (b) embryos (E18) were stained with X-Gal reagent to detect beta-galactosidase activity. Blue color denotes Nrp2 expression. Neurons in the brain, melanocytes in the skin, endothelial cells in the lung and kidney, and smooth muscle cells in the intestines are all visible
There is some degree of specificity between the expression of NRPs within smooth muscle subtypes such that vascular smooth muscle cells express Nrp1 [59] and visceral smooth muscle including cells from the GI tract and the bladder express predominantly Nrp2 [110]. NRPs are also strongly expressed in immune cells including T cells, macrophages, and dendritic cells [93, 116, 117]. Immunoregulatory CD4+ T cell subsets called Tregs highly express NRP1 [93, 118]. These NRP1+ Tregs may follow the gradient of VEGF in order to infiltrate a tumor. Evidence of this phenotype was seen when Nrp1 was knocked out in CD4+Foxp3+ Tregs, and tumor infiltration was reduced [119].
Neuropilins in Cancer
As mentioned above, most epithelial cells express NRP1; therefore, most carcinomas express NRP1 including carcinomas of the skin (see Fig. 14.2a) [111, 120], tongue [121], breast [122], colon [123], stomach (gastric) [124], endometrium [125], ovary [126–128], prostate [129, 130], liver [28], pancreas [131, 132], kidney [133], and lung [32, 134]. NRP1 is normally found in differentiated epithelial cells but may be upregulated in basal cells in dysplastic tissues and is therefore an early marker of tumor progression [121]. Soluble NRP1 is an early diagnostic marker for cervical cancer and cervical intraepithelial neoplasia (CIN) [135]. NRP2, on the other hand, is not normally found in cells of epithelial origin (Fig. 14.1) but is reportedly upregulated in later stages of carcinogenesis especially in aggressive or metastatic carcinomas [136–138]. NRP2 is highly expressed in cancer cells of neuronal origin such as melanoma cells (Fig. 14.2b) [106], glioblastoma cells [4], neuroblastoma [139], and medulloblastoma cells [140], as well as in some sarcomas [141]. The topic of neuropilins in cancer has been reviewed previously [4, 75, 142–147]. Herein, we focus on the current theories explaining the function of NRPs in tumor cells.


Fig. 14.2
Neuropilin expression in tumor biopsies. (a) The majority of carcinomas highly express NRP1 protein. Shown here is a human cutaneous squamous cell carcinoma biopsy (highly differentiated) stained with anti-human NRP1 antibody. Brown color denotes NRP1 expression, and blue color shows nuclei stained with hematoxylin. Section was photographed at 100×. Panel A is modified from [111]. (b) NRP2 is a marker for melanocytes and melanoma. Nearly all melanomas (and other neural crest-derived tumors) express NRP2. Shown here is a human melanoma biopsy stained with anti-human NRP2 antibody. Brown color denotes NRP2 expression, and blue color shows nuclei stained with hematoxylin. Section was photographed at 40×. Both panels were digitally enhanced for publication
The current paradigm of NRP1/2 function in endothelial cells is that they act as receptors of VEGF family members (VEGF-A, VEGF-C, VEGF-D) and enhance VEGF receptor (VEGF-R1, VEGF-R2, VEGF-R3) tyrosine kinase signaling [17, 35, 45, 55]. The cytoplasmic portion of the NRP protein is small and does not have intrinsic kinase activity. Therefore, NRPs are ligand-binding proteins in the VEGF/NRP/VEGFR2 receptor complex but not signal-transducing proteins. Based on this information, it is unclear what role NRP is playing in carcinoma cells which typically lack expression of VEGFRs. Four potential scenarios have been suggested.
Tumor-Derived NRP Acts as a Reservoir for VEGF
In this model, tumor cells secrete VEGF which can bind to NRP on the tumor cell surface, but this VEGF does not become internalized or signal in an autocrine fashion in the tumor cell since there are no VEGF receptor tyrosine kinases in these cells. The NRP protein acts somewhat like a heparan sulfate proteoglycan on the tumor cell surface in that it can bind VEGF, hold VEGF, and present the VEGF to juxtacrine cells such as endothelial cells. The NRP may help to increase the gradient of VEGF in the local tumor microenvironment as a way to recruit neovessels toward the hypoxic areas of the tumor. Several papers present findings that favor this model. In one report, recombinant VEGF protein given to the tumor cells in vitro had no effect on proliferation or migration of the tumor cells [148], yet when tumor cells are transfected with NRP1 and implanted in mice, the resulting tumors stain strongly for VEGF, and tumor angiogenesis is increased [123, 148, 149]. Alternately, when NRP levels were diminished in tumor cells in vitro by shRNA and then implanted in vivo, tumor size and vascularity were reduced [138, 150].
