Study
Method
Subtype
Normal
3p
9p
Chr. 22
Other
Gibas 1986
Cytogenetics
Epithelioid
1/8 (13 %)
4/8 (50 %)
3/8 (38 %)a
Loss chr. 22
2/8 (25 %)
Translocation 22q 1/8 (13 %)
Biphasic
0/4 (0 %)
3/4 (75 %)
1/4 (25 %)
Translocation 22q
2/4 (50 %)
All subtypes combined
1/12 (8 %)
7/12 (58 %)
4/12 (33 %)
5/12 (42 %)
Chr 1p, Chr 6, Chr 11, Chr 17
Popescu 1998
Cytogenetics
Epithelioid
0/1
1/1
Biphasic
1/1
0/1
All subtypes combined
1/2 (50 %)
1/2 (50 %)
Hagemeijer 1990
Cytogenetics
Not specified
9/39 (23 %)
Loss or translocations
27/39 (69 %)
Loss
24/39 (62 %)
Loss chr. 22 15/39 (38.4)
Loss 4 q or p
Gain Chr. 5, 20
Taguchi 1993
Cytogenetics
Not specified
3/23 (13 %)b
13/23 (57 %)
Loss Chr. 9
2/23 (9 %)
Loss9p
14/23 (61 %)
Loss chr. 22
13/23 (57 %)
Chr. 1p and 1q rearrangements
6q, loss of chr. 14, 16, 18
Bjorkqvist 1997
CGH
Epithelioid
2/8 (25 %)
0/8 (0 %)
4/8 (50 %)
Loss 22q
1/8 (13 %)
Sarcomatoid
1/1 (100 %)
0/1 (0 %)
0/1 (0 %)
0/1 (0 %)
Biphasic
9/18 (50 %)
0/18 (0 %)
5/18 (28 %)
Loss 22q
2/18 (11 %)
All subtypes combined
12/27 (44 %)
0/27 (0 %)
9/27 (33 %)
3/27 (11 %)
Loss 4q, 6q, 13, 14q
Gain 1q
Krismann 2002
CGH
Epithelioid
3/27 (11 %)
Loss chr 3
1/27 (4 %)
Loss 3p
9/27 (33 %)
8/27 (30 %)
Loss chr. 22
6/27 (22 %)
Loss 22q
5/27 (19 %)
Sarcomatoid
5/28 (18 %)
2/28 (7 %)
Loss Chr 9
1/28 (4 %)
Loss 9p21
8/28 (29 %)
Loss chr. 22
2/28 (7 %)
Loss 22q
3/28 (11 %)
Biphasic
3/22 (14 %)
1/22 (5 %)
5/22 (23 %)
Loss chr. 22
1/22 (5 %)
Loss 22q
1/22 (5 %)
All subtypes combined
11/77 (14 %)
13/77 (17 %)
22/77 (29 %)
18/77 (23 %)
Loss 1p, 3p, 4q, 4p, 6q, 10p, 17p, 13q, 14q
Gains 1q, 7p, 8q, 15q
One of the most common chromosomal abnormalities described in 29–62 % of cases is loss of a region of the short arm of chromosome 9 (9p), most frequently involving bands 9p21–p22 [2, 4, 5, 7, 8] Chromosome 22 or 22q is reported to be lost in 11–57 % of cases [2−7]. Abnormalities in the short arm of chromosome 3, in particular, around band 3p21, have been reported in 0–69 % of mesothelioma cases [2−7]. These studies have also reported other “nonrandom” chromosomal losses and gains. Subsequent to these studies, various research groups have identified some of the genes present in some of these chromosomal regions and determined the functions of the proteins encoded by these genes.
9p21 Deletion
The most common chromosomal abnormality reported in malignant mesothelioma lies in the short arm of chromosome 9, in particular homozygous deletion of 9p21-p22 [2−8]. This region is lost in many tumor types including melanoma, non-small cell lung carcinomas, gliomas, osteosarcomas, and other tumors [9−13]. The 9p21-p22 region contains the gene for cyclin-dependent kinase inhibitor (CDK) 2A/alternative reading frame gene (CDKN2A/ARF), an adjacent related gene CDKN2B, and the methylthioadenosine phosphorylase (MTAP) gene [9,14,15].
Since the initial report, studies have described CDKN2A deletions in 49–80 % of malignant mesotheliomas (non-subtyped), 56–77 % of epithelioid mesotheliomas, 100 % sarcomatoid mesotheliomas, and 84–100 % of biphasic mesotheliomas (Table 6.2) [16−22]. In addition mutations in CDKN2A, which lead to loss of function and hypermethylation of 5’ CpG islands leading to downregulation of expression of proteins encoded by CDKN2A, have also been described [23]. Because of the frequency of deletion of CDKN2A, identification of CDKN2A deletions by fluorescence in situ hybridization has been suggested as a marker for separation of benign mesothelial proliferations and malignant mesotheliomas, particularly in cytology specimens [19,24]. CDKN2A may also have prognostic significance; patients that have CDKN2A deletions fare worse than those for whom deletions are absent [23].
Table 6.2
Frequency of CDK2N2a homozygous deletion in mesothelioma, polymerase chain reaction (PCR), Southern blotting, fluorescence in situ hybridization (FISH), and/or DNA sequencing observed in studies from various authors who are listed in the first column
Method | Epithelioid | Biphasic | Sarcomatoid | Biphasic or sarcomatoid | Total | |
---|---|---|---|---|---|---|
Cheng 1994 | PCR and Southern Blotting | 5/23 (22 %) | ||||
Illei 2003 | FISH | 49/71 (69 %) | 16/19 (84 %) | 5/5 (100 %) | 70/95 (74 %) | |
Takeda 2010 | FISH | 24/28 (86 %) | 6/7 (86 %) | 3/3 (100 %) | 33/38 (87 %) | |
Bott 2011 | Sequencing | 20/30 (67 %) | 6/6 (100 %) | 3/3 (100 %) | 29/39 (74 %) | |
Takeda 2012 | FISH | 23/30 (70 %) | 12/12 (100 %) | 35/42 (83 %) | ||
Wu 2013 | FISH | 10/18 (56 %) | 7/8 (87.5 %) | 22/22 (100 %) | 35/42 (83 %) |
The CDKN2A/ARF gene encodes two proteins p16INK4a and p14ARF.25−29 P16INK4a is encoded by exons 1α, 2, and 3, whereas p14ARF is encoded by exons 1β, 2, and 3, as well as an alternative reading frame for exon 2 [25−29]. Thus, p16INK4a and p14ARF do not share an amino acid sequence and have distinct binding partners and functional pathways in the cell. However, both p16INK4a and p14ARF are thought to function as tumor suppressors [25−29].
The protein p16INK4a controls the cell cycle via interaction with the cyclin-dependent kinase 4/Cyclin D/pRB pathway. P16INK4a binds to CDK4 and is an inhibitor of CDK4 activity [9, 14, 26]. CDK4 activity