Introduction
Despite modern advances in the diagnosis and management of infective endocarditis (IE), inpatient mortality rate for patients with IE remains high at 15%–20% [ ]. In IE patients with neurologic complications, mortality has been reported to be as high as 45% [ ]. In literature, the prevalence of neurologic complications in patients with left-sided IE ranges from 20% to 80% in literature as the definition of “neurologic complication” varies from study to study [ ]. Nonetheless, when they do occur, neurologic complications have major implications for the management of IE.
Cerebral emboli/ischemic stroke
Embolic cerebral infarction is by far the most common neurologic complication of left-sided IE. Well-recognized predictors of cerebral embolism are vegetations located on the anterior mitral valve leaflet, left-sided vegetation >10 mm in length, and severe vegetation mobility [ ]. Staphylococcus aureus is not only the most common causative organism of native-valve IE, but also of IE-associated cerebral infarction [ , , ]. Fungi and beta-hemolytic streptococcal species are rare pathogens that give rise to large vegetations and therefore increase cerebral embolism risk [ , , ].
Large cohort studies observed increased stroke risk in patients with IE as early as 40 days before their IE diagnosis [ , , ]. Contemporary data indicate that 29%–47% of IE patients initially presented with stroke symptoms [ , , ]. It has been postulated that the pathogenesis of stroke in these patients is related to systemic inflammation, which creates a proinflammatory milieu for accelerated atherosclerosis and thromboembolic events [ , ]. Initiating the appropriate antimicrobial treatment is by far the most beneficial medical therapy for reducing septic embolism [ , ]. In one prospective cohort study, antimicrobial therapy decreased the incidence of embolic stroke from 4.82 per 1000 patient days in the first week of therapy to 1.71 per 1000 patient days in the second week [ ]. Antiplatelets were studied as an adjunctive therapy to antibiotics in mitigating the risk of septic embolism. Promising data from animal models of IE suggested that acetylsalicylic acid may facilitate the resolution of valve vegetations [ , ]. However, in a double-blinded randomized control trial (RCT) of 115 IE patients, frequencies of embolic cerebral infarcts were similar in patients who received aspirin 325 mg for 4 weeks after their IE diagnosis and those taking a placebo. Although no difference was observed in rates of intracranial hemorrhage (ICH), the aspirin arm had more bleeding complications overall compared to placebo [ ]. A subsequent retrospective study assessed the effect of long-term antiplatelet therapy on embolization and found that embolism was lower in IE patients who had been on continuous antiplatelet therapy daily for at least 6 months prior to their IE diagnosis [ ]. While this study demonstrated an association between long-term daily antiplatelet therapy and reduced frequency of embolism, it did not provide any data on the risk of ICH in IE patients on continuous antiplatelet therapy. The decision to continue antiplatelet therapy therefore rests on each patient’s unique risk–benefit profile.
Ischemic infarcts associated with IE resemble other causes of cardioembolic infarction with regard to imaging patterns. The majority of embolic infarcts associated with IE are clinically asymptomatic and appear as a single punctate lesion with restricted diffusion or multiple tiny foci scattered throughout the brain parenchyma ( Fig. 10.1 ) [ , , ]. A distinction is made between symptomatic embolic stroke (including transient episodes of neurologic deficits) and silent infarcts. Embolic stroke occurs in 20%–40% of patients with IE [ , , ], whereas 48%–82% of IE patients have silent infarcts on magnetic resonance imaging (MRI) [ , , , , ]. Silent infarcts, although clinically asymptomatic by definition, have implications for the urgency and safety of valve surgery as well as long-term neurologic outcome. Septic embolization can occlude large intracranial vessels and cause not only debilitating symptoms but also delay in surgical planning. In one of the largest brain MRI studies of patients with acute IE, 25% of the cohort (33/130 patients) had a considerably large infarct as a result of a large-vessel occlusion (LVO) [ ].

Reperfusion therapy
Although endocarditis was not an exclusion in the original National Institute of Neurological Disorders and Stroke intravenous tissue plasminogen activator (IV tPA) trials, the use of IV thrombolysis (IVT) in IE patients with acute ischemic stroke was uncommon [ , ]. Case reports of favorable outcomes following IVT in IE patients are challenged by retrospective studies and systematic reviews [ ]. Using National Inpatient Sample data, Asaithambi et al. published the largest study to date comparing rates of postthrombolytic hemorrhage between IE and non-IE patients and cautioned against the use of IVT for acute ischemic stroke in IE patients. In their cohort, 20% (44/222) of IE patients with acute ischemic stroke who were treated with IVT had postthrombolytic hemorrhage, compared to 6.5% (8730/134,048) of patients without IE. Systematic reviews have yielded congruent findings regarding thrombolysis-related ICH [ , ]. In a newly published review, Bettencourt and Ferro determined that the risk of postthrombolytic hemorrhage was four times higher in IE patients who received IVT [ ]. Currently, The American Heart Association/American Stroke Association (AHA/ASA) does not recommend IV alteplase for the treatment of acute ischemic stroke in patients with IE [ ].
The efficacy of mechanical thrombectomy (MT) for treating proximal LVO associated with IE is not well established. Bacterial IE and presumed septic emboli were explicitly excluded in two of the five MT randomized controll trials published in 2015, as well as landmark trials of late window MT, DEFUSE 3, and DAWN [ ]. Case series and systematic reviews have given credence to MT as a tenable treatment option for IE-associated proximal LVOs. In two case series that assessed reperfusion grade, rates of successful recanalization, defined as Thrombolysis in Cerebral Infarction (TICI) grade 2B or better, were 66% and 83%, respectively [ , ]. Pooled data from published case series and individual case reports suggest that MT may be a promising therapy for IE-associated LVOs. Moreover, MT appeared to be fairly safe in this patient population in terms of postprocedural ICH [ ]. In Marquardt et al.’s systematic review, the frequency of postprocedural ICH was markedly lower in patients who underwent MT for IE-associated LVOs when compared to those who received IVT [ ]. While not founded on RCTs, MT may still be of value for patients with LVOs secondary to septic emboli.
Intracranial hemorrhage
ICH in patients with IE is associated with an increased risk of in-hospital mortality [ , ]. Its management is nuanced as ICH is not a single entity. Rather, ICH encompasses hemorrhagic cerebral complications that differ in etiology and clinical significance. Our current understanding of the mechanisms by which ICH occur in IE patients is based on histopathologic analyses of postmortem brain. Proposed mechanisms are: (1) hemorrhagic transformation of ischemic infarcts, potentiated by underlying coagulopathy (acquired or otherwise); (2) septic emboli that cause pyrogenic arteritis; (3) bacterial invasion of arterial wall, leading to formation of infectious intracranial aneurysm (IIA) [ ]. One or more of these mechanisms may underlie the development of intraparenchymal hemorrhage (IPH), subarachnoid hemorrhage (SAH), and cerebral microbleed (CMB) in patients with IE.
Intraparenchymal hemorrhage
IPH in patients with IE can arise as either a hemorrhage, or “coagulum,” within an infarct bed from hemorrhagic transformation [ ], or as a primary intraparenchymal hematoma. Henceforth, the discussion of IPH will focus on the latter. The prevalence of primary IPH in patients with IE is usually quoted to be between 3% and 9% [ , , , , ], but much higher numbers have been observed, specifically in imaging-based studies of critically ill patients with IE [ ]. In practical terms, IE-associated primary IPH is caused by rupture of intracerebral vessels and the subsequent formation of a hematoma [ ]. This process is no different from the formation of IPH in the general population [ ], but the mechanisms by which vessels become prone to rupture in IE patients are distinctive. While IIA has gained notoriety for causing fatal IPH, it is not the leading cause of IPH in the IE population. Instead, pyrogenic arteritis is thought to be responsible for most cases of primary IPH in patients with IE [ ]. The pathophysiology was elucidated in two remarkable studies, which described purulent inflammatory cells infiltrating the wall of an artery in response to septic microemboli within the arterial lumen [ , ]. The affected artery becomes eroded, then ruptures, resulting in hemorrhage. Extravasated blood triggers an inflammatory cascade that leads to further breakdown of surrounding vessels [ ]. Staphylococcus aureus IE has a propensity for causing IPH in IE patients early in the course of their disease by the mechanism of pyrogenic arteritis [ , , , ]. Antithrombotic agents and underlying coagulopathies can also potentiate IPH formation in patients with IE [ , , , ].
Cerebral embolization is not only a necessary substrate for pyrogenic arteritis, but also for IIA. Fragments of septic emboli carry bacteria into cerebral vessels or the vasa vasorum. An invasion of the arterial wall by bacteria then ensues, disrupting the integrity of the arterial wall. In animal models, bacteria from the vasa vasorum breached the adventitia and spread through the tunica media to the internal elastic membrane [ ], thereby creating a focal aneurysmal dilation. An IIA is a pseudoaneurysm and precipitates both IPH and SAH. Diagnosis and management of IIA is discussed separately in Infectious intracranial aneurysm section.
Subarachnoid hemorrhage
Prior studies reported that SAH was observed in 1%–3% of IE patients [ , , ]. It is thought to occur early in the course of patients’ valve disease. In one series, SAH was identified either before or concomitant with acute IE diagnosis in six of eight patients. The remaining two patients developed an SAH within 3–7 days of their IE diagnosis [ ]. It is unclear to what extent IIA rupture is responsible for causing SAH in IE patients. Rupture of a small occult IIA may leave behind no evidence of its aneurysmal origin. SAH can also arise from blood leaking out of an unruptured IIA [ ]. Similarly, pyrogenic arteritis can cause focal hemorrhage into the subarachnoid space [ ]. Confusion and focal neurologic deficits, accompanied by diffuse headaches, are common presenting symptoms of SAH in IE patients [ , ]. Rarely, a high-grade SAH occurs secondary to a ruptured IIA, causing patients to seize and rapidly deteriorate [ ].
Cerebral microbleeds
In patients with active IE, the most common abnormal finding on their MRI is CMBs. Present in 50% of IE patients [ , , ], CMBs appear as round, hypointense foci on T2∗-weighted sequences, e.g., gradient echo and susceptibility-weighted images (SWIs) [ , ]. They usually measure ≤5 mm in diameter and have a cortical predominance in patients with IE. CMBs are observed in a number of pathologies other than IE and arise from different pathophysiologic mechanisms [ , ]. These mechanisms converge upon the eventuality of blood extravasating from damaged blood vessels, followed by phagocytosis of hemosiderin by macrophages [ , ]. In the IE patient population, it has been postulated that CMBs represent infectious microangiitis [ ], which is akin to pyrogenic arteritis, but instead involves capillaries and arterioles.
The significance of CMBs in IE patients centers primarily around ICH risk. Okazaki et al. observed that the presence of two or more CMBs was an independent risk factor for symptomatic ICH within 3 months [ ]. IE patients who developed ICH had median 11 CMBs (interquartile range [IQR] 5–13) compared to zero CMBs (IQR 0–1) in patients without ICH. It should be noted that in this study, there was no difference in age and frequencies of hypertension or antithrombotic use between ICH and non-ICH patients. It remains unclear whether or not there was a difference in time interval from valve surgery to the onset of ICH between the two groups. In a larger study, Murai et al. contended that CMBs were not associated with an increased risk of symptomatic hemorrhage after valve surgery [ ]. Hess et al. also found no association between CMBs and parenchymal hemorrhages in their cohort [ ]. Nevertheless, for IE patients with multiple CMBs and neurologic deficits, caution is warranted when making decisions about anticoagulation and surgical timing.
Infectious intracranial aneurysm
IIA, commonly known as mycotic aneurysm, is a dreaded complication of IE for its potential for causing catastrophic ICH. IIAs are clinically recognized in 2%–10% of IE patients [ ], but their true prevalence is unknown since cerebral angiography is not routinely performed as it is an invasive procedure. Additionally, IIAs can recede and even resolve with antibiotic therapy [ ]. Diagnostic considerations of IIA pertain to when and how to optimally evaluate for IIA. Management strategy factors in aneurysm morphology, location, and clinical equipoise.
As previously discussed, IIAs are thought to arise from the disruption of arterial wall secondary to bacterial invasion and focal inflammation [ ]. They are usually small in size (<5 mm) and have a thin, friable wall with a fusiform or saccular morphology ( Fig. 10.2 ) [ ]. They have a predilection for distal cerebral arteries, most commonly branches of the middle cerebral artery [ ]. Focal neurologic deficits are observed in 15%–30% of IIA patients at their time of presentation [ , ]. Patients may present with changes in mental status or seizures, heralding an active IIA that leaks blood or is close to rupture [ ]. However, they are usually asymptomatic until they cause devasting neurologic injury at the time of rupture [ , ].

The evaluation of IIA should start with a noncontrast computed tomography (CT) of the brain. Presence of intracranial blood on noncontrast CT brain necessitates dedicated vessel imaging [ ]. Similarly, patients with certain brain MRI findings should be evaluated for IIAs. Specifically, Cho et al. described that CMBs with contrast enhancement and larger sulcal SWI lesions with or without contrast enhancement were associated with IIAs [ , ]. The absence of hemorrhage on MRI, as Monteleone et al. previously reported, has a strong negative predictive value for presence of IIA; ergo, vessel imaging is not essential in this setting [ ]. Digital subtraction angiogram (DSA) remains the gold standard for detecting IIAs, but it is typically reserved for IE patients with ICH or when suspicion for IIA is high. CT angiography (CTA) is a noninvasive imaging modality that is attractive for its availability and convenience. Large meta-analyses of single-section CTA studies reported sensitivity of at least 90% and specificity of 77%–86% [ , ]. Multisection CTA has further improved the sensitivity and specificity of CTA. Wintermark et al. reported 94% sensitivity for IIAs that measured 3–4 mm and an overall specificity of 95% [ ]. As an alternative to CTA, MR angiography (MRA) spares patients exposure to radiation and iodinated contrast. Advancements in the diagnostic performance of MRA have improved the sensitivity of MRA to rival that of CTA in diagnosing intracranial aneurysms [ , ]. Even so, neither modality is infallible. Diagnostic accuracy is ultimately influenced by aneurysm size, location, environment, the use of reconstruction algorithms, as well as the experience of the center performing and interpreting the imaging studies [ ]. Society guidelines recommend performing DSA when suspicion for IIA remains high despite “negative” results from noninvasive imaging modalities [ , ].
Therapeutic considerations of IIA
At present, guidelines for the treatment of IIAs do not exist, but the treatment goal is clear: to reduce aneurysm size and prevent aneurysm rupture. Regional and institutional practices vary, but the general consensus on treatment is antibiotic therapy with or without endovascular intervention or open surgery. A 4- to 6-week course of pathogen-specific antibiotic therapy has been the mainstay of IIA treatment [ , , ]. A number of substantial case series of unruptured IIAs found that even though IIAs can decrease in size or completely resolve, the overall response of IIAs to antibiotics was variable [ , , ]. For example, Corr et al. reported that while one-third of their cohort had complete resolution, another one-third had no change in aneurysm size. Further, 17% of their cohort had enlarged IIAs while on antibiotics on follow-up imaging [ ]. The clinical course during antibiotic treatment is unpredictable, as evidenced by cases of IIA rupture while on antibiotic therapy in the abovementioned case series. Conservative management with antibiotics alone has become a progressively less popular treatment strategy, notably in the last 10 years [ ]. Current literature supports antibiotics in combination with endovascular or neurosurgical therapies [ , ], albeit there are circumstances in which antibiotic therapy is the only treatment option. Unstable clinical condition, medical comorbidities, and proximity of IIA to an eloquent cortex are some common scenarios. For these patients, serial imaging of IIA at an interval of 7–14 days is advisable [ ].
The contemporary armamentarium for treating IIA has been transformed by advancement in endovascular therapy. A plethora of case series from recent years has placed endovascular approaches in the forefront. Direct embolization of IIAs with coils or flow-diverter stents is an established strategy for treating intracranial aneurysms and usually chosen for proximal IIAs. Liquid embolization, using n-butyl cyanoacrylate or ethylene-vinyl alcohol copolymer (Onyx®), is a novel alternative whose safety and efficacy has been welldescribed [ ]. It may be an attractive strategy for addressing multiple IIAs, which were seen in 25%–30% of cases in large case series [ ]. For distally located IIAs or aneurysms with a complex morphology, liquid embolization agents or coils are used to occlude the parent artery. This method is referred to as the indirect approach. Although there is a risk of intraprocedural aneurysm rupture, endovascular treatment of IIAs is less invasive than open neurosurgery. Treatment preferences have naturally shifted in the last 5 years toward favoring endovascular therapy for treating IIAs, including ruptured IIAs [ ]. While decades-old studies reported mortality close to 80%, data from the last several years indicated improved survival following IIA rupture with mortality between 12% and 30% [ ].
Nonetheless, open neurosurgical approaches remain a valuable treatment strategy for a subset of patients. Open surgery is well-suited for young patients with low surgical risk whose IIAs are surgically accessible. IIA growth despite adequate antibiotic therapy and endovascular intervention may require direct neurosurgical intervention. Clipping or excision of an IIA should be considered in patients who are undergoing decompressive craniectomy for hematoma evacuation. However, neurosurgical treatment of IIAs can be technically challenging. First, IIAs involving distal branches of the anterior circulation can be difficult to identify and/or access during open surgery [ ]. Second, friable walls and poorly defined aneurysm necks can render surgical clipping unfeasible. In the absence of RCTs, treatment strategies will largely be determined by expert opinions and institutional or regional practices. High-volume, experienced centers with multidisciplinary experts are best suited to manage IIAs in patients with IE.
Implications of stroke on surgical timing
The American Heart Association and American College of Cardiology (AHA/ACA) and American Association for Thoracic Surgery (AATS) guidelines recommend surgical treatment of IE for patients with large mobile vegetations, paravalvular extension, persistent sepsis, or recurrent systemic embolization. Heart failure and severe valve dysfunction secondary to IE also warrant surgical intervention, as does prosthetic-valve IE [ , , ]. There is uncertainty, however, concerning the urgency of valve surgery and optimal surgical timing. Timing of valve surgery after an acute ischemic or hemorrhagic stroke is a topic of ongoing debate. Benefits of urgent or early surgery are weighed against the risk of postoperative neurologic deterioration secondary to hemorrhagic transformation of infarcted tissue or expansion of an existing ICH secondary to intraoperative heparinization.
Existing literature offers varying perspectives on surgical timing, ranging from 72 hours after an initial neurologic event to delaying surgery by 2–4 weeks [ ]. In a systematic review, Angstwurm et al. calculated the risk of neurologic deterioration relative to surgical timing [ ]. For ischemic infarcts, the risk of neurologic deterioration in patients who underwent surgery within 3 days of infarction was 15%–35% and 20%–50% when surgery was performed between day 4 and 14. This risk decreased to <10% after 2 weeks and fell to 0.4% after 1 month. A similar trend was observed by Eishi et al. in a retrospective multicenter study [ ]. Rates of mortality and neurologic deterioration were 31% and 44% in patients who underwent surgery during the first week of their initial ischemic stroke and 10% in those who had valve surgery 2 weeks after. The practice of delaying surgery by at least 2 weeks was reinforced by Gillinov et al. in their notable 1996 retrospective series, in which they proposed delaying valve surgery by 2–3 weeks in patients with embolic strokes [ ]. Their experience, however, was based on a cohort of just 34 patients, of whom nearly half were IV drug users. As García-Cabrera et al. later pointed out, little is known about the severity of ischemic stroke in these early studies. In their multicenter observational study, stroke severity was defined by radiographic parameters [ ]. Twenty-eight percent (15/54) of patients with moderate to severe ischemic lesions, defined as “multiple cerebral embolisms or a single embolism affecting ≥30% of a brain lobe,” underwent valve surgery. The postoperative morality rate in patients who had surgery within 2 weeks of a moderate to severe ischemic stroke was 40% (2/5), compared to 20% (2/10) when surgery was delayed by 2 weeks.
In 2012, Kang et al.‘s monumental RCT showed that early surgery (within 48 h from randomization) reduced patient mortality from embolic events or other causes in IE patients with ischemic stroke [ ]. Their RCT, together with prospective studies, called into question the practice of delaying valve surgery after an acute ischemic stroke. First, data from Thuny et al.‘s study indicated that the prevalence of postoperative neurologic complication was markedly lower than previously reported. In their cohort of 63 patients with IE and acute stroke, 6.3% (4/63) of patients had a postoperative neurologic complication after undergoing valve surgery at median 9 days [ ]. In addition, postoperative neurologic complications were limited to patients with ischemic or hemorrhagic strokes, not transient ischemic attacks (TIAs) or silent infarcts. Although the overall mortality was higher in IE patients with stroke, only one patient died as a direct result of his or her stroke. Their work demonstrated that stroke was not a contraindication to valve surgery, and more importantly, surgery need not be delayed by TIAs and silent infarcts. The latter conclusion is a Class I recommendation in the European Society of Cardiology guidelines [ ]. Second, in a robust multicenter prospective study of 198 IE patients with predominantly ischemic stroke, Barsic et al. observed no survival benefit in delaying valve surgery when it was indicated [ ]. After adjusting for age, paravalvular abscess, and heart failure, valve surgery within 1 week of ischemic stroke did not increase in-hospital mortality rate. Regarding postoperative neurologic complications, two prospective MRI-based studies reported zero cases in their cohort, among whom one-fourth of patients suffered an acute ischemic stroke before valve surgery. Median time from stroke to surgery was 6 and 8 days, respectively [ , ]. In view of these studies, AHA/ACA guidelines were updated in 2017 to the position that surgery without delay “may be considered” in IE patients with an indication for surgery if their ischemic stroke did not cause “extensive neurological damage (Class IIB) [ ].
Retrospective studies published in the last 5 years have contributed to a growing body of evidence for early surgery. Recent studies suggest that valve surgery performed between median 4 and 10 days carries a low risk of neurologic deterioration and mortality [ ]. Factoring in infarct volume, our prior study reported that ischemic infarcts were not associated with poor postoperative neurologic outcome, irrespective of volume [ ]. Median infarct volume (excluding punctate infarcts) was 12.9 cm 3 (IQR 6–17 cm 3 ). In a related study, Oh et al. compared postoperative hemorrhagic complications and neurologic outcomes of early (≤7 days of IE diagnosis) and late surgical intervention in patients who had acute ischemic infarcts, which were categorized by vascular territory involvement [ ]. For instance, infarction of territory supplied by small cerebral arterial branches was regarded as a “small” infarct, whereas a “moderate” infarct was defined as territory supplied by a major arterial branch, and “large” as an entire vascular territory. In their study, early surgery did not lead to an increase in poor neurologic outcome or mortality, regardless of infarct size. While retrospective studies should be interpreted with care, benefits of early surgical intervention in patients with compelling indications for surgery likely outweigh the risk of postoperative neurologic complication associated with small- to medium-sized infarcts.
Data on postoperative neurologic outcome in IE patients with ICH remain scant. Angstwurm et al. calculated the rate of neurologic deterioration to be 15% in patients who underwent surgery 4 weeks after an ICH [ ]. Findings from García-Cabrera et al.’s 2013 multicenter study heightened concerns about poor postoperative outcome in IE patients with ICH [ ]. Mortality was 75% in patients who underwent surgery within 4 weeks of an ICH, which encompassed IPH, hemorrhagic transformation, and SAH. Mortality rate was still high at 40% when surgery was performed 4 weeks after an ICH. The safety of valve surgery following ICH has been reevaluated by numerous studies published in the last 5 years. In a 2016 multicenter study, in-hospital mortality rate of IE patients who underwent valve surgery on median 21 days after their ICH was 5.6% [ ]. A subsequent 2018 study found that valve surgery conferred a mortality benefit to IE patients with ICH when compared to patients who were treated conservatively [ ]. Median time from ICH to surgery was 34 days. These observational studies all share one major limitation: data analysis did not take hemorrhage volume into account. In our retrospective study, the median hematoma size of patients without perioperative neurologic complications was 2.8 cm 3 (IQR 1.1–8.1). Admittedly, all of these studies were subject to selection bias. Even so, they compel us to reexamine current recommendations and practice guidelines. Delaying surgery by at least 1 month may not be advisable for some IE patients who have a strong indication for valve surgery. Cardiovascular ramifications of surgical delay must be considered, particularly consequences of ongoing cardiac structural deterioration such as abscess formation, valve destruction, and conduction block.
Conclusions
The clinical course of IE is one that is often complicated by its neurologic manifestations. The heterogeneity and complexity of IE-associated cerebrovascular complications necessitate a multidisciplinary team approach at experienced centers. Insights gained from the last decade have tipped the balance in favor of early valve surgery (within 14 days) for IE patients with stroke. Caution is warranted for patients with large ischemic strokes and ICHs, and further research is needed to determine optimal timing of surgery for patients with such complications. Treatment decisions, while guided by society and consensus guidelines, should be tailored to individual patients.
References
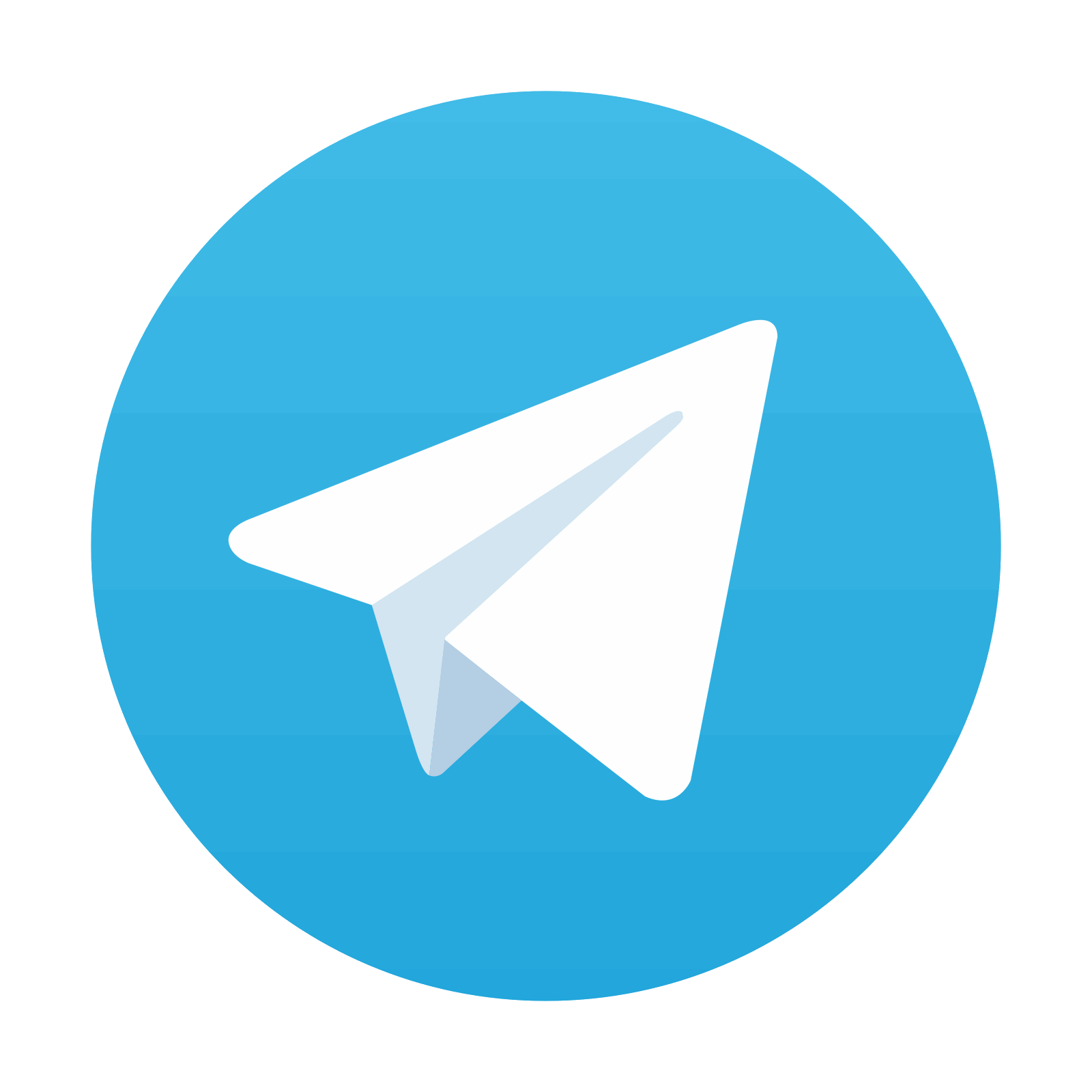
Stay updated, free articles. Join our Telegram channel

Full access? Get Clinical Tree
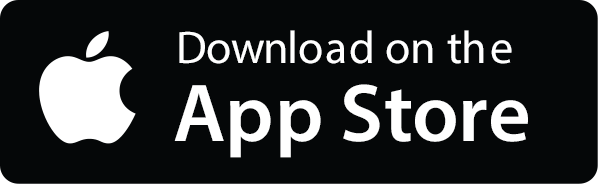
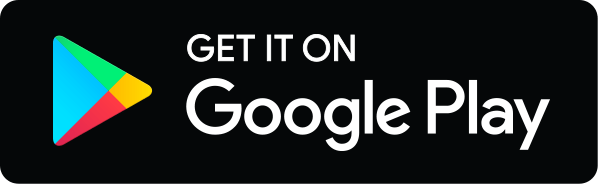