VEGF Acts as an Autocrine Growth Factor for Tumor Cells That Express NRP and VEGFR1/2
Although it is rare, some tumor cells do upregulate VEGFRs, and in these tumor cells, signaling is similar to endothelial cells. Several studies have reported a VEGF autocrine signaling loop in tumor cells. In breast cancer cells, VEGF was shown to stimulate migration in vitro [122]. Highly metastatic cells that expressed NRP1 also upregulated VEGFR2 after cycling in mice from the primary organ to the metastatic site [130]. These tumor cells that expressed NRP1 and VEGFR2 showed increased proliferation and migration in response to VEGF [130]. In another study, merely repeatedly collecting cells that had invaded through Boyden chambers was enough stress to cause them to upregulate VEGFR2 and NRP1 [32]. Likewise, lung cancer cells that endogenously expressed VEGFR2 and were transfected with NRP1 showed increased growth in nude mice compared to control transfected cells [151].
VEGF Acts as an Autocrine Growth Factor for Tumor Cells That Express NRP via Synectin Signaling
New studies suggest that NRPs may indeed “signal” but not in the canonical fashion of tyrosine phosphorylation (reviewed by [152]). As discussed above, the intracellular domains of both NRP1 and NRP2a proteins terminate in the same three amino acids, SEA, and bind to the PDZ domain of the cytoplasmic scaffold protein, synectin [22–24]. Yoshida and colleagues show that squamous cell carcinoma cells which express NRP1 but do not express any VEGFR can proliferate in the presence of VEGF and that this proliferative signal is mediated by the interaction of synectin and Syx, a RhoGEF [153]. This autocrine signaling through VEGF/NRP1 resulted in the activation of RhoA and the degradation of p27, a cyclin-dependent kinase inhibitor. When the authors deleted the cytoplasmic domain of NRP1 in these tumor cells, this signaling was lost [153]. VEGF/NRP1 direct interaction was also shown to be critical in epidermal stem cells for the initiation of skin cancer [120, 154]. Similar autocrine signaling was observed between PGF and NRP1 in medulloblastoma tumor cells [155].
NRP Is an HGF Receptor in Cancer Cells
Although the majority of all research on NRP has focused on its role as a VEGF receptor, this may not be its main function in carcinoma cells. While carcinoma cells rarely express VEGFRs, they typically express high levels of c-Met, the tyrosine kinase receptor for HGF (discussed above). HGF is a potent oncogene and tumor promoter, and HGF-induced invasion in human glioma [156] and human pancreatic cancer [157] is dependent on NRP1. NRP1 depletion using shRNA in gastric cancer cells inhibited the VEGF/VEGFR, the EGF/EGFR, and the HGF/c-Met pathways [158]. Peptide N, a small protein corresponding to the N-terminal domain of human HGF that binds to NRP1 and inhibits the binding of endogenous VEGFA or HGF, inhibited hepatocellular carcinoma progression in a transgenic model [18, 28, 159].
Neuropilin as a Biomarker
As outlined above, NRPs are expressed in cancer cells, in tumor-associated endothelial cells that participate in neoplastic angiogenesis, in tumor-associated lymphatic endothelial cells that contribute to lymphangiogenesis and metastasis , and in tumor-infiltrating immune cells. NRP1 may be a potential biomarker in many different carcinomas. In some cases the expression of NRP1 merely increases in the early stages of transformation but is still found at a lower level in the surrounding epithelial cells [111, 123], in other cases the localization of the NRP1 protein may change within the epithelium from differentiated cells to basal cells [121], and in still other tissues, normal epithelial cells may lack NRP1 expression entirely but upregulate expression in carcinoma cells—such is the case in the liver [28]. NRP1 copy number gain (CNG) as analyzed by fluorescence in situ hybridization (FISH) in non-small cell lung cancer biopsies correlated with a worse overall survival (OS) and progression-free survival (PFS) [160]. Beyond carcinomas, NRP1 also serves as a novel biomarker associated with poor prognosis in acute myeloid leukemia patients [161].
In a screen of biomarkers for lung tumors induced by benzo[a]pyrene (coal tar), serum NRP2 was found to be highly expressed [162]. NRP2 has been shown to be a novel biomarker for aggressive human melanoma [95]. Furthermore, NRP2 gene silencing in human melanoma cells decreased tumorigenicity and metastasis in a preclinical trial [163].
Based on the aforementioned studies, NRPs are excellent candidates for targeted therapies in multiple forms of cancer. Potential strategies used to target NRPs have been reviewed previously [164–166]. Most successful have been antibodies targeting the VEGF-binding domain of NRP1 or NRP2 [107, 108]. When used in combination with bevacizumab (anti-VEGFA antibodies), anti-NRP1B antibodies were shown to inhibit tumor size, tumor angiogenesis, and tumor progression [107]. These antibodies were later humanized and tested in a phase Ib clinical trial with bevacizumab and chemotherapy [167]. Unfortunately, this combination therapy caused a high degree of proteinuria in patients—likely due to the off-target effect of anti-NRP1 on podocytes in the kidney [114]. Antibodies to NRP2 were also shown to be efficacious at blocking lymphangiogenesis and metastasis in preclinical trials [108] and have not been tested to date in human clinical trials.
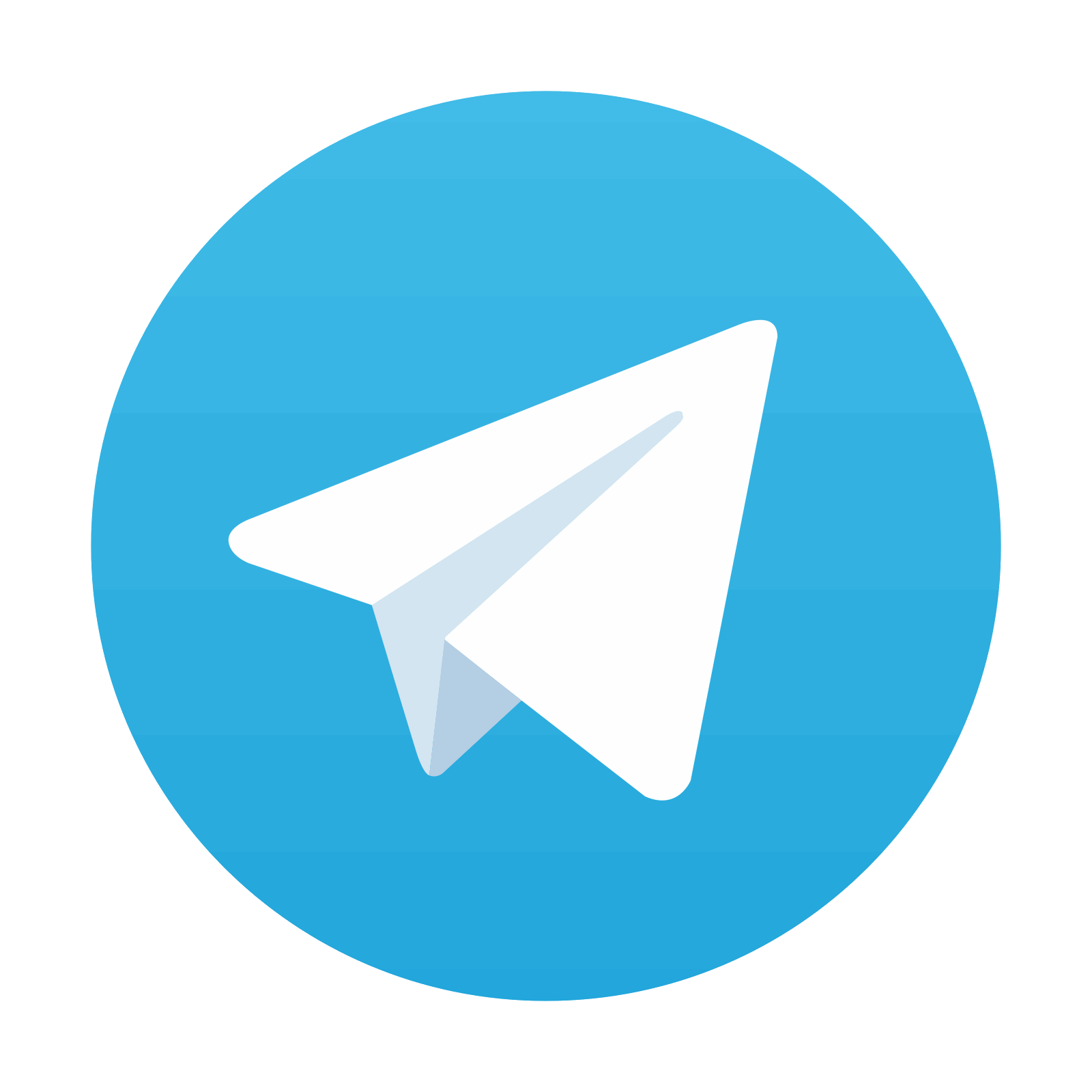
Stay updated, free articles. Join our Telegram channel

Full access? Get Clinical Tree
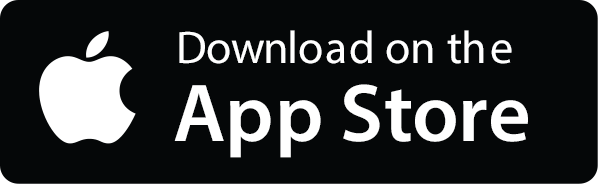
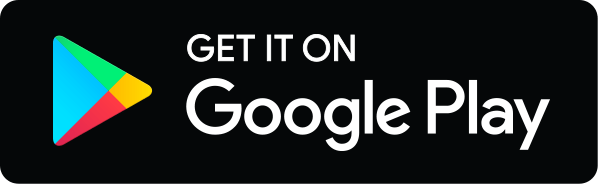