Overview
Substance use disorders represent complex phenotypes that result from the intricate interplay of genetic variation, neurobiological mechanisms, psychosocial variables, and environmental variables. To date, one of the least-studied factors has been genetic variation. However, basic research on the human genome is progressing at a rapid pace, and investigations of genetic factors that influence the etiology and treatment of substance use disorders are now much more common. The promise of this research is that it may help scientists optimize the success of treatments by matching specific treatments with individuals who have specific genetic vulnerabilities. The ability to match a specific treatment with an individual who is most likely to benefit from that treatment is especially exciting because, while a number of treatment alternatives exist, the overall effectiveness of these treatments is quite modest and there are currently no objective criteria that can be used to match an individual with the treatment that is most likely to be effective. It is only a matter of time before much of the genetic variation that contributes to the risk of addiction is uncovered and, likewise, only a matter of time before clinicians begin to utilize genetic information to match individuals with the treatment that is safest and most likely to benefit them.
This chapter provides a critical review of the expanding literature with respect to molecular genetics and the treatment of addiction. First, we present a brief overview of key concepts in the genetics of addictions. Second, we provide a more extended review and discussion of pharmacogenetics and pharmacogenomics applied to addiction medicine. Recent studies of genetic differences and responses to pharmacological, and to a lesser degree psychosocial, treatments for addictions will be reviewed for various substances of abuse, including alcohol, nicotine, cocaine, and opiates. Third, we discuss practical and ethical issues in the translation of pharmacogenetics science into clinical practice. Fourth, we outline several future directions for the field of molecular genetics applied to the treatment of addictions. Finally, we present a summary and some concluding remarks.
Genetics and the Treatment of Addictions
Twin and adoption studies have suggested that approximately 50% of the variance in risk for developing alcohol dependence can be explained by genetic factors. Likewise, studies have demonstrated that genetic factors account for a significant portion of variance in drug use, abuse, and dependence. a
a References 9, 43, 44, 66, 68, 138, 139.
The progression from initial use to abuse or dependence for substances such as marijuana and cocaine also appears to be largely due to genetic factors.Just as the etiology of substance use disorders appears to be under moderate genetic control, so does the response to pharmacotherapies. Broadly speaking, evidence for heritability of medication effects in psychiatry dates as far back as 1967, when heritable variation in plasma concentration of the tricyclic antidepressants, desipramine and nortriptyline, were first shown in twin and family studies. In addition, more recent research has documented the heritability of response to typical antipsychotics, including differences in antipsychotic response among ethnic groups. As discussed in detail below, genetic factors also seem to play a role in response to pharmacotherapies, and perhaps psychosocial treatments, for substance use disorders.
After determining that genetic variation plays a substantial role in the etiology of addictive disorders and the response to treatment through family, twin, and adoption studies, the next step in genetics research often consists of identifying specific genetic variations that contribute to the etiology and response to treatment for these disorders. In many ways, research on genetics of addiction has already transitioned from establishing that genetic variables contribute to the variance in a disorder to identifying the specific genetic variables that actually contribute to the disorder.
Currently, there are two basic approaches to the identification of genetic variations that influence substance use disorders and/or treatment outcomes. The first is a hypothesis-driven approach, in which investigators develop a priori hypotheses based on what is known about the genetic variation and the neurobiology of the disorder or the mechanism of action for a specific treatment. For example, one might hypothesize that a specific genetic variation that influences the mu opioid receptor expression might also predict acute responses to alcohol and the effects of a medication (e.g., naltrexone) that targets this receptor.
In many cases, it is more common to work with a gene for which function variations have yet to be identified. In this situation, a variation on the approach described earlier is to hypothesize that a gene is related to a specific aspect of a substance use disorder or the effects of a medication and then use special analytic approaches to probe genetic variation across the entire gene. This approach is commonly known as a haplotype-based approach, and is designed to capture most of the genetic variation across the gene even when the function variations have not been identified. To that end, “tag single nucleotide polymorphisms” are often used, as they allow scientists to capture genetic variation in various loci by genotyping fewer, but informative, markers. More specifically, tag single nucleotide polymorphisms are selected on the basis of patterns of linkage disequilibrium that indicate whether several polymorphisms are highly correlated, in which case, instead of having to genotype all markers, scientists can identify a few that strongly predict genetic variation in a given area or locus. This approach has become increasingly accessible due to the availability of bioinformatics resources, most notably results from the International HAPMAP Project, which have been made publicly available in the user-friendly HAPMAP Project website ( http://www.hapmap.org/ ). The next step after identifying tag single nucleotide polymorphisms for areas of interest is often to build haplotypes, which describe common patterns of DNA sequence variation. In fact, the objective of the HAPMAP Project is to develop a haplotype map of the human genome, which in turn can aid scientists in finding genes affecting health, disease, and responses to medications and environment. A detailed review of haplotype-based techniques in pharmacogenetics is beyond the scope of this chapter and can be found elsewhere.
Finally, a more recent approach is to conduct exploratory genome-wide analyses to identify genetic variation that influences substance use disorders or responses to medications. The genome-wide association study is currently in vogue and represents one of the most cutting-edge approaches in terms of identifying sources of genetic variation that may eventually be used to predict response to treatment. A genome-wide association study utilizes a high-density single nucleotide polymorphism array to generate data on more than one million genetic markers (e.g., using the Illumina 1 M array). This vast array of genetic data can then be analyzed in combination with a set of phenotypes. A number of reviews have been published recently on the advantages, disadvantages, limitations, and recommendations associated with this approach. One obvious problem with this approach is the sheer number of statistical tests and the resulting increase in type I error that may lead to false positives. A corollary is the requirement of strict statistical corrections and the need for massive sample sizes. To date, there have been a number of genome-wide association study reports in the psychiatric genetics literature, including major depressive disorder, bipolar disorder, and schizophrenia, but only one that involves a substance abuse disorder, namely nicotine dependence. Although genome-wide association is the approach du jour and has generated much excitement in the field, it is important to note that genome-wide association studies represent a transition to even more difficult and time-consuming work. Once new genetic variations are identified, models will need to be developed and hypothesis-driven research will be needed to translate the effect of genetic variation uncovered in the genome-wide association studies regarding the effect of the genetic variations from the molecular level, to the cellular level, to the systems level, and to the behavioral level in order to understand the implications of these findings for the etiology, prevention, and treatment of substance use disorders. This translation will likely lead to new findings on as-yet unknown neuronal mechanisms that influence the development of substance use disorders and lead to new targets for pharmacotherapies as well as generate information about which individuals will be most likely to respond to those new pharmacotherapies.
Pharmacogenetics and Pharmacogenomics
Pharmacogenetics is a field of research that seeks to understand individual differences in the metabolism and efficacy of medications. As described by Vogel, pharmacogenetics is the study of heritable differences in the metabolism and activity of exogenous agents, including medications and environmental toxins. Current pharmacogenetics research focuses on identifying genetic factors that account for variability in pharmacotherapy effects, in terms of both pharmacodynamics and efficacy. In recent years, the term pharmacogenomics has been defined as the application of genomics to the study of pharmacogenetics. In brief, the distinction between the two terms refers to the methodological and theoretical approach such that pharmacogenetics investigations are generally hypothesis driven and focus on a few loci at a time. Conversely, pharmacogenomics investigations include the use of high-throughput genotyping and genome-wide association approaches to understanding genetic determinants of pharmacotherapy response. In essence, the objective of pharmacogenomics is the same as pharmacogenetics, which is to elucidate genetic variants that influence the efficacy and safety of pharmacotherapies. For simplicity, we will refer simply to pharmacogenetics in this chapter.
The field of pharmacogenetics has grown rapidly and has benefited greatly from advancements in molecular genetics tools for identifying gene polymorphisms, developments in bioinformatics and functional genomics, and new findings from the human genome project. The foremost goal of this line of research is to optimize pharmacotherapy by identifying genetic factors that predict who is more likely to respond to certain pharmacotherapies and who will not respond, thereby matching individuals to medications on the basis of genetic factors. Genetic factors can account for individual differences in medication toxicity and response in many ways. Genetic polymorphisms may lead to functional differences in medication metabolism and disposition, such as functional differences in enzyme activity or medication transporters. Alternatively, genetic polymorphisms may impact the target of a medication, such as a particular receptor. An example of the first case is a polymorphism of the CYP2D6 gene, which is involved in the availability of specific medication-metabolizing enzymes associated with one’s response to opioid painkillers, such as codeine or morphine. Individuals who are homozygous for the nonfunctional CYP2D6 alleles were found to be resistant to the analgesic effects of opioid painkillers. It is important to note that this response in poor metabolizers may be moderated by ethnicity. Specifically, individuals who carry the CYP2D6∗10 allele, which is most common among Chinese individuals, have reduced metabolism of opioid analgesics, such as codeine or tramadol. Furthermore, treatments such as methadone maintenance therapy can inhibit the metabolism of tramadol to O -desmethyltramadol by interfering with CYP2D6 activity. This has important implications for therapy as those individuals receiving methadone may not experience the same degree of opioid analgesia, as those receiving other treatment, such as buprenorphine maintenance. Thus both ethnic variation in allele types and treatment considerations are important when determining the potential efficacy of opioid analgesics.
On the other hand, genetic polymorphisms involved in a medication’s target may also impact one’s response to pharmacotherapy. For example, polymorphisms of the dopamine D4 receptor gene ( DRD4 ) have been associated with differential response to antipsychotic medications. For example, a recent finding suggests that for the DRD4 exon III repeat polymorphism, the 4R allele is associated with better clozapine response, but other individual single nucleotide polymorphisms (SNPs) in this study showed no association with clozapine response. Similarly, when individual SNPs of DRD4 (rs1800955 and rs4646984) were analyzed, they did not show any associations with antipsychotic drug response. Thus future studies may consider further exploring the efficacy of the 48-bp repeat polymorphism of the 4R allele. There is also a growing literature on the pharmacogenetics of antidepressant medications. Specifically, research has suggested that the functional polymorphism of the serotonin transporter gene located in the 5′ upstream regulatory region consisting of a 44-bp insertion/deletion, which results in a long or short variant, predicts response to various selective serotonin reuptake inhibitors, including fluoxetine, fluvoxamine, and paroxetine. Carriers of the long allele of the serotonin transporter promoter polymorphism have better clinical response to antidepressant medications compared with individuals who are homozygous for the short allele, which results in twofold decreased expression and transport activity of the receptor in vitro. These results suggest that pharmacogenetics may soon inform a more targeted use of antidepressant medications. In addition to medications’ efficacy, pharmacogenetics research has focused on identifying susceptibility loci contributing to adverse effect profiles and medications’ toxicity, thereby enhancing the safety profile of pharmacotherapies. Next we review pharmacogenetic studies in the field of addictions to various substances of abuse.
Nicotine
Currently, there are two non-nicotine pharmacotherapies approved by the FDA for the treatment of nicotine dependence, namely bupropion hydrochloride and varenicline. In addition, there are five FDA-approved nicotine replacement therapies, which vary mostly in terms of their delivery kinetics; these include transdermal patch, gum, lozenge, inhaler, and nasal spray. Several candidate genes have been subjected to pharmacogenetic studies, mostly those of nicotine replacement therapies and bupropion, as described in recent reviews of the pharmacogenetics of smoking cessation. Specifically, pharmacogenetic studies of nicotine dependence have examined genes underlying the metabolism of nicotine, focusing primarily on the cytochrome P450 (CYP)2A6 gene ( CYP2A6 ). This gene codes for the primary enzyme that converts nicotine to cotinine and cotinine to 3-hydroxycotinine. In a study of transdermal patch and nasal spray nicotine replacement therapies, at the same levels of nicotine replacement, carriers of CYP2A6 alleles coding for a slower metabolism were found to have higher plasma nicotine concentrations following 1 week of the nicotine patch than normal metabolizers. Those differences were not seen using the nasal spray, and at 6-month follow-up, slow metabolizers had higher quit rates in the transdermal patch condition, as compared with normal metabolizers. A community sample of treatment-seeking nicotine-dependent patients who received transdermal nicotine therapy were also found to benefit more from the treatment if they were slow metabolizers, whereas another study found that extended release transdermal nicotine therapy (24 weeks) was more effective than standard therapy (8 weeks) in slow metabolizers. Other important considerations may be examining whether interactions between the CYP2A6 gene and other genes predict quit success rates. For example, a study investigating the role of serotonergic systems in treatment response found that slow metabolizer patients with the 5-HTTL allele or HTR2A-1438GG alleles benefited more from nicotine replacement therapy, suggesting reduced levels of synaptic serotonin may be a mechanism by which slow metabolizers may show better success for smoking cessation. However, the results have not been consistent, and a study found that slow metabolizers had higher relapse rates when treated with the nicotine patch, whereas another study reported that nicotine replacement therapy was more effective in fast metabolizers. It is important to note that findings may also depend on the treatments being tested. For example, in one study, bupropion was shown to result in 1.7 times longer abstinence in slow metabolizers than fast metabolizers. More research is needed to identify the reason for discrepancies across studies, but the CYP2A6 gene appears to be a promising target for translating personalized treatment to the clinic.
In addition, nicotinic receptor genes have been subjected to pharmacogenetic investigations. Nicotine binds to nicotinic acetylcholine receptors, which are ligand-gated ion channels for which there are several subunits. Allelic variation in the gene coding for the nicotinic acetylcholine receptor’s α4 subunit (CHRNA4) has been associated with nicotine dependence. More recent molecular work has suggested that certain single nucleotide polymorphisms in the CHRNA4 gene are functional and related to smoking cessation during nicotine replacement therapy and varenicline treatment. Although promising, these findings await replication. Likewise, a series of studies have examined the role of functional genetic variation in the DRD2 in response to bupropion and nicotine replacement therapy. Results revealed that the DRD2–141C Ins/Del genotype was associated with treatment response to bupropion, such that smokers homozygous for the Ins C allele had a more favorable response to treatment compared with those carrying the Del C allele. Conversely, regardless of nicotine replacement therapy type, those carrying the Del C allele had higher quit rates from nicotine replacement therapy compared with those homozygous for the Ins C allele. Additional polymorphisms that have received attention as putative genetic moderators of smoking cessation in response to nicotine replacement therapies and bupropion include dopaminergic genes (e.g., the Val/Met single nucleotide polymorphism of the catechol- O -methyltransferase gene), opioidergic genes (e.g., Asn40Asp single nucleotide polymorphism of the OPRM1 gene), and serotonergic genes (e.g., the serotonin transporter promoter polymorphism).
Perhaps one of the more exciting new developments in the pharmacogenetics of smoking cessation is a series of genome-wide association studies of smoking cessation with bupropion and nicotine replacement therapy. These studies revealed that genetic variants in quit-success were likely to alter cell adhesion, enzymatic, transcriptional, structural, and protein-handling functions. The genes identified through these genome-wide association studies had modest overlap with genes associated with addictions and memory processes. Clearly, as noted previously, there are limitations to the genome-wide association approach, and these results should be interpreted with caution until replicated in an independent sample.
Alcohol
Several studies to date have investigated genetic polymorphisms in the context of pharmacotherapies for alcohol dependence. Naltrexone, a mu-opioid receptor antagonist, is one of the very few pharmacotherapies currently approved for the treatment of alcoholism by the US Food and Drug Administration (FDA). From a pharmacogenetics perspective, there has been recent interest in the gene coding for mu-opioid receptors ( OPRM1 ), as they represent a primary target of naltrexone. More specifically, studies have focused on the Asn40Asp mutation of the OPRM1 gene, given evidence that this nonsynonymous mutation leads to an amino acid change, which in turn codes for more potent receptors. Human laboratory studies have shown that the Asp40 allele of the OPRM1 gene is associated with greater sensitivity to the reinforcing effects of alcohol and greater neural activation in the mesocorticolimbic structures following a priming dose of alcohol.
In a pharmacogenetic study of naltrexone, Oslin and colleagues found that the Asn40Asp allele of the OPRM1 gene was associated with clinical response to naltrexone for the treatment of alcohol dependence. The relationship was such that individuals with at least one copy of the Asp40 variant showed lower relapse rates and longer time to return to heavy drinking when treated with naltrexone, as compared with homozygotes for the Asn40 allele. These findings have been recently replicated and extended in the multisite COMBINE Study, such that carriers of the Asp40 allele of the OPRM1 gene had better clinical response to naltrexone, in combination with medication management, as compared with homozygotes for the Asn40 allele. A double-blind, placebo-controlled laboratory trial of naltrexone (50 mg) found that carriers of the Asp40 allele of the OPRM1 gene showed significantly greater naltrexone-induced blunting of the alcohol high, as compared with individuals who were homozygous for the Asn40 allele. These findings suggest that the differential clinical response to naltrexone may be due to differential blunting of alcohol-induced reward as a function of genotype and propose a mechanism for this important pharmacogenetic relationship. Nevertheless, there have been null findings regarding the association between this functional polymorphism and the efficacy of naltrexone for alcoholism such that further work is necessary before these findings can be translated into clinical practice.
Limitations notwithstanding, the pharmacogenetics of naltrexone and the putative moderating effects of a functional single nucleotide polymorphism of the OPRM1 gene represent an interesting line of work that is both promising and exciting from a pharmacogenetics perspective. In this case, functional variation in a gene coding for a medication (i.e., naltrexone) target, namely mu-opioid receptors, may be used to predict the efficacy of a pharmacotherapy. This case most likely represents an exception, rather than the rule, in pharmacogenetics research as most pharmacotherapies do not have such targeted neurobiological effects and the functional significance of most single nucleotide polymorphisms are not well characterized.
It is important to note, however, that ancestral background can moderate the efficacy of pharmacotherapeutic treatments. For example, the prevalence of the Asp40 allele varies by ancestral background, such that individuals of East Asian ancestry, are more likely to carry the Asp40 allele. This suggests that this population may particularly benefit from naltrexone treatment. A study of Korean alcohol-dependent adults found that treatment-adherent patients who carried the Asp40 allele (G allele) took significantly longer to relapse than Asn40 homozygotes. Again, the underlying mechanism of this effect may be through a reduction of reward-associated craving, as an intravenous alcohol administration study showed that individuals of East Asian descent who carried the Asp40 allele were less likely to crave alcohol when administered with naltrexone relative to placebo or those participants who were Asn40 homozygotes.
Using a similar hypothesis-driven approach, a series of pharmacogenetic studies have tested the association between olanzapine, a medication that targets dopamine receptors, alcohol craving, and a polymorphism of the dopamine D4 receptor gene (DRD4 variable number tandem repeat). One study found an association between the 7-repeat allele of the DRD4 variable number tandem repeat polymorphism and increased craving for alcohol after a priming dose of alcohol. Another study found that olanzapine decreased craving after a priming dose of alcohol in a nonclinical sample of college drinkers. Finally, results from a recent clinical trial revealed that the efficacy of olanzapine in the treatment of alcohol dependence was moderated by this genetic variation, such that individuals with at least one copy of the long allele showed greater reductions in cue-elicited craving and greater decreases in alcohol consumption during the 12-week clinical trial, as compared with individuals who were homozygous for the short allele. This genetic variation has also been associated with increased activation of mesocorticolimbic regions during the presentation of alcohol cues. Together these series of laboratory and clinical trials have established a relationship between a polymorphism of the DRD4, craving for alcohol in the laboratory, and response to a medication that targets dopamine receptors. Despite this intriguing relationship, much work is needed to determine whether there is clinical utility of olanzapine for treating alcohol dependence. For example, a recent 12-week clinical trial that did not examine genetic moderators of olanzapine response, suggests that despite reductions in craving and proportion of drinking days with olanzapine treatment, the cost-benefit of this medication does not currently support its clinical utility.
The approach in this series of studies of olanzapine for alcoholism was theory driven and focused on intermediate phenotypes for alcoholism, in this case alcohol craving, rather than the diagnostic phenotype of alcohol dependence per se. There are important advantages to a theory-driven pharmacogenetics approach, such as the ability to answer more specific questions about the mechanisms of action of pharmacotherapies, genetic variants, and biobehavioral risk makers of complex disorders, such as alcoholism. It is notable that theory-driven approaches can be complementary to data-driven pharmacogenomics investigations, which are likely to become increasingly accessible given recent advances in DNA genotyping technology.
Topiramate, an antiepileptic medication that is a glutamatergic antagonist and γ-aminobutyric acid (GABA)ergic agonist, has also shown promise for the treatment of alcohol dependence across several studies. A study of problem drinkers by Kranzler and colleagues found that topiramate was able to significantly reduce heavy drinking days compared to placebo, and in European Americans, this effect was moderated by the SNP rs2832407 in the glutamate receptor, ionotropic, kainate 1 ( GRIK1 ) gene. Specifically, CC homozygotes reduced their drinking significantly more than A allele carriers. This same gene has also been shown to be associated with the severity of topiramate-induced side effects and the topiramate serum concentration, which were both found to be higher in A allele carriers than CC homozygotes. Furthermore, the findings by Kranzler and colleagues were extended in a subsequent study that suggested that the GRIK1 genotype also moderated positive expectancy of alcohol’s effects and the desire to drink when patients’ daily reports on these measures were examined. This was followed by an analysis that indicated an interaction between genotype, expectancies, and medication, such that topiramate was less effective in CC homozygotes at reducing heavy drinking on days when more positive effects of alcohol were expected. This series of studies not only showed that the GRIK1 genotype may be an important marker of topiramate’s efficacy, but that the genotype is associated with cognitive processes and craving associated with alcohol use. It is notable that treatment, genotype, and cognitive processes may interact to predict heavy drinking days in problem drinkers.
Cocaine
Currently, there are no FDA-approved pharmacotherapies for the treatment of cocaine dependence; however, many recent advances have taken place in the search for medications to treat this disorder. Medications such as dopamine agonists, disulfiram, the cocaine vaccine, and GABAergic treatments have all been explored, and many of them have undergone testing in phase II or phase III clinical trials. For example, the cocaine vaccine, which creates an immunologic response and reduces the rewarding effect of cocaine, may be most effective at preventing relapse to cocaine use. Other treatments, including GABAergic medications, such as topiramate, appear to be advantageous for treatment of comorbid alcohol and cocaine dependent patients, whereas results of studies with disulfiram have been mixed.
Associations between treatment and pharmacogenetics have recently been explored with some of these medications. One such example is with disulfiram, which is believed to inhibit the activity of dopamine β-hydroxylase (DβH), an enzyme that converts dopamine to norepinephrine. Mixed findings with disulfiram may be attributed to differences in genetic variation among individuals. In a trial for disulfiram in patients with comorbid cocaine and opioid dependence, disulfiram treatment was not effective in individuals who had a variant in the DβH gene that resulted in low DβH enzyme levels. Thus genotyping of individuals for this particular DβH polymorphism could determine the success of disulfiram treatment, and exemplifies the importance of pharmacogenetics in treatment of cocaine addiction. In another study of cocaine-dependent patients, the methylene tetrahydrofolate reductase ( MTHFR ) gene was studied as a moderator of disulfiram response, as it is involved with global methylation, which may also be moderated by disulfiram. Although disulfiram treatment was effective at reducing cocaine-positive urine samples across all individuals relative to placebo, a slightly greater efficacy was present in patients who were homozygous or heterozygous for the T allele of the MTHFR gene, suggesting that the MTHFR genotype may be used to tailor pharmacotherapy for cocaine-dependent individuals. Other moderators of the disulfiram response have also been shown for the α1A-adrenoceptor ( ADRA1A ) gene, the ankyrin repeat and kinase domain-containing 1 ( ANKK1 ) gene, and the dopamine receptor D2 ( DRD2 ) gene. Together, these studies suggest that the efficacy of disulfiram treatment may be determined by a number of different polymorphisms in genes involved with decreased stimulation of the α1A-adrenoceptor, global methylation, and dopaminergic function.
It is important to consider that the interaction of genetic variants and treatment response is not the same across different medication types. Of interest, the DβH gene variant showed the opposite effect in a treatment trial for the cocaine vaccine, such that patients with the low-activity DβH gene displayed a reduction in cocaine-positive urine samples with treatment, but not those with the high-activity gene variant. Within the same cocaine vaccine clinical trial, individuals who carried the protective A allele of the κ-opioid receptor (OPRK1) gene, which inhibits dopamine-associated reinforcement, were also significantly less likely to have cocaine-positive urines.
Furthermore, topiramate, an antiepileptic drug that has shown promise for the treatment of alcohol dependence, appears to be another promising new pharmacotherapy for cocaine dependence, as suggested by results from a phase II clinical trial.
Over the past two decades, several pharmacotherapies have been under study for other stimulant use disorders, such as methamphetamine dependence. These clinical trials have shown the most promising results for naltrexone, methylphenidate, bupropion, and mirtazapine. Unfortunately, although there have been some promising findings across these studies, there is not a clear candidate among these medications.
Opiates
Opiate addiction is treated pharmacologically through opiate agonists, antagonists, and partial agonists (for a review see Haile et al. ) The first medication for opioid addiction was methadone, a selective synthetic opioid agonist. Buprenorphine is another synthetic opiate that functions as a partial agonist at mu-opioid receptors and an antagonist at kappa-opioid receptors. Both methadone and buprenorphine are equally effective for maintenance treatment of heroin dependence. Methadone and buprenorphine are metabolized by CYP3A4; however, buprenorphine is metabolized to a much lesser degree than methadone by CYP2D6. Studies have found that Caucasians who lack CYP2D6 function have a poor metabolizer phenotype, which in turn is protective against opiate dependence. Nevertheless, when slow opioid metabolizers go on to develop opioid addiction, they tend to respond well to methadone treatment, whereas opioid-dependent individuals with the CYP2D6 genotype coding for the “ultra-rapid” opioid metabolism are less responsive to the withdrawal relief afforded by methadone maintenance therapy and respond better to buprenorphine, which is not as significantly metabolized by CYP2D6. The case of pharmacogenetics of opioid addiction is an interesting one in that what was learned about the pharmacogenetics of responsiveness to opiates for pain management purposes (described earlier) has informed the clinical literature on the use of opiates for the treatment of opiate addiction and has ultimately led toward the optimization of pharmacotherapy for opioid dependence.
In addition to CYP3A4 and CYP2D6 , a number of other genes have recently been investigated as moderators of the response to methadone and buprenorphine treatment. As an example, the OPRD1 gene, which encodes for the δ-opioid receptor, was examined as a moderator of treatment response in European American and African American opioid-dependent patients assigned to either 24 weeks of methadone or buprenorphine treatment. The authors found that African American CC homozygotes, for the SNP rs678849, were more likely than T allele carriers to have opioid-negative urines if they received methadone treatment. However, of interest, the opposite effect was seen in CC homozygotes who received buprenorphine treatment, as they were more likely than the T allele carriers to have opioid-positive urines. This study exemplifies that not only is genetic variation related to treatment response, but that among these two common treatments for opioid dependence, very different effects may be seen depending on genetic variation of this SNP in the OPRD1 gene. Furthermore, the effect was present only in African American patients, which suggests that genetic variation of certain alleles may not have the same effects across different ancestral backgrounds. Another study of the OPRD1 gene found that genetic variation at other SNPs, namely rs581111 and rs529520, interacted with buprenorphine treatment in females but not in males of European ancestry. Thus sex may be another important factor in the selection of pharmacotherapies for opioid dependence.
Furthermore, the brain-derived neurotrophic factor (BDNF) has been examined for SNPs that may moderate methadone maintenance treatment because BDNF is involved with the neural plasticity that may underlie opioid-induced plasticity. The authors found a haplotype consisting of six SNPs for which genetic variation was related to a greater likelihood of being a poor responder to methadone treatment. OPRD1 and BDNF are just a few of many genes that have been recently explored in pharmacogenetic studies of opioid dependence, but these preliminary findings have yet to be replicated in future studies.
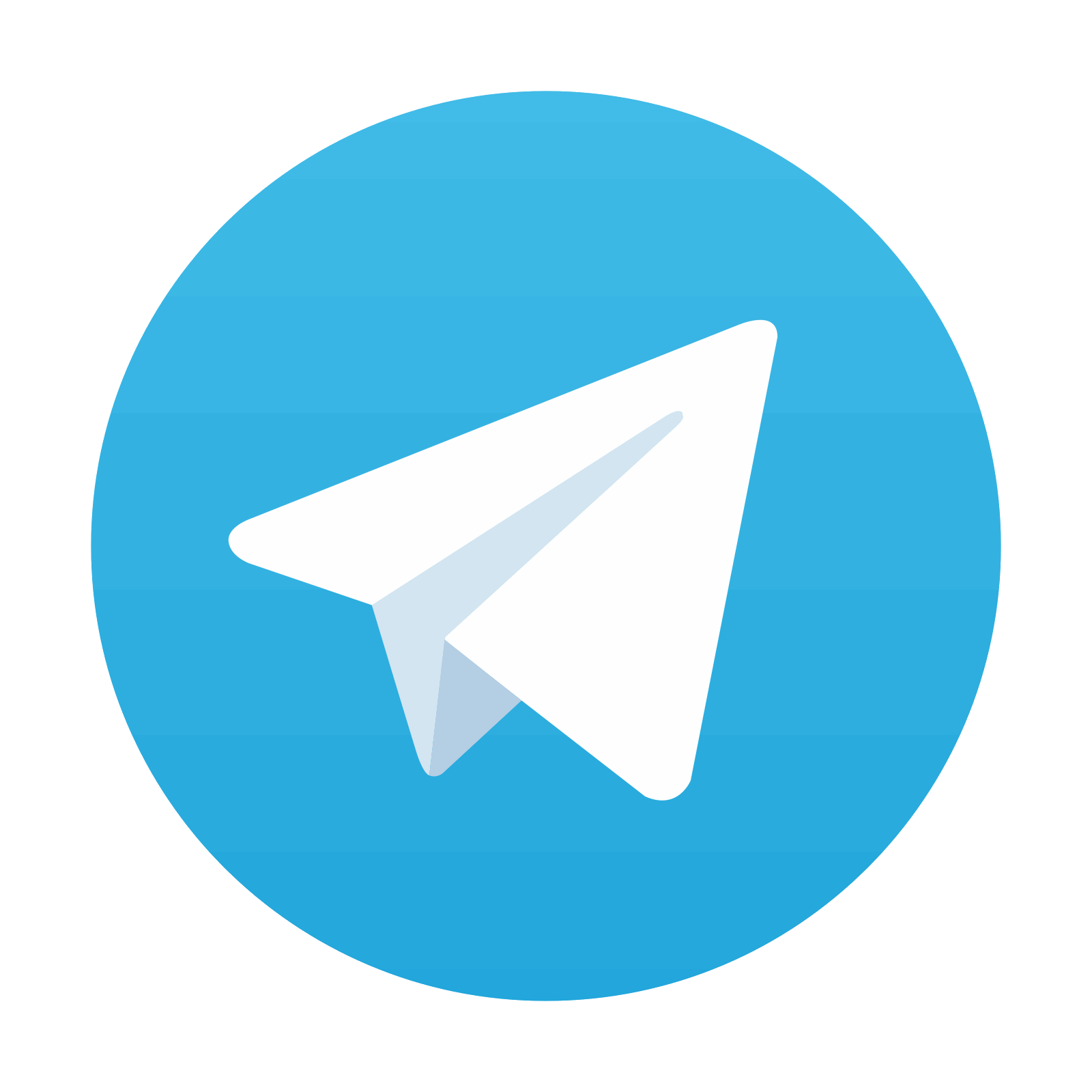
Stay updated, free articles. Join our Telegram channel

Full access? Get Clinical Tree
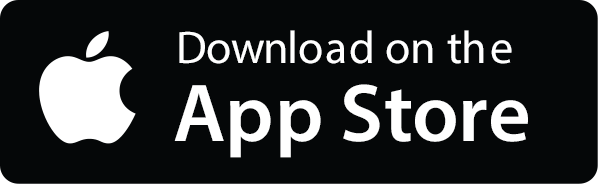
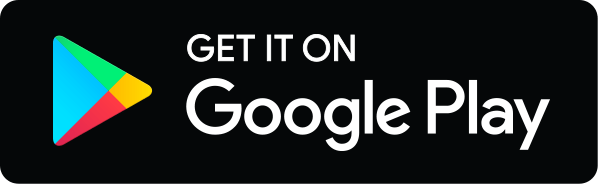