Acknowledgments
We thank the National Institute on Alcohol Abuse and Alcoholism for its support through the Neurobiology of Adolescent Drinking in Adulthood (NADIA) consortium (AA020024, AA020023), the Bowles Center for Alcohol Studies (AA011605), and the U54 collaborative partnership among NCCU, and UNC (AA019767), and K08-AA024829.
Innate Immune Signaling in the Brain
Immune Cells of the Brain
Historically the brain has been considered an “immune privileged” organ, meaning it is protected from immune activation that occurs in the periphery. Currently, this is largely considered true; however, the brain has its own immune defenses. The resident immune defenses in the brain are known to be composed of innate immune responses. This allows for recognition and elimination of viral, bacterial, and fungal pathogens. Historically, immune function in the brain has primarily been considered the role of glial—microglia and astrocytes. Microglia are the resident macrophages of the brain and derive from mesodermal tissue-specific monocytes. Microglia transition from a resting state to various stages of activation in response to infections, stressors, and drugs of abuse such as alcohol and cocaine. a
a References .
Stages of microglial activation have been traditionally classified as M1 (proinflammatory) and M2 (antiinflammatory). M1 activation is associated with the release of canonical proinflammatory cytokines such as tumor necrosis factor α (TNFα), interleukin 1β (IL-1β), and IL-6, as well as generation of reactive oxygen species (ROS) through increased inducible nitric oxide synthase (iNOS) and nicotinamide adenine dinucleotide phosphate (NADPH)-oxidase expression. The M2 state is associated with the release of canonical antiinflammatory cytokines such as IL-10 and IL-4. Microglia also regulate physiological processes in the healthy brain, such as synaptic pruning, debris clearance, immune surveillance/defense, and neurogenesis. Astrocytes are another important cell type in the neuroimmune system. Astrocytes express immune receptors and cytokines in response to immune activation. Astrocytes undergo an activation known as reactive gliosis to help limit tissue damage in different contexts. Some suggest that astrocytes adopt proinflammatory and antiinflammatory states, similar to microglia. Astrocytes are also involved in numerous physiological processes, such as maintenance of fluid homeostasis, metabolic support of neurons, and modulation of synaptic transmission through uptake of glutamate. Both alcohol and cocaine also cause astrocyte activation. It is important to note that both microglia and astrocytes regulate synaptic plasticity. Thus their activation by drugs of abuse might result in synaptic changes and neuronal firing. Of interest, neurons have also been proposed to play a role in innate immune responses through modulation of glia and the induction of cytokines. In addition, a variety of cytokine receptors, such as those for, suggesting that neurons respond to cytokines. Indeed, IL-1β, monocyte chemoattractant protein-1 (MCP-1) and other immune-signaling molecules alter neuronal firing and modulates γ-aminobutyric acid (GABA) transmission. These studies indicate that in the brain, cytokines and other immune-signaling molecules modify synapses and neurocircuits similar to neurotransmitters.Pattern Recognition Receptors and Their Ligands
The innate immune system functions to recognize foreign pathogens for their elimination. These pathogenic elements are detected by pattern recognition receptors (PRRs). These receptors recognize specific molecular signatures associated with bacteria and viruses, termed pathogen-associated molecular patterns (PAMPs). PRRs are promiscuous receptors that have also been found to recognize endogenous molecules associated with cell stress or trauma, known as damage-associated molecular patterns (DAMPs). This is considered “sterile” inflammation, when innate immune activation occurs without the presence of a foreign pathogen. The release of DAMPs has been implicated in the pathologies of numerous peripheral immunological diseases. DAMP release also occurs in the brain, which is normally a sterile environment. PRRs play critical roles in addiction pathology. To date, five classes of PRRs have been identified including: Toll-like receptors (TLRs), C-type lectin receptors, nucleotide binding domain receptors (leucine-rich repeat containing or nucleotide-binding oligomerization domain [NOD]-like receptors), RIG-I-like receptors, and absent in melanoma 2 (AIM2)-like receptors . TLRs are the most studied PRRs, and have been implicated in both cocaine and alcohol addiction. To date, 10 TLRs have been identified in humans and 12 in mice. TLR ligands include a variety of molecules from bacterial endotoxin to mammalian high-mobility group box 1 (HMGB1) and heat shock proteins ( Table 20.1 ). TLRs are characterized by an N-terminal extracellular leucine-rich repeat sequence and an intracellular Toll/IL-1 receptor/resistance motif (TIR). TLR signaling operates through key adapter proteins that initiate the signaling cascade upon ligand recognition. All TLRs, except for TLR3, utilize the MyD88 adapter protein complex. TIRAP/MyD88 complex formation causes activation of the IL-1 receptor–associated kinases (IRAKs) and the TNF receptor–associated factor 6 (TRAF6) leading to IκB and MAPK activation. IκB and MAPK activation result in activation of the nuclear factor kappa-light-chain-enhancer of activated B cells (NF-κB) and activated protein-1 (AP-1) transcription factors, respectively. These transcription factors regulate the expression of proinflammatory cytokines that propagate and magnify the immune response. Because TLRs share common intracellular signaling with several cytokines, subsequent cytokine release leads to an amplification of immune responses. Activation of these transcription factors is involved in addiction (detailed below). TLR signaling was initially described in peripheral immune cells. Thus the precise signaling pathways for the TLRs in each brain cell type have yet to be delineated. Both microglia and astrocytes appear to show canonical TLR4 signaling in response to ethanol resulting in NF-κB activation. However, responses in neurons are poorly understood. There is debate on whether neurons are capable of activating NF-κB. Some suggest that neurons do,not while others find that NF-κB activation in glutamatergic and cultured neurons regulates plasticity, learning, and memory. Furthermore, activated NF-κB subunit colocalizes with dorsal horn spinal neurons and different neuronal cell lines exhibit NF-κB-dependent regulation of μ-opioid receptor expression.
TLR | Foreign Immunogen | Endogenous TLR Ligand | Neuropsychiatric Disease |
---|---|---|---|
2 | Bacterial di- and tri-acylated polypeptides Gram (+) lipoglyans | α-Synuclein | Alcoholism Parkinson disease |
3 | dsRNA | Stathmin, dsRNA | Alzheimer disease Multiple sclerosis |
4 | Bacterial endotoxin Peptidoglycans | HMGB1 HSPs 60, 70/72 | Alcoholism Cocaine abuse Stroke, traumatic brain injury Chronic pain |
7 | ssRNA | Let-7, miR-21 | Alcoholism Alzheimer disease Chronic pain |
A key feature of TLR signaling is the induction of proinflammatory DAMP release. These DAMPs can subsequently further TLR activation by binding to their respective receptors. Because the brain is sterile, TLR activation in the brain in response to drugs of abuse likely involves DAMP-mediated signaling. One such DAMP that has been found to play a role in alcohol addiction in particular is the protein high-mobility group box 1 (or HMGB1), a nuclear chromatin binding protein that can be released during cellular stress, activation, or damage. Upon its release, HMGB1 acts as an immune mediator via TLR4 or RAGE receptors. HMGB1 has been implicated in alcohol addiction pathology and might be involved in other drugs of abuse (detailed further later in this chapter). HMGB1 is also released prior to hyperexcitable states, such as seizures, and modulates glutamatergic signaling. Neuroimmune activation and neuronal signaling could be interconnected by DAMP release. Several cytokines have been found to regulate normal brain function and could be dysregulated by drugs of abuse. Thus DAMP and cytokine paracrine and autocrine signaling across glia through kinase cascades may represent brain plasticity mechanisms that could contribute to the development of addiction
Immune Signaling Molecules as Neuromodulators
In the brain, the neuroimmune system functions not only to address foreign pathogens, but also regulates normal brain function. Recently, numerous immune signaling molecules have also been found to be important for synaptic activity, learning, and memory. For example, the classic proinflammatory cytokine TNFα is required for development of long-term potentiation (LTP) in the visual cortex and is critical for synaptic strength. At higher concentrations, however, TNFα disrupts LTP. This translates into behavioral alterations, as TNFα-overexpressing mice show decreased performance on spatial learning and memory tasks. The cytokine IL-1β also modulates LTP, promoting it at lower levels, but disrupting LTP at higher concentrations. In addition, IL-1β modulates neuronal GABA transmission in the central amygdala. The M2 (antiinflammatory) microglia-associated protein TGF-β1 promotes LTP and object recognition memory. IL-4 and IL-13 knockout (KO) mice show learning and memory impairments. The inflammatory chemokines MIP-1α and CX3CL1 also regulate synaptic plasticity and memory function. CX3CL1 KO mice show impaired LTP, whereas exogenously added MIP-1α impairs LTP. These immune-associated molecules have multiple functions. Thus in the brain, immune signaling molecules seem to function as endogenous neuromodulators. Disruption of the expression or release of these molecules by drugs of abuse could alter synaptic activity and behavior.
Addiction as a Neuroimmune Disease
Neuroimmune Components of the Stages of Addiction
The neuroimmune contribution to the pathology of addiction has become the topic of recent numerous reviews. b
b References 15, 49, 51, 52, 54, 56, 57, 86, 115, 123, 124, 126, 129, 156, 180.
Neuroimmune signaling has been identified in each of the stages of addiction. The pathology of addiction has been modeled by a three-stage process to identify different features that may be targeted by intervention. Addicted individuals cycle through binge intoxication, withdrawal and negative affect, craving, and preoccupation, and back to binge intoxication ( Fig. 20.1 ). Much work remains to be done to better understand the impact of innate immune activation during the various stages; however, several inflammatory mediators have been found to play important roles at various stages.
During the binge/intoxication stage, several immune-regulating interventions have been found to alter alcohol consumption. Immune activation by injection of the TLR4 agonist lipopolysaccharide (LPS) increases ethanol consumption in mice, whereas local knockdown of TLR4 in the central amygdala also decreases ethanol self-administration. MCP-1 increases alcohol self-administration after intracerebroventricular injection in rats. Meanwhile, the antiinflammatory mediators IL-1 receptor antagonist and IL-10 each reduce alcohol self-administration when injected into the basolateral amygdala. Blocking IL-1β signaling in the VTA also prevents cocaine-induced dopamine release in the nucleus accumbens. Furthermore, ablation of certain key neuroimmune genes, such as IL-6, IL-1ra, Ccr2, Ccl2, and Ccl3, decreases ethanol consumption. Compounds that inhibit microglia also reduce the binge/intoxication stage. Minocycline, a microglial inhibitor, reduces ethanol self-administration and conditioned place preference to cocaine. An analysis of genetically paired rodents found that high ethanol drinking animals show increased NF-κB and other proinflammatory gene expression. These studies suggest that neuroimmune activation may play a role in the rewarding properties of drugs of abuse. However, care should be taken when extrapolating rodent studies to humans, as in addicted humans, the rewarding properties of drugs of abuse shift from the drug itself to cues associated with the drug. The majority of rodent work to date has been done in nondependent animals and should be interpreted in that context.
Neuroimmune activation also occurs during the craving/preoccupation stage. In human alcoholics, increased plasma levels of multiple proinflammatory cytokines have been found such as TNFα (which correlates with severity of alcoholism), and IL-1β, IL-6, and IL-8, which correlate with alcohol craving. TNFα, IL-6, and IL-1β each cross the blood-brain barrier to exert their CNS effects. Infusion of the opioid-insensitive TLR4 antagonist (+)-naltrexone during withdrawal reduces cue-induced heroine seeking. More work needs to be done to discern the role of neuroimmune activation in the craving of drugs of abuse. However, these findings suggest a potential role and a possibility of immune therapeutics to prevent drug-associated craving.
Neuroimmune activation is also involved in the withdrawal/negative affect stage of addiction. A large body of literature does suggest that the development of negative affective states such as anxiety and dysphoria contribute to the maintenance of addiction. The negative affect stage is often described as stress. It is thought that continued substance abuse is an attempt to alleviate these negative states. Alcohol withdrawal causes increased inflammatory cytokines in the brain. In addition, intracerebroventricular injection of cytokines sensitizes anxiety-like behavior during alcohol withdrawal. Thus, cytokine induction is thought to contribute to negative affect associated with withdrawal. Heightened activation of stress pathways during withdrawal also cause neuroinflammation. Stress causes sensitization of microglia to inflammation in an HMGB1-dependent manner. TLR4 activation modulates serotonin transporter (SERT) function and increases depression-like behavior. Furthermore, various types of acute and chronic stress causes microglia activation in multiple brain regions and leads to depression-like behavior. The proinflammatory cytokines TNFα, IL-6, and IL-1β contribute to the pathologies of mood disorders. Agents that inhibit microglial activation block the development of depression-like behavior. Ethanol increases microglial markers such as CD11b and Iba1, consistent with microglial activation, and primes the microglial response to peripheral inflammation. In addition, stress also causes NF-κB activation. Human psychosocial stress causes NF-κB activation in blood monocytes. Restraint stress in rodents causes NF-κB activation with subsequent production of TNFα and proinflammatory prostaglandins. Ethanol activates NF-κB in rat and mouse brain, and human astrocytes. Therefore, it is clear that both stress and alcohol can affect glia and lead to neuroimmune activation. The exact neuroimmune milieu associated with drug withdrawal–induced stress is not clear. However, stress pathways overlap with neuroimmune pathways associated with drug withdrawal and are clearly activated. Further work needs to be done to determine the exact contributions of neuroimmune activation to negative affect and stress associated with drug withdrawal.
Neuroimmune Signaling in Alcoholism
Alcohol use activates the neuroimmune system, contributing to the development of alcohol use disorders. Findings from postmortem human alcoholic brain tissue, rodent studies, and cell culture work support this hypothesis. Postmortem human alcoholic brains show increased microglial and astrocyte markers. Postmortem human alcoholic brains show increased expression of neuroimmune molecules, such as MCP-1, TLR2, TLR3, TLR4, TLR7, and HMGB1. Ethanol exposure studies in vivo also find increased expression of TLRs2–4 in cortex and cerebellum, with subsequent NF-κB activation and cytokine induction. Binge alcohol treatment persistently increases neuroimmune molecules such as TLR3, TLR4, HMGB1, and RAGE. Ethanol also sensitizes neuroimmune responses to TLR3 and TLR4 agonists. Studies in vitro find that ethanol directly activates immune cells. Alcohol activates microglia in vitro, increasing expression of TNFα, IL-1β, iNOS, and NADPH oxidase. In addition, alcohol activates astrocytes, with increases in GFAP. These effects in vitro and in vivo involve TLR activation. TLR4 activation has been shown to play a pivotal role in alcohol-induced neuroimmune signaling. TLR4 KOs and TLR4 KO glia are protected from much of neuroimmune activation by ethanol including glial cell activation, NF-κB activation, caspase-3 activation, anxiety-like behavior, and memory impairment. c Astrocytes treated in vitro with siRNA against TLR4 or MD-2 and CD14 (critical adaptor molecules for TLR4 signaling) are protected from ethanol-induced NF-κB induction. In addition, ethanol increases the formation of TLR4/TLR2 heterodimers in microglia cell membrane, causing iNOS induction and MAPK activation. Both TLR4 and TLR2 KOs were protected. In an alcohol-preferring rat strain (p-rats), increased expression of TLR4 in the VTA was found to correspond with binge ethanol responding, with TLR4 expression being regulated by GABA(A)α2 receptor and the stress-associated corticotropin-releasing factor (CRF). TLR4 activation has also been implicated in cocaine and heroin abuse. Furthermore, ethanol-induced innate immune activation caused impairment of short and long-term memory for object recognition in mice. This was accompanied by a reduction of H3 and H4 histone acetylation as well as histone acetyltransferase activity in the frontal cortex, striatum, and hippocampus. TLR4 KO mice were protected from behavioral impairments and histone modifications. TLR7 has also been identified as important in neuroimmune activation by ethanol leading to hippocampal neurodegeneration, and should be investigated further. Activation of TLRs is a central feature in the neuroimmune responses to ethanol. This leads to NF-κB activation and amplification of immune responses. Ethanol causes NFκB activation in neurons in brain slice culture and in vivo. Although the unique cellular activation patterns need to be further elucidated, it is clear that ethanol activates NFκB in brain. Ethanol increases NFκB-DNA binding both in vivo in mice and in vitro in rat hippocampal-entorhinal cortex slice culture. Ethanol also induces NFκB target genes, such as MCP-1, proinflammatory cytokines (TNFα, IL-1β, and IL-6), proinflammatory oxidases (iNOS, COX, and NOX ), and proteases (TNFα-converting enzyme [TACE] and tissue plasminogen activator [tPA]). Cocaine also causes NF-κB activation in vivo that is required for increased conditioned place preference. The exact mechanism of TLR activation by alcohol and other drugs of abuse is not clear. However, the involvement of multiple TLRs as well as the coordinate induction of endogenous TLR-activating DAMPs suggests a DAMP-mediated phenomenon.
The Role of Endogenous TLR Agonist DAMPs in the Pathology of Addiction
The key role of TLR signaling in the neuroimmune pathology of addiction begs the question of—What is the mechanism of TLR activation? The brain is typically a sterile environment; however, TLRs are expressed and follow differential developmental expression patterns. Certain TLRs have also been found to have nonimmune functions, such as TLR3 and TLR8, which regulate axonal and neurite outgrowth, respectively. Thus TLR signaling occurs in brain function in the absence of infection, through the release of endogenous TLR agonists. This led to the hypothesis that TLR activation in addiction is mediated through the release of endogenous agonists. Indeed, induction of DAMPs has been found in the pathology of alcoholism. The endogenous TLR4 and RAGE agonist, HMGB1, in particular, has been identified as a critical neuroimmune mediator in alcoholism. HMGB1 is increased in postmortem human alcoholic brain in several brain regions and correlates with lifetime alcohol consumption. Ethanol administration in vivo increases HMGB1 in cortex and cerebellum. In addition, microglia secrete HMGB1 in response to ethanol as well as tobacco smoke extract. Thus ethanol and other drugs of abuse can cause sterile inflammation by the release of HMGB1. Recently, ethanol has been found to cause TLR7 activation through the vesicular secretion of the miRNA let-7b DAMP. Let-7b binds the single-stranded RNA sensing TLR7 leading to neuroimmune activation and neurodegeneration. HMGB1 is actually required for immune responses to TLRs 3, 7, and 9. Therefore, HMGB1 may represent a critical modulator of multiple neuroimmune signals. Indeed, blocking HMGB1 release prevents cytokine induction by chronic ethanol. Methamphetamine also induces HMGB1 in vivo and in vitro , with HMGB1 inhibition preventing neuroimmune activation by methamphetamine.
Neuroimmune Activation and the Progression to Addiction
The progression from drug or alcohol abuse to addiction occurs over time ( Fig. 20.2 ). This progression often begins during adolescence. Alcohol and drug abuse during adolescence are common. Regarding alcoholism, the age at drinking onset is strongly related to the risk of developing an alcohol use disorder in adulthood. Adolescence is a unique developmental period during which maturation of key structures that regulate cognitive function and decision-making occurs (for review see Crews et al. ). Binge drinking during this time has long-lasting effects including persistent neuroimmune activation, leading to prolonged cognitive dysfunction. Indeed, neuroimmune markers in alcoholics correlate with lifetime alcohol consumption and age at drinking onset, with some remaining elevated long after ethanol administration, during abstinent periods. It is well known that repeated cycles of binge exposure and withdrawal amplify alcohol-induced pathology. This is similar to the innate imune system, as autocrine and paracrine signaling by the release of DAMPS, such as HMGB1, amplify immune responses with each recurring exposure. This amplification might be similar to an LTP-type of phenomenon, and could represent neuroimmune plasticity. This is interesting, especially given the modulation of LTP systems in the brain by many proinflammatory cytokines. The recurrent and persistent NF-κB activation by repeated alcohol or drug intake might mimic a chronic inflammatory state. This is supported by the increased innate immune markers in the postmortem brains of human alcoholics, as well as the upregulation of NF-κB target genes. This persistent and chronic immune response has also been seen after one dose of systemic LPS, a TLR4 agonist. One administration of high-dose LPS (5 mg/kg) causes persistent microglial activation with ongoing ROS generation leading to dopaminergic neuron loss over a period of months. Although seemingly on a lower scale, binge-ethanol causes similar pathophysiology. The recurrent and prolonged amplification of neuroimmune responses causes TLR and NF-κB activation, leading to further increased TLR expression, cytokine production, and DAMP release. This involves the release of HMGB1 as well as miRNA-let-7b -containing microvesicles. Let-7 isoforms are increased in postmortem human alcoholic brain and in rodent chronic ethanol models. Furthermore, ethanol induces ROS generation that also activates NF-κB and leads to further cytokine release and paracrine signaling. Each progressive binge may increase the neuroimmune baseline, leading to further damage and pathology. This “smoldering” level of inflammation due to repeated drug binges, as is typical with alcoholism and drug abuse, might underpin the transition from abuse to addiction. Intervention of this feature of disease pathology might belay this transition.

Another important cognitive feature in the progression to addiction is a loss in frontal cortical mediated executive function. These functions include motivation, planning and goal setting, and behavioral flexibility. Frontal cortical dysfunction results in perseveration and repetition of previously learned behaviors due to failure to associate new information (e.g., negative consequences) into decision-making. Binge drinkers report more negative mood and perform worse on executive functioning tasks. In alcoholics this is common and manifests in impulsivity and behavioral inflexibility. Reversal learning—the ability to change previously learned behaviors—is an index of behavioral flexibility. Both human alcoholics and cocaine addicts demonstrate reversal learning deficits. Rodents also show persistent deficits in reversal learning following binge ethanol or cocaine. Frontal cortical function is key in this circuitry that involves loops between the prefrontal cortex, striatum, and amygdala. Frontal cortical regulation of mood and cognition occurs through reciprocal glutamatergic connections with multiple brain regions. A hyperglutamatergic state has also been demonstrated in both cocaine- and stimulant-addicted brains. Astrocytes are key regulators of synaptic glutamate levels. Ethanol exposure induces NF-κB activation in astrocytes, leading to increased expression of proinflammatory genes and impaired astrocyte glutamate transport. Increased extracellular glutamate levels cause enhanced neuronal excitation, microglial activation, and excitotoxicity. TLR4 KOs are protected from this ethanol induced-hyperglutamatergic state and its associated neurotoxicity. Innate immune gene induction in the frontal cortex contributes to glutamatergic hyperexcitability and the impairment of executive function. Thus, neuroimmune activation by ethanol may be a component of the progressive development of hyperexcitability and cognitive dysfunction seen in alcohol use disorders.
Neuroimmune Basis of Addiction—A Work in Progress
We have presented much of the work that shows that neuroimmune activation is a key feature in the pathology of addiction. However, much work remains to be done. Little research has been done regarding brain regional differences in neuroimmune responses to drugs of abuse. Each brain region has specific populations of neurons that may respond differently to alcohol and other drugs of abuse. Furthermore, the complex interactions between cell types—neurons, microglia, and astrocytes—need to be further elucidated. In Fig. 20.3 , we summarize some of the cell-cell interactions between neurons and glia regarding HMGB1, let-7b, and TLRs 4 and 7. However, there remains much to be discovered regarding these interactions. In addition, the interaction between stress, psychiatric disorders, and drugs of abuse on the neuroimmune system is also likely very important in human populations. Stress is a key feature in the cycle of addiction; however, addiction-related stress needs to be better differentiated from other non–addiction-related stressors. A hypothesis is presented in this chapter of stress and drug-induced neuroimmune signaling that inactivates frontal cortex and sensitizes limbic circuitry. This leads to persistent increases in TLRs and DAMPs (e.g., HMGB1) amplified by cycles of stress and intoxication. Innate immune signaling impacts cognitive and emotive function, leading to dysfunction. These mechanisms might underlie the progressive and persistent nature of addiction. Currently, neuroimmune mechanisms have been most studied in the context of alcohol abuse and alcoholism. Considerable emerging evidence supports a role for HMGB1/TLR signaling, innate immune gene induction, and alterations in epigenetics and neurotransmission as culminating in the neurobiology of alcoholism. Innate immune activation and TLR signaling seem to be essential for ethanol induction of pathology. Ethanol and other drugs of abuse promote innate immune gene induction. which seems to be linked to changes in executive function, reinforcement-reward, and negative affect-craving-anxiety to promote addiction. Although much of this work is convincing regarding the presence of a role of neuroimmune activation involvement in the pathology of addiction, there remain many gaps regarding the precise mechanism and the significance of immune activation. Nonetheless, significant findings exist to warrant the investigation of neuroimmune therapies for efficacy in the treatment or prevention of addiction.

Future Immune Therapies for Addiction
Toward Novel Addiction Treatment Strategies Based on Immune Pharmacology
Given the data presented, several potential neuroimmune therapies are given in Table 20.2 . Some of these medications are currently being tested in clinical trials for alcohol use disorders. Due to the challenges to the human condition associated with translating animal models of alcohol and drug abuse, and gaps in knowledge, it is unclear whether these therapies would be of highest benefit for prevention or recovery from addiction. It is known that neuroimmune activation and addiction can cause neurodegeneration. Although immune therapies would likely not be of benefit in brain regions where neurodegeneration has already occurred, there may still be a benefit for recovery of normal synaptic function and neuroplasticity. Microglia and cytokines can alter synaptic signaling. For example, IL-1β reduces eIPSCs in the central amygdala to modulate ethanol effects on GABA receptors. Therefore, significant exploration of immune therapies in various models of alcohol abuse is warranted. Many US Food and Drug Administration (FDA)–approved drugs have also been found to have antiinflammatory actions in the CNS. Table 20.2 lists some candidate drugs that have been found to be beneficial in the context of addiction or other neuroimmune conditions. For example, minocycline is a tetracycline antibiotic and microglial inhibitor that prevents ethanol induced–microglial activation and reduces alcohol self-administration. A recent human study found that in a laboratory setting minocyline did not alter subjective responses to ethanol or ethanol-induced craving; however, alcohol self-administration has not yet been reported in humans. Phosphodiesterase 4 (PDE4) inhibitors reduce inflammation by increasing cAMP concentration, resulting in reduced NF-κB activation. Recently, these agents have been found to reduce ethanol intake in rodents. A recent phase 2 clinical trial using the PDE4 inhibitor ibudilast showed beneficial effects, including improvements in mood after stress and alcohol-cue exposures, and reduced levels of tonic craving. A different placebo-controlled trial found that ibudilast reduced some of the rewarding effects of methamphetamine. PPARγ agonists might also be helpful in alcohol use disorders through their antiinflammatory activities. The PPARγ agonist pioglitazone is a microglial inhibitor that reduces neurotoxicity in fetal alcohol spectrum disorder models that could potentially play a beneficial role in alcoholism. Each of these drugs, and others with antiinflammatory actions, should be investigated for their potential efficacy in alcohol use disorders.
Drug | Mechanism Primary Immune | CNS Activity |
---|---|---|
Minocycline | Tetracycline antibiotic Microglial inhibitor | Reduces alcohol self-administration Reduces ethanol microglia activation Prevents reinstatement of morphine and amphetamine seeking |
Rapamycin | Macrolide antibiotic mTORC1 inhibitor | Reduces binge ethanol intake in male patients Neuroprotection via autophagy promotion |
Azithromycin | Macrolide antibiotic Microglial inhibitor | Promotes antiinflammatory M2 microglial activation state |
Rifampin | Bacterial RNA polymerase inhibitor TLR4 inhibition | Inhibits microglia activation to TLR4 , |
Indomethacin | COX-2 inhibitor | Reduces alcohol self-administration Reduces ethanol neurotoxicity |
Simvastatin | HMG-CoA Reductase inhibitor NF-κB inhibition | Reduces inflammation and neurotoxicity to ischemia and injury |
Glycyrrhizin | HMGB1 inhibition | Blocks ethanol-induced cytokine release Reduces neuroinflammation after traumatic brain injury |
Pioglitazone, DHA | PPARγ agonists | Reduce toxicity and proinflammatory cytokines in fetal alcohol spectrum disorder model |
Ibudilast, mesopram, rolipram, CDP 840 | Phosphodiesterase 4 inhibition | Reduce ethanol intake in C57BL/6J mice Reduces ethanol self-administration in rats |
Naltrexone/naloxone and nalmefene | μ-Opioid antagonists TLR4 inhibition | Reduces alcohol self-administration Binds TLR4 adaptor protein MD2 , Prevent neuroimmune activation by ethanol |
Etanercept | TNFα antagonist | Prevents REM sleep disruption in alcoholics |
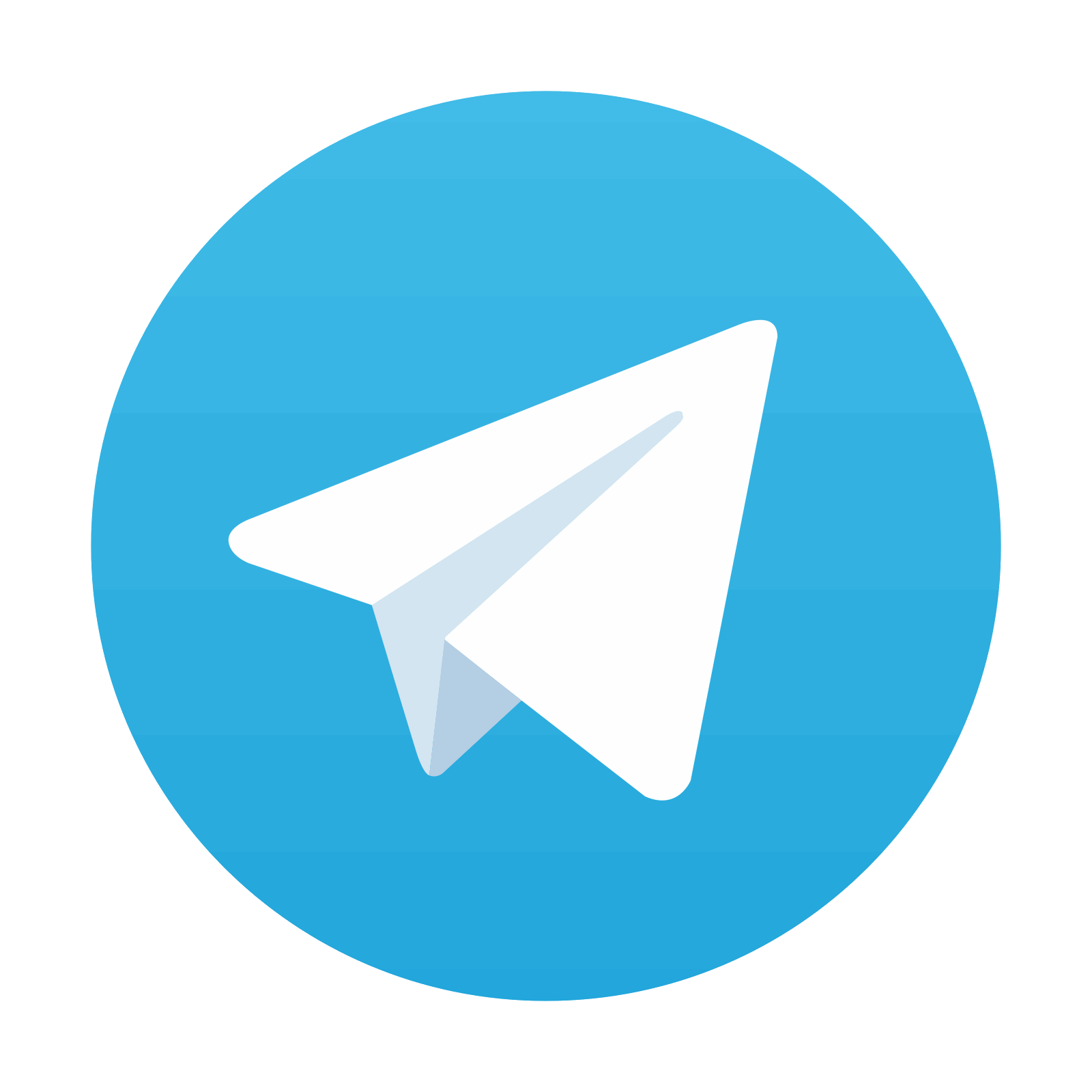
Stay updated, free articles. Join our Telegram channel

Full access? Get Clinical Tree
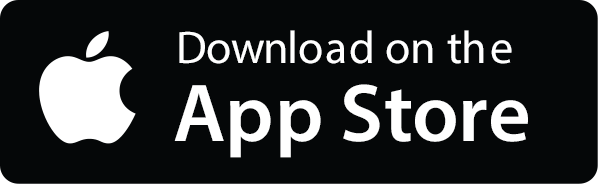
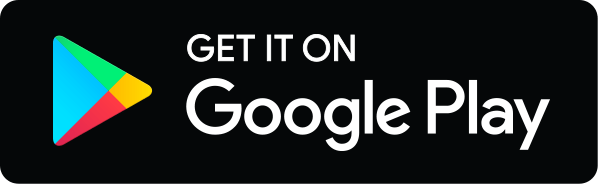
