Chapter 1 The prevention of infectious disease transmission from human exposure to contaminated food, water, soil, and air remains a major task of environmental and public health professionals. There are numerous microbial hazards, including exposure via food, water, air, and malicious release of pathogens that may arise. Indeed, some have argued that the property of virulence of human pathogens is one which is favored by evolutionary interactions between pathogens and host populations and therefore will always be of important concern [1]. To make rational decisions in preparing, responding, and recovering from exposures to such hazards, a quantitative framework is of high benefit. The objective of this book is to comprehensively set forth the methods for assessment of risk from infectious agents transmitted via these routes in a framework that is compatible with the framework for other risk assessments (e.g., for chemical agents) as set forth in standard protocols [2, 3]. In this chapter, information on the occurrence of infectious disease in broad categories will be presented, along with a historical background on prior methods for assessment of microbial safety of food, water, and air. This will be followed by an overview of key issues covered in this book. Outbreaks of infectious waterborne illness continue to occur, although it remains impossible to identify the infectious agent in all cases. For example, in 1991, a waterborne outbreak in Ireland resulting from sewage contamination of water supplies infected about 5000 persons. However, the infectious agent responsible for this outbreak could not be determined [4]. In the United States, it has been estimated that 38 million cases of foodborne infectious disease occur annually with unidentified agents [5]. In the United States, there have typically been three to five reported outbreaks per year in community drinking water systems involving infectious microorganisms, with perhaps up to 10,000 annual cases [6]. The 1994 Milwaukee Cryptosporidium outbreak with over 400,000 cases [7, 8] was a highly unusual event among these statistics. As shown in Figure 1.1, there has been an increasing ability to identify microorganisms responsible for waterborne diseases, and it is expected that with advances in molecular biology, this will increase. Figure 1.1 Percentages of outbreaks associated with public water systems (n = 680) by time period 1971–2006 that had unknown etiologies based on data from Ref. [6]. There are substantially more outbreaks and cases of foodborne infectious diseases than are reported. Table 1.1 summarizes reports of U.S. cases of principal microbial infectious foodborne illnesses for two 5-year periods (1988–1992 and 2002–2006). There is a mix of causal agents, including bacteria, virus, and protozoa. It is noteworthy that (as in the case of waterborne outbreaks) the frequency of outbreaks of unknown etiology has dramatically decreased but the frequency of outbreaks associated with norovirus has dramatically increased. These changes are due in part to the ability to better identify causal agents (e.g., via molecular methods). Table 1.1 Comparison of Five-Year Averages for Common Foodborne Reported Outbreaks Source: From Refs. [9, 10]. a Include both Shiga toxigenic and enterotoxigenic. It is generally recognized that reported outbreaks, either of water- or foodborne infectious disease, represent only a small fraction of the total population disease burden. However, particularly in the United States, voluntary reporting systems and the occurrence of mild cases (for which no medical attention is sought but nevertheless are frank cases of disease) have made it difficult to estimate the total caseload. In the United Kingdom, comparisons between the number of confirmed cases in infectious disease outbreaks and total confirmed laboratory illnesses (occurring in England and Wales) have been made (Table 1.2). This suggests that the ratio of reported outbreak cases to total cases that may seek medical attention may be from 10 to 500:1, with some dependency on the particular agent. Table 1.2 Comparison of Laboratory Isolations and Outbreak Cases in England and Wales, 1992–1994 Source: Modified from Ref. [11]. Colford et al. [12] developed estimates for the total disease burden associated with acute gastroenteritis from drinking water. This relies on combining the reported outbreak data with interventional epidemiologic studies. Based on their analysis, the total U.S. disease burden is estimated to be 4.26–11.69 million cases per year in the United States, which is substantially in excess of the reported outbreaks. In the case of foodborne illness, there are an estimated 14 million cases per year [13]. Drinking water and food are by no means the only potential routes of exposure to infectious agents in the environment. Recreation in water (either natural or artificial pools) containing pathogens can produce illness [14]. Indoor air transmission can be a vehicle of infection. Legionella transmitted through indoor environments has been a concern since the 1970s [15]. The multinational epidemic of severe acute respiratory syndrome (SARS), caused by a coronavirus, was abetted at least in one location in Hong Kong by indoor aerosol transmission between apartments of infected individuals and susceptible individuals [16]. A broad spectrum of other respiratory pathogens including influenza, rhinoviruses, and mycobacteria can be transmitted by this route [17]. The deliberate release of Bacillus anthracis spores in 2001 (the “Amerithrax” incidents) brought widespread awareness to the potential for indoor releases (as well as releases in other venues) of bioterrorist agents to cause risk [18]. Therefore, of necessity, microbial risk assessors may need to consider the impact of malicious activity in certain applications. Concerns for microbial quality of food, water, and other environmental media have long existed. In the early twentieth century, the use of indicator microorganisms was developed for the control and assessment of the hygienic quality of such media and the adequacy of disinfection and sterilization processes. The coliform group of organisms was perhaps first employed for this purpose [19–21]. Indicator techniques have also found utility in the food industry, such as the total count for milk and other more recent proposals [22]. Other indicator groups for food, water, or environmental media have been examined, such as enterococci [23–25], acid-fast bacteria [26], bacteriophage [27–29], and Clostridia spores [29–31]. The use of indicator organisms was historically justified in because of difficulty in enumerating pathogens. However, with the increasing availability of modern microbial methods, for example, PCR, immunoassay, etc., for direct pathogen assessment, this justification has become less persuasive. In addition, in order to develop health-based standards from indicators, extensive epidemiologic surveillance is often necessary. The use of epidemiology has limitations with respect to detection limits (for an adverse effect) and is also quite expensive to conduct. Indicator methods are also limited in that many pathogens are more resistant to die off in receiving environments or source waters than indicators or have greater resistance to removal by treatment processes than indicators [26, 28, 29, 32]. Thus, the absence of indicators may not suffice to ensure the absence of pathogens. Even after a century of use, the indicator concept remains imperfect [33]. The use of quantitative microbial risk assessment (QMRA) will enable direct measurements of pathogens to be used to develop acceptance/rejection guidelines for food, water, and other vehicles that may be the source of microbial exposure to human populations. The objective of this book is to present these methods in a systematic and unified manner. QMRA is the application of principles of risk assessment to the estimate of consequences from a planned or actual exposure to infectious microorganisms. In performing a QMRA, the risk assessor aims to bring the best available information to bear in understanding the nature of the potential effects from a microbial exposure. Since the information (such as dose–response relationships, exposure magnitudes) is almost invariably incomplete, it is also necessary to ascertain the potential error involved in the risk assessment. With such information, necessary steps to mitigate, control, or defend against such exposures may be developed. At the outset of performing a risk assessment, a scoping task should be undertaken. This task should set forth the objectives of the analysis and the principal issues to be addressed. Items such as consideration of secondary cases, individual versus population risk, agent or agents to be examined, exposure routes, and/or accident scenarios must be stipulated. However, this scoping may be changed during the course of a QMRA, to reflect the input derived from the risk manager(s) and other stakeholders. There may be diverse objectives for a QMRA. These objectives relate to the rationale for the performance of the assessment, as well as the methods to be employed. Broadly, the different objectives reflect different scales at which a risk assessment may be performed. The step of problem formulation is critical to any risk estimate [34]. It is necessary that the problem be formulated to meet the needs of the risk managers and stakeholders; indeed, it is now recognized that the successful practice of risk analysis requires frequent interchange with manager and stakeholders [3]. In general, the problems posed are of several types. The simplest type of QMRA that may be performed involves one site or exposure scenario. The following are typical of the questions that might be asked:
Motivation
Prevalence of Infectious Disease
Agent
Annual Average 1988–1992
Annual Average 2002–2006
Cases
Outbreaks
Cases
Outbreaks
Campylobacter
99.6
4.4
624
22
Escherichia coli
48.8
2.2
481a
30a
Salmonella
4,235.4
109.8
3,475
144
Shigella
957.6
5
495
12
Staphylococcus aureus
335.6
9.4
554
25
Hepatitis
421.8
8.6
238
1
Listeria monocytogenes
0.4
0.2
22
2
Giardia
36.8
1.4
2
1
Norovirus
58.4
0.4
10,854
338
Vibrio (all)
11.4
1.8
114
5
Unknown etiologies
40,483
1,422
4,052
30
Agent
Cases: 1992–1994
Ratio
All Laboratory Reports
Confirmed Outbreak Cases
Campylobacter
122,250
240
509.4
Rotavirus
47,463
127
373.7
S. sonnei
29,080
847
34.3
Salmonella
92,416
5,960
15.5
Cryptosporidium
14,454
1,066
13.6
E. coli O157
1,266
128
9.9
Prior Approaches
Scope of Coverage
Potential Objectives of a QMRA
Site-Specific Assessment
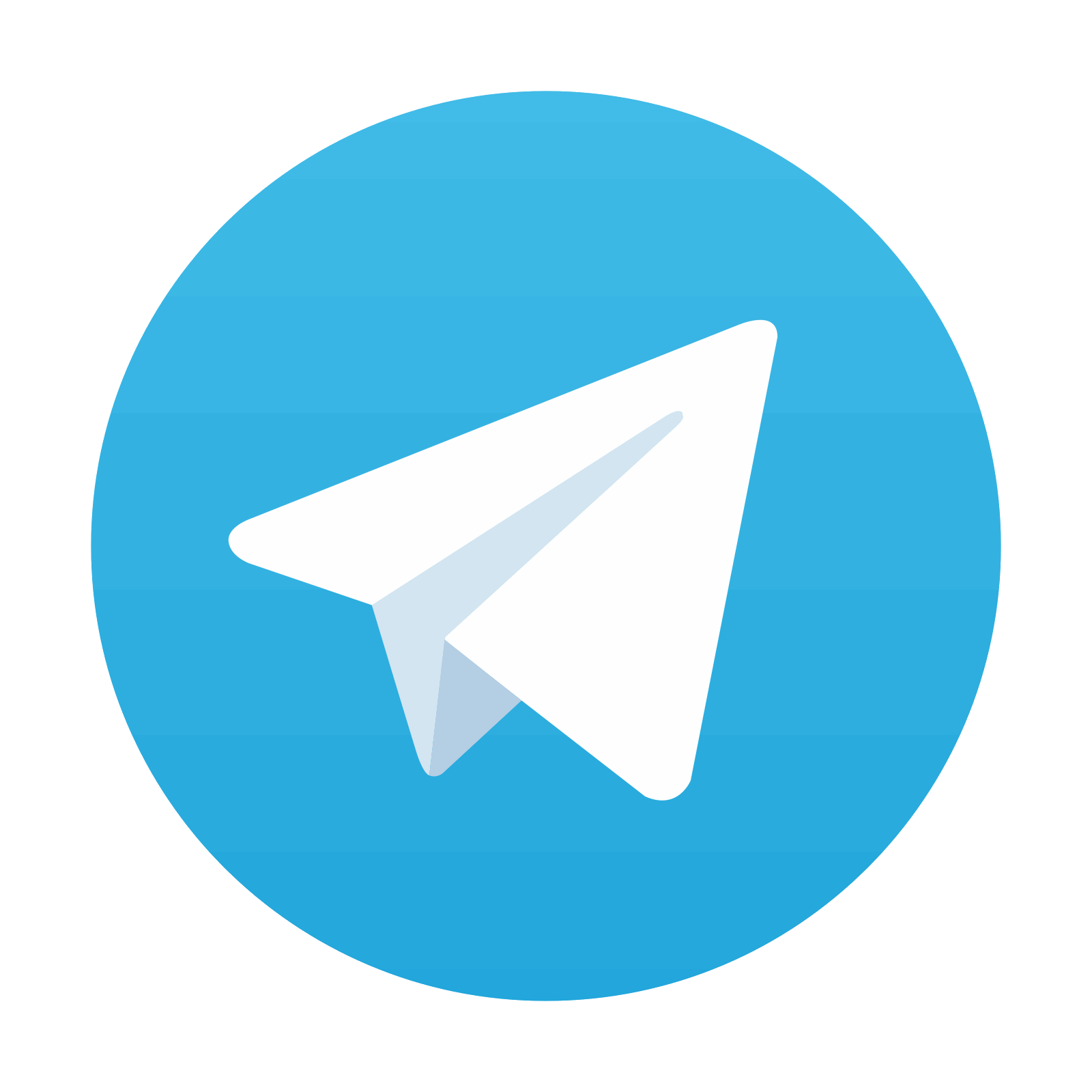
Stay updated, free articles. Join our Telegram channel

Full access? Get Clinical Tree
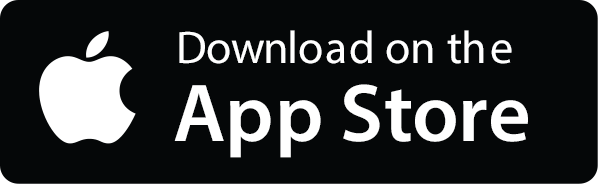
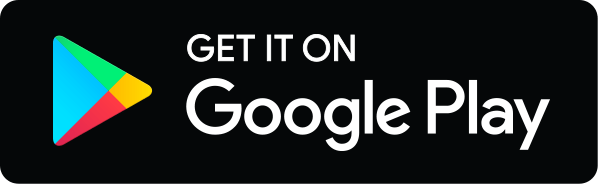