Musculoskeletal System Disorders
Learning Objectives
After studying this chapter, the student is expected to:
1. Describe the general structure and function of bone and joints.
2. Describe the general structure and function of skeletal muscle.
3. Describe the types of fractures, the healing process in bone, and potential complications.
4. Compare dislocations, sprains, and strains.
5. Describe the pathophysiology of osteoporosis, the predisposing factors, and possible complications.
6. Compare the causes and effects of rickets, osteomalacia, and Paget’s disease.
7. Describe the common bone tumors.
8. Describe the characteristics of Duchenne’s muscular dystrophy.
9. Describe the effects of fibromyalgia.
11. Describe the distinguishing features of infectious (septic) arthritis.
Key Terms
anabolic steroids
ankylosis
arthroscopy
articulation
crepitus
diaphysis
electromyograms
endosteum
epiphysis
fascia
hyperuricemia
kyphosis
lordosis
medullary cavity
metaphysis
motor unit
neuromuscular junction
osteoblasts
osteoclast
osteocytes
periosteum
pseudohypertrophic
scoliosis
uveitis
Review of the Musculoskeletal System
Bone
The skeletal system provides rigid support for the body, particularly when it is in an upright position or is in motion. The skeletal framework determines the basic size and proportions of the body. Protection is provided for the viscera, such as the heart and lungs, and for fragile structures such as the spinal cord and brain. Bone also has important metabolic functions related to calcium metabolism and storage and the bone marrow, which serves as the area where new blood cells are produced by a process called hematopoiesis.
Bones may be classified by shape:
• Long bones, such as the humerus and femur, consist of a long, hollow shaft with two bulbous ends.
• Short bones are generally square-like in shape and are found in the wrist and ankle.
• Flat bones occur in the skull and are relatively thin and often curved.
Individual bones have unique markings, which may be lines, ridges, processes, or holes. Such landmarks provide for attachment of tendons or passage of nerves and blood vessels.
Bone is special connective tissue consisting of an intercellular matrix and bone cells. The matrix is organized in microscopic structural units called Haversian systems or osteons, in which rings of matrix (lamellae) surround a Haversian canal containing blood vessels (Fig. 9-1). The matrix is composed of collagen fibers and calcium phosphate salts (e.g., hydroxyapatite crystals), which provide a very strong and rigid structure. Mature bone cells, or osteocytes, lie between the rings of matrix in spaces called lacunae. Small passages termed canaliculi provide communication between the Haversian canals and the lacunae.
• A dynamic equilibrium is maintained between new bone, which is constantly being produced by osteoblasts, and the resorption of bone by osteoclast activity, in accordance with the various hormonal levels and the degree of stress imposed on the bone substance. Osteoblast and osteoclast activity provide the homeostasis of bone. Osteoprogenitor cells differentiate into osteoblasts.
• Osteoprogenitor cells are derived from embryonic mesenchymal cells.
• Osteoblasts are responsible for secreting the matrix of bone.
• Osteoclasts are derivatives of macrophage progenitor cells.
• Osteoblast and osteoclast activity depend on two hormones: calcitonin and parathyroid hormone.
• Calcitonin stimulates osteoblasts.
Bone tissue consists of two types, which differ in density. Compact bone is formed when many Haversian systems are tightly packed together, producing a very strong, rigid structure that forms the outer covering of bones. Cancellous or spongy bone is less dense and forms the interior structure of bones. Spongy bone lacks Haversian systems but is made up of plates of bone bordering cavities that contain marrow.
A typical long bone consists of the diaphysis, a thin shaft, between two larger ends or epiphyses (see Fig. 9-1B). The diaphysis is formed of compact bone surrounding a medullary cavity containing marrow. The metaphysis is the area where the shaft broadens into the epiphysis. The epiphysis is made up of spongy bone covered by compact bone. The end of each epiphysis is covered by hyaline cartilage (articular cartilage), which facilitates movement at points of articulation between bones.
The epiphyseal cartilage or plate (“growth” plate) is the site of longitudinal bone growth in children and adolescents, such growth being promoted by growth hormone and sex hormones. Longitudinal bone growth ceases when the epiphyseal plate ossifies during adolescence or early adulthood depending on the specific bone. The epiphyseal plate is referred to as the epiphyseal line following ossification or closure. No bone growth in length occurs after the epiphyseal plate becomes the epiphyseal line.
However, bone may change in density or thickness at any time under the influence of hormones such as growth hormone, parathyroid hormone, or cortisol. The stress (weight-bearing or muscle tension) placed on the bone also affects the balance between osteoblastic and osteoclastic activity. With aging, bone loss is accentuated, resulting in decreased bone mass and density. Osteoporosis, loss of bone density due to loss of calcium salts, is common in older people, particularly women (see Chapter 24). Except for the surface of the bone covered by articular cartilage, the bone is covered by periosteum, a fibrous connective tissue. The periosteum contains osteoblasts, blood vessels, nerves, and lymphatics, some of which penetrate into the canals in the bone. When the periosteum is stretched or torn, severe pain results.
The medullary cavity is lined with endosteum, also containing osteoblasts. These osteoblasts are required for bone repair and remodeling as needed. At birth the medullary cavity in most bones contains red bone marrow in which hematopoiesis takes place. Gradually, yellow (fatty) bone marrow replaces red bone marrow in the long bones. In adults, red bone marrow is found in the cranium, bodies of the vertebrae, ribs, sternum, and ilia, the last two being the usual sites of bone marrow aspiration used in the diagnosis and monitoring of leukemias and blood dyscrasias.
Skeletal Muscle
Skeletal muscle has four basic functions:
1. To facilitate body movement by muscle contraction
2. To maintain body position by continuing muscle tone
3. To stabilize the joints and prevent excessive movement
4. To maintain body temperature by producing heat through muscle contraction
Skeletal muscle is considered to be under voluntary control, although some muscle activities occur without deliberate intent, such as respiratory movements, postural reflexes, blinking, shivering, or certain facial expressions.
Skeletal is striated muscle that consists of bundles of muscle fibers (cells) covered by connective tissue. The striated or striped appearance results from the arrangement of the actin and myosin filaments within the muscle fibers.
Connective tissue coverings of skeletal muscles are:
• Epimysium—surrounding the entire muscle
• Perimysium—surrounding the fascicles
• Endomysium—surrounding the individual muscles fibers (cells)
Muscle tissue is well supplied with nerves and blood vessels, necessary to fulfill its function. Each muscle fiber is an elongated muscle multinucleated cell containing many mitochondria that supply energy for the contraction process. A muscle is stimulated to contract when an efferent impulse is conducted along a motor neuron to a muscle. The axon of the motor nerve branches as it penetrates a muscle so that each muscle fiber in the muscle receives a stimulus to contract at the same time. The motor neuron of the spinal cord and all the muscle fibers it stimulates are referred to as the motor unit. At the neuromuscular junction, where the synapse between the end of the motor nerve and the receptor site in the muscle fiber is located, the chemical transmitter acetylcholine is released (Fig. 9-2). Following its release and the subsequent muscle contraction, acetylcholine is inactivated by the enzyme acetylcholinesterase (AChE). Skeletal muscle relaxing drugs may act by blocking acetylcholine at the muscle receptor sites, whereas muscle activity may be promoted by drugs that interfere with cholinesterase activity.
Each muscle cell contains myofibrils, which in turn are made up of smaller myofilaments consisting of the proteins actin and myosin. Actin and myosin filaments are the contractile elements of the muscle fiber.
The mechanism of muscle contraction starts at the neuromuscular junction and ends with the actual contraction of the skeletal muscle fibers.
• An action potential from the motor neuron arrives at the presynaptic terminal.
• The arrival of the action potential results in the depolarization of the presynaptic terminal.
• The depolarization is followed by a calcium influx into the presynaptic terminal.
• The calcium influx results in the exocytosis of the neurotransmitter (Ach) into the synaptic cleft.
• Diffusion of the neurotransmitter to the postsynaptic receptor results in a muscle action potential.
• The muscle action potential travels down the t-tubules to cause a second messenger activation.
• During muscle relaxation calcium is transported back into the sarcoplasmic reticulum.
• Both muscle contraction and relaxation require cellular energy (adenosine triphosphate, ATP).
During exercise, the blood vessels in the muscles are dilated to promote greater blood flow into the muscle, thus increasing the supply of oxygen and nutrients (glucose and fatty acids) to provide energy for the contraction and remove metabolic wastes. Limited amounts of oxygen can be bound to myoglobin and stored in muscle fibers. Myoglobin is a red oxygen-binding protein, similar in structure to hemoglobin, which is present in muscle cells. Glycogen, a source of glucose, is also stored in muscle.
Aerobic respiration to produce ATP can be maintained in muscle fibers as long as adequate oxygen is made available from the myoglobin and the circulating blood. If the supply of oxygen does not meet the demand, the process of anaerobic respiration begins, using glucose as the primary energy source and incurring an oxygen debt (the amount of oxygen required to restore the muscle cell to its normal resting state, including converting lactic acid to pyruvic acid, glucose, or glycogen and replenishing stores of ATP). Anaerobic respiration produces lactic acid rather than carbon dioxide, and smaller amounts of ATP. This state of acidosis leads to the increased respirations commonly observed during exercise. These respirations operate as a compensatory mechanism to reduce acidosis by decreasing carbon dioxide levels in the blood (see Chapter 2).
The accumulated lactic acid may cause local muscle pain and cramping during and immediately after exercise. A muscle cramp is pain resulting from a strong muscle contraction or spasm, usually caused by local irritation from metabolic wastes. Muscle spasm reduces blood flow, thus leading to ischemic pain. Muscle soreness and pain that appear a day or so after strenuous exercise are often due to minor damage to muscle cells and subsequent inflammation. Also, during periods of strenuous physical activity and anaerobic metabolism, excessive lactic acid diffuses into the blood, lowering serum pH and causing metabolic acidosis.
A muscle may be attached directly to the periosteum of a bone, but more often the connective tissue covering the muscle (perimysium) extends to form a cordlike structure or tendon, which attaches each end of the muscle to the two bones that articulate at a joint. At a joint, one bone remains fixed, forming the origin of the muscle. The other bone attached to the same muscle is moved by the muscle contraction and is called the insertion. Ligaments form a direct attachment between two bones. Tendons and ligaments are composed of collagen fibers arranged in bundles, a structure that can withstand considerable stress. At the insertion point of tendons or ligaments there is a gradual transition from the connective tissue to the bone or cartilage. Tendons and ligaments have little blood supply; therefore healing of these structures is difficult and slow.
Muscles may work singly or in groups to perform a specific movement. Also, muscles at a site may be designated as antagonists because one muscle opposes the action of another, allowing movement in either direction. For example, at the elbow, the triceps brachii muscle functions as an extensor muscle, whereas the biceps brachii is a flexor muscle. Antagonistic muscles prevent excessive movement and provide better control of movements.
Skeletal muscle cells do not undergo mitosis; therefore that process cannot be used to enhance muscle activity or replace damaged muscle. However, muscle cells may undergo hypertrophy (increased size of the muscle cell) when the demands are increased, such as with regular exercise. Aerobic or endurance exercise, such as swimming or running, increases the muscle’s capacity to work for a longer time without causing marked hypertrophy of the muscle. Such exercise increases the capillaries and blood flow in a muscle as well as the mitochondria and myoglobin content, thus improving efficiency and endurance. This type of exercise also promotes general respiratory and cardiovascular function. Anaerobic or resistance exercise, such as weight lifting or bodybuilding, focuses on increasing muscle strength by increasing muscle mass (hypertrophy). It is helpful for those persons interested in developing strong muscles to incorporate some aerobic exercise into the training program to improve cardiopulmonary fitness as well as strength.
Anabolic steroids are synthetic hormones similar to testosterone, the male sex hormone. They are used by some athletes, bodybuilders, and others interested in changing the body image to build up muscle strength and mass. Speed and endurance do not appear to be affected. These synthetic hormones (e.g., methenolone [Primobolan]) have been developed to increase the anabolic effects, or protein synthesis, and decrease the androgenic or male characteristics produced by these chemicals. Serious and sometimes life-threatening side effects are associated with the use of these substances, such as liver damage, cardiovascular disease, personality changes, emotional lability, and sterility. Unfortunately, this type of steroid is abused by many adolescents and young adults, including those involved in sports, those with eating disorders, and those with psychological problems related to body image and poor self-esteem. The use of anabolic steroids by participants in athletic competition has been banned by many organizations.
Skeletal muscle also may atrophy, in which muscle cell size is decreased, when the muscle is not used (see Chapter 1). Atrophied muscle becomes weak and flaccid. Atrophy may occur within a short period of time when a fractured limb is placed in a cast or the pain of arthritis limits movement. Such disuse atrophy is also associated with immobilization and chronic illness (see Chapter 25). Atrophy may be secondary to nerve injury, with resultant flaccid paralysis. Also, nutritional deficiencies, particularly protein, secondary to disorders such as anorexia or Crohn’s disease, lead to atrophy. Skeletal muscle may also become weak owing to degenerative changes involving accumulations of fatty or fibrous tissue. With aging, muscle mass decreases owing to both a decrease in number of muscle cells and a decrease in size (diameter) of the fibers. Muscle strength generally diminishes as well, although this may vary with the individual’s degree of activity and general health status.
Muscle twitch or tetany usually results from increased irritability of the motor nerves supplying the muscle. For example, hypocalcemia causes increased permeability of the nerve membrane and therefore increased or spontaneous stimulation of the skeletal muscle fibers, causing a contraction or spasm of the muscle. Note that sufficient calcium is stored and returned to storage in the skeletal muscle cell following contraction, and therefore hypocalcemia does not directly affect skeletal muscle function, but rather its innervation.
Joints
Joints or articulations between bones vary in the degree of movement allowed:
Different types of diarthroses allow a variety of movements. For example, a hinge joint, providing flexion and extension, is found at the elbow, whereas a ball-and-socket joint at the shoulder provides a wide range of motion, including rotation. Both hinge and gliding movements are found in the temporomandibular joint (TMJ), controlling the opening of the mouth. Common body movements are illustrated in Ready Reference 1 (see Fig. RR 1-6).
In a synovial joint, the ends of the bone are covered with articular (hyaline) cartilage, providing a smooth surface and a slight cushion during movement (see Fig. 9-13A). With aging, the cartilage in joints tends to degenerate and become thin, leading to difficulty with movement and potential changes in the alignment of bones.
The joint cavity or space between the articulating ends of the bones is filled with a small amount of synovial fluid, which facilitates movement. The synovial fluid prevents the articular cartilage on the two surfaces from damaging each other and also provides nutrients to the articular cartilage. The synovial fluid is produced by the synovial membrane (synovium), which lines the joint capsule to the edge of the articular cartilages. The synovial membrane is well supplied with blood vessels.
The articular capsule is composed of the synovial membrane and its outer covering, the fibrous capsule, a tough protective material that extends into the periosteum of each articulating bone (Sharpey’s fibers). The capsule is reinforced by ligaments, straps across the joint that link the two bones, which support the joint and prevent excessive movement of the bones.
In a few joints there are some variations in structure. The knee has additional moon-shaped fibrocartilage pads, termed lateral and medial menisci, which act to stabilize the joint. Bursae are fluid-filled sacs composed of synovial membrane and located between structures such as tendons and ligaments; they act as additional cushions in the joint. The TMJ, the only movable joint in the skull and face, has two synovial cavities and a central articular cartilage of dense collagen tissue.
The nerves supplying a joint are those supplying the muscles controlling the joint. These motor fibers are accompanied by sensory fibers from proprioceptors in the tendons and ligaments that respond to the changing tensions related to movement and posture. The joint capsule and ligaments are supplied with pain receptors.
Diagnostic Tests
In persons in whom trauma, tumors, or metabolic disease are suspected, bone abnormalities may be evaluated using x-rays (radiographs) and bone scans.
Electromyograms (EMGs) measure the electrical charge associated with muscle contraction and are helpful in differentiating muscle disorders from neurologic disease. Also, the strength of individual muscle groups can be determined. Muscle biopsy is required to confirm the presence of some muscular disorders, such as muscular dystrophy. Joints may be visualized by arthroscopy (insertion of a lens directly into the joint) or by magnetic resonance imaging (MRI), a noninvasive imaging procedure. Synovial fluid may be aspirated and analyzed to ascertain whether inflammation, bleeding, or infection is present.
Serum calcium, phosphate, and parathyroid hormone levels may indicate metabolic changes, perhaps secondary to renal disease or parathyroid hormone imbalance. Muscle disorders may be checked by determining levels of components such as serum creatine kinase (CK), which is elevated in persons with many muscle diseases. Creatine kinase, an enzyme with an essential role in energy storage, leaks out of damaged muscle cells into body fluids.
Trauma
Fractures
A fracture is a break in the rigid structure and continuity of a bone (Fig. 9-3). Fractures can be classified in several ways:
• Open-closed. An open or compound fracture results when the skin is broken (Fig. 9-4). The bone fragments may be angled and protrude through the skin. In open fractures there is more damage to soft tissue, including the blood vessels and nerves, and there is also a much higher risk of infection. In a closed fracture the skin is not broken at the fracture site.
• Comminuted fracture, in which there are multiple fracture lines and bone fragments
• Depressed fracture occurs in the skull when the broken section is forced inward on the brain.
• Direction of the fracture line; for example:
• A fracture across the bone is a transverse fracture.
• A break along the axis of the bone is a linear fracture.
• A break at an angle to the diaphysis of the bone is an oblique fracture.
• A break that angles around the bone, usually due to a twisting injury, is a spiral fracture.
• Unique names for certain types of fractures; for example:
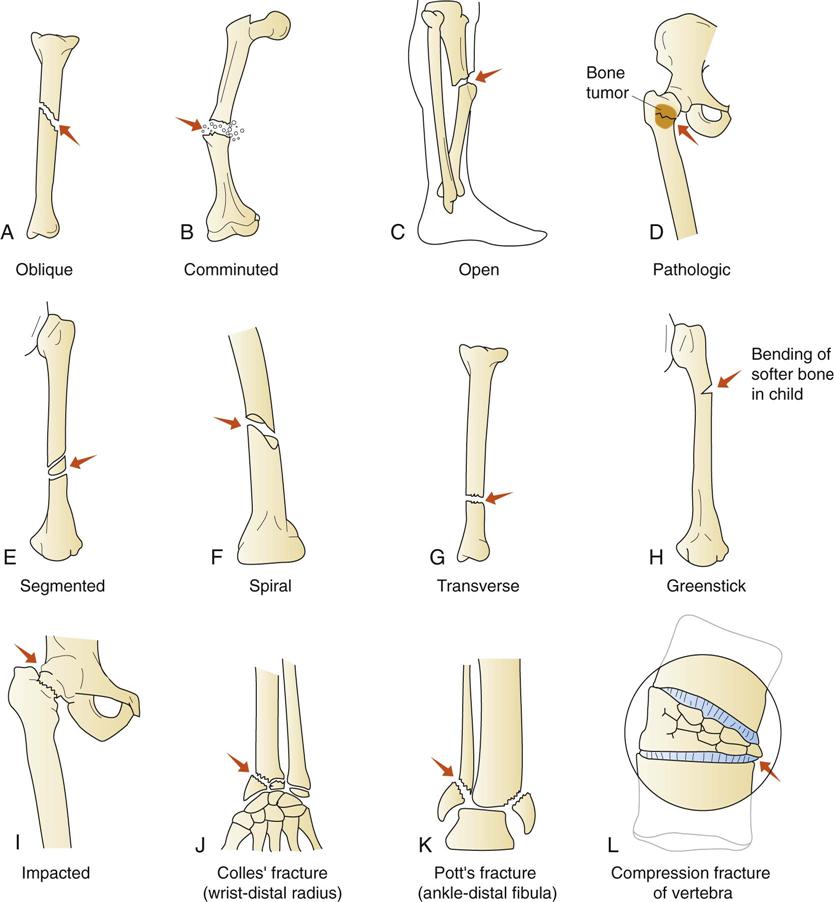
Pathophysiology
When a bone breaks, bleeding occurs from the blood vessels in the bone and periosteum. Bleeding and inflammation also develop around the bone because of soft tissue damage. This hematoma or clot forms in the medullary canal, under the periosteum, and between the ends of the bone fragments (Fig. 9-5). Necrosis occurs at the ends of the broken bone because the torn blood vessels are unable to continue delivery of nutrients. An inflammatory response develops as a reaction to the trauma and the presence of debris at the site.
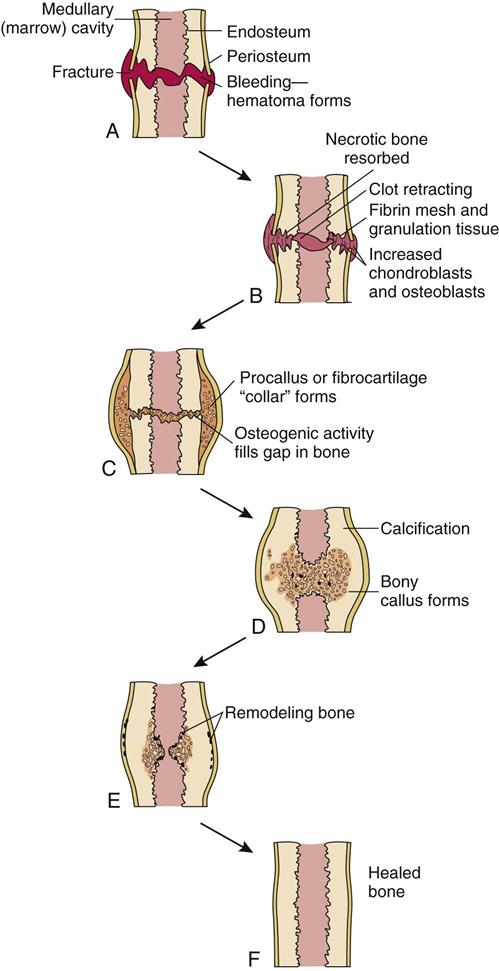
At fracture sites, the hematoma serves as the basis for a fibrin network into which granulation tissue grows. Many new capillaries extend into this tissue, and phagocytic cells (for removing debris) and fibroblasts (for laying down new collagen fibers) migrate to it. Also, chondroblasts begin to form cartilage. Thus, the two bone ends become splinted together by a procallus or fibrocartilaginous callus (collar). This structure is not strong enough to bear weight, but constitutes the preliminary bridge repair in the bone.
Osteoblasts from the periosteum and endosteum begin to generate new bone to fill in the gap. Gradually the fibrocartilaginous callus is replaced by bone through extensive osteogenic activity, which forms a bony callus. Note that damaged bone is repaired by new bone formation, not by scar tissue. During subsequent months the repaired bone is remodeled by osteoblastic and osteoclastic activity in response to mechanical stresses on the bone. The excessive bone in the callus is removed, more compact bone is laid down, and eventually the bone assumes a normal appearance.
To summarize, the five stages of bone healing are hematoma, granulation tissue, procallus (fibrocartilage), bony callus, and remodeling.
Factors Affecting the Healing of Bone
Many factors affect the healing process in bone. In children fractures usually heal in approximately 1 month; in adults the process requires 2 or more months. A fracture in an elderly person may require many months.
• Any secondary problem such as foreign material or infection at the site delays healing.
• Numerous systemic factors also affect the healing process in bone. For example, fracture repair is delayed in older persons and individuals with circulatory problems, anemias, diabetes mellitus, or nutritional deficits as well as in those taking drugs such as glucocorticoids (see Chapter 5).
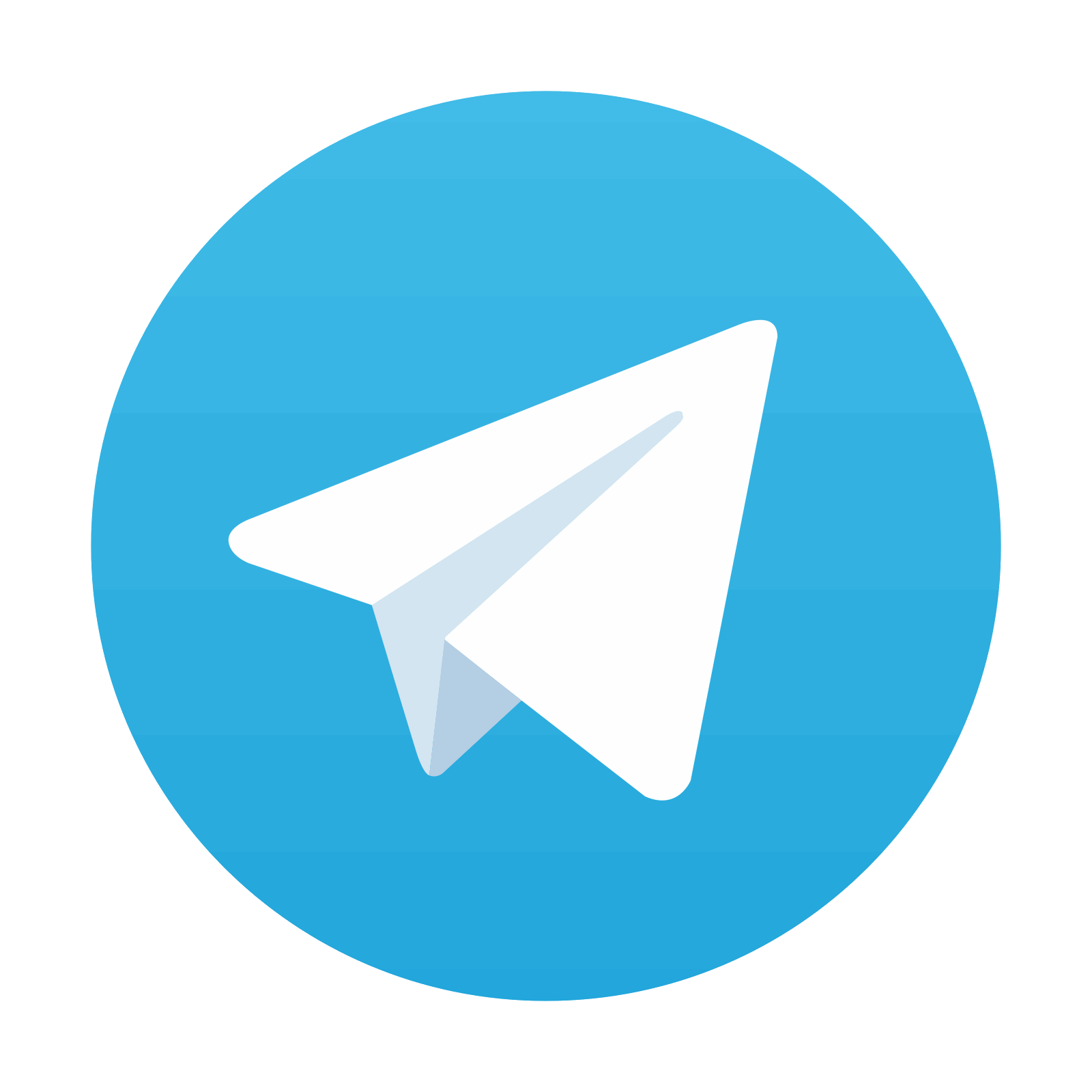
Stay updated, free articles. Join our Telegram channel

Full access? Get Clinical Tree
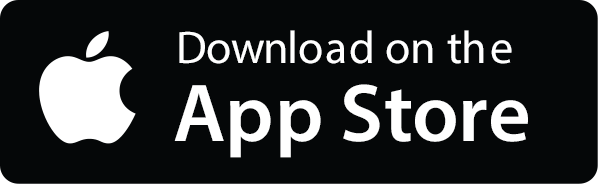
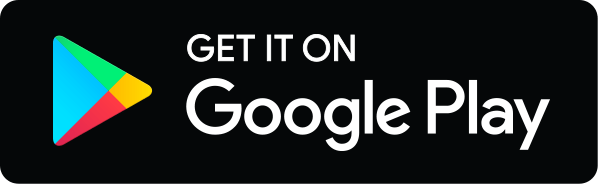