(1)
Faculté de Parmacie, Laboratoire de Biophotonique et Pharmacologie, UMR 7213 CNRS, Université de Strasbourg, Illkirch, France
Abstract
Environment-sensitive fluorescent membrane probes are attractive tools for investigating the membrane properties and their changes under perturbing conditions. Membrane probes of the 3-hydroxyflavone family are of particular interest due to their excited-state intramolecular proton transfer (ESIPT) reaction, which confers a dual emission highly sensitive to the polarity and hydration of the environment. In the present work, we will describe the protocols used with these probes in order to monitor the physicochemical properties of lipid membrane models and cell plasma membranes and to detect apoptosis.
Key words
Fluorescent membrane probeEnvironment-sensitive dyeExcited-state intramolecular proton transferF2N12SRatiometric responseCell membraneLipid orderLipid domainsApoptosis1 Introduction
Fluorescent membrane probes are important tools to investigate the physicochemical properties of model and cellular lipid membranes. The most common properties addressed by these probes are the membrane fluidity, hydration, and electrostatics, such as surface, dipole, and transmembrane potential [1]. These properties correlate with other membrane properties, which generally cannot be directly measured by these probes, such as lipid order, interlipid distance, phase transitions, and phase separations. Among the existing membrane probes, the 3-hydroxyflavone derivatives are of particular interest [2–4]. These probes exhibit the remarkable property to emit two bands, due to an excited-state intramolecular proton transfer (ESIPT). This dual emission provides an additional channel of spectroscopic information and through the ratio of the two bands offers a signal that is independent of the probe concentration. Particularly attracting is the probe F2N12S, which due to its apolar chain and zwitterionic group stains specifically the outer leaflet of plasma membranes, without rapid internalization inside the cells. In contrast to fluorescent lipids, this probe binds spontaneously lipid bilayers and thus does not require any helper agent, such as surfactants or cyclodextrins. Being bound to lipid membranes, the dual fluorescence spectrum of F2N12S is characterized by a short-wavelength band, originating from the emission of the normal N* (H-bonded and H-bond free) emissive species and a long-wavelength band assigned to the emission of the ESIPT tautomer T* [5, 6]. The dual emission of F2N12S is highly sensitive to several membrane properties. By deconvoluting the emission spectrum of F2N12S, both the polarity and hydration, two different properties of lipid membranes can be monitored simultaneously [4, 7]. The polarity parameter correlates well with the membrane electrostatics, i.e., surface and dipole potentials, while the hydration parameter shows strong variation in response to changes in the membrane phase state [1]. This bi-parametric sensitivity of F2N12S allowed its applications for detecting apoptosis and probing lipid domains.
Apoptosis, the programmed cell death, is a fundamental process controlling cell homeostasis. In normal cells, the plasma membrane is asymmetric, so that the outer leaflet presents a large quantity of sphingomyelin (SM), while phosphatidylserine (PS) and phosphatidylethanolamine (PE) are mainly concentrated in the inner leaflet [8]. In apoptotic cells, due to inactivation of flippases, this asymmetry is lost, so that PS is exposed at the outer leaflet. Moreover, PS exposure is associated with a decrease in SM at the outer leaflet [9], which may decrease the fraction of the liquid-ordered (Lo) phase at this leaflet. Both exposure of PS and loss of Lo phase induce a significant change in the dual emission of F2N12S, providing an increase in the relative intensity of the short-wavelength band [4].
Another application of probe F2N12S is to monitor lipid domains. In cell membranes, lipid domains attracted large attention since they are thought to be involved in the regulation of various cell functions such as signal transduction, lipid trafficking, and membrane protein activity [10]. Lipid domains composed of saturated lipids (SM in particular) and cholesterol form a liquid-ordered (Lo) phase. These domains are thought to float in the liquid-disordered (Ld) phase, formed by unsaturated lipids and cholesterol. As the F2N12S probe is sensitive to the phase state, it was applied for imaging lipid domains in giant vesicles, showing a good contrast between Lo and Ld phases in ratiometric images [3]. In cell membranes of astrocytoma, we further evidenced the presence of a high fraction of Lo phase, which could be substantially decreased by cholesterol extraction [4]. Remarkably, cholesterol extraction gave the same spectroscopic response as apoptosis, suggesting that both phenomena lead to similar loss in the Lo phase at the outer membrane leaflet. Thus, the F2N12S probe appears as a promising tool for monitoring the biophysical properties of model and cell membranes and for probing lipid domains and apoptosis. In the present work, we review the sensing methodology based on F2N12S for these two applications. In particular, we will present protocols for (a) spectroscopic studies of phase state in model lipid membranes, (b) imaging membrane domains in giant unilamellar vesicles, (c) spectroscopic studies of membrane modifications due to apoptosis and cholesterol extraction, (d) detection of apoptosis by flow cytometry, and (e) imaging apoptosis by fluorescence microscopy.
2 Materials
Prepare stock solution of 0.1 mM (0.64 g/L) F2N12S in DMSO and store it at −20 °C. Dioleoylphosphatidylcholine (DOPC) and cholesterol were purchased from Sigma-Aldrich (Lyon, France). Bovine brain sphingomyelin was from Avanti Polar Lipids (Alabaster, USA).
All lipids were dissolved in chloroform.
2.1 Large Unilamellar Vesicles
1.
20 mM of phosphate buffer, pH 7.4 (10 mL).
2.
1 mM of lipids (DOPC, sphingomyelin, cholesterol) in chloroform.
3.
Lipex Biomembranes extruder (Vancouver, Canada) with two sizes of filters, 0.2 and 0.1 μm, respectively.
2.2 Giant Unilamellar Vesicles
1.
Homebuilt liquid cell (University of Odense, Denmark) with platinum wires.
2.
0.1 mM of lipids (DOPC, sphingomyelin, cholesterol) in chloroform.
3.
300 mM of sucrose solution in mQ water (500 μL/sample).
4.
300 mM of glucose solution in mQ water (200 μL/sample).
5.
Thermostat.
6.
Power supply: 2 V, 10 Hz.
2.3 Cell Culture
1.
X-VIVO 15 serum-free medium (Cambrex, France) for human lymphoid CEM T cells.
2.
EMEM (Eagle’s minimal essential medium, Lonza): with 10 % heat-inactivated fetal bovine serum (PAN Biotech GmbH), 0.6 mg/mL glutamine (Biowhittaker) for U87MG human glioblastoma cell line.
3.
DPBS (Dulbecco’s Phosphate Buffered Saline-Lonza): without Ca2+ and Mg2+.
4.
HBSS (Hank’s Buffered Salt Solution-Sigma): with NaHCO3 (0.35 g/L), without phenol red, calcium chloride and magnesium sulfate.
5.
1× of trypsin diluted in phosphate buffer.
6.
Opti-MEM: Reduced Serum Medium from Invitrogen.
7.
Actinomycin D (Sigma-Aldrich): dissolved in DMSO, stored at −20 °C.
8.
Methyl-β-cyclodextrin (Sigma-Aldrich): dissolved in DPBS, stored at +4 °C.
9.
35 mm imaging dishes (μ-Dish35mm, low, IBiDi) to prepare cells for microscopy experiments.
10.
Cell lines: human lymphoid CEM T cells and U87MG human glioblastoma cell line.
2.4 Fluorescence Spectroscopy and Microscopy
1.
Jobin-Yvon Fluoromax 3 spectrofluorometer equipped with a thermostated cuvette holder was used to record fluorescence spectra in vesicles and cells.
2.
Quartz cuvettes of 1 mL were used to measure fluorescence spectra.
3.
For fluorescence microscopy experiments, a homebuilt two-photon laser scanning setup based on Olympus IX70 inverted microscope with an Olympus 60× 1.2 NA water immersion objective was used. Two-photon excitation was provided by a titanium-sapphire laser (Tsunami, Spectra Physics), and photons were detected with avalanche photodiodes (ADP SPCM-AQR-14-FC, Perkin Elmer) connected to a counter/timer PCI board (PCI6602, National Instrument). Imaging was carried out using two fast galvo mirrors in the descanned fluorescence collection mode. Typical acquisition time was 20 ms with an excitation power around 20 mW (λ = 830 nm) at the sample level. Images corresponding to the short-wavelength and the long-wavelength emission bands were recorded simultaneously using a dichroic mirror (Beamsplitter 550DCXR) and two band-pass filters (Brightline HC 520/20 and HQ 585/40) in front of the APDs.
2.5 Flow Cytometry
1.
Annexin V-FITC Kit: from Immunotech.
2.
Propidium iodide (PI): from Sigma.
3.
Binding buffer provided with the Annexin V-FITC kit: 10 mM Hepes/NaOH, pH 7.4, 140 mM NaCl, 2.5 mM CaCl2.
4.
F2N12S.
5.
FACSAria Cell Sorter (BD Biosciences, CA) equipped with an argon laser (488 nm) and a diode laser (405 nm) and emission filters DF30, DF42, and DF20 centered at 530, 585, and 610 nm, respectively.
6.
FACSDiva Software (flow cytometer analysis software from BD Biosciences).
3 Methods
Carry out all procedures at room temperature unless otherwise specified.
3.1 Spectroscopic Studies of Phase State in Model Lipid Membranes
3.1.1 Large Unilamellar Vesicle Preparation and Staining
1.
Dissolve 5 mM of lipids: DOPC (19.65 mg), DOPC/35 % Chol (12.78 mg DOPC + 3.38 mg Chol), or SM/35 % Chol (11.88 mg of SM + 3.38 mg Chol) in 5 mL chloroform to ensure their homogeneous distribution.
2.
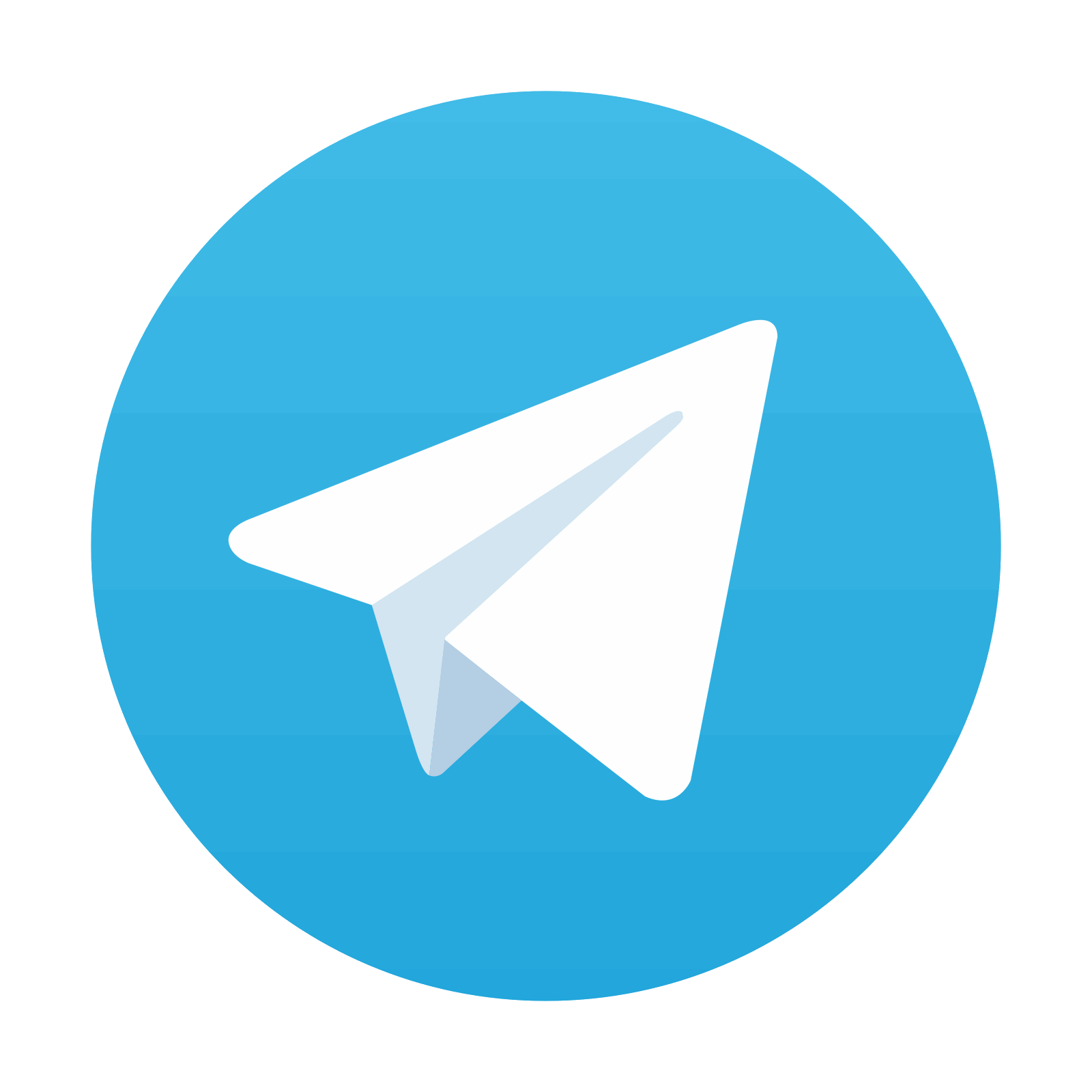
Evaporate chloroform by rotary evaporation to get a dry thin lipid film on the wall of a round-bottom flask with gentle heating (about 35 °C) during 30 min.
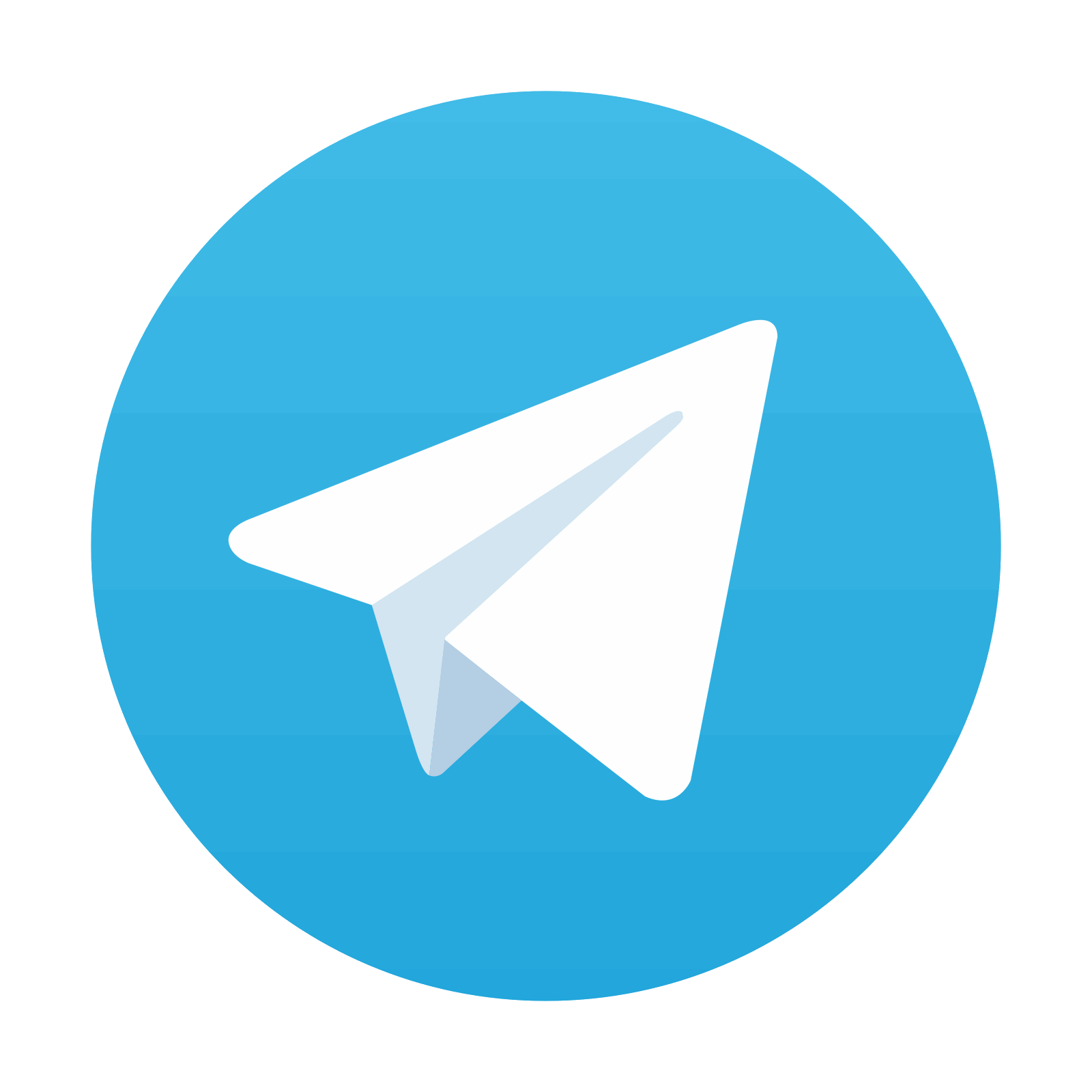
Stay updated, free articles. Join our Telegram channel

Full access? Get Clinical Tree
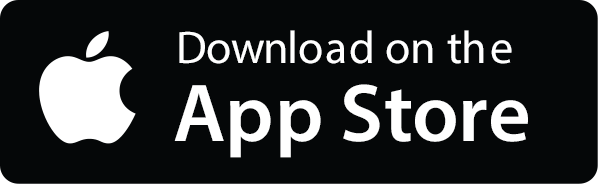
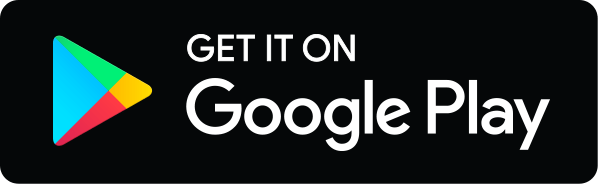
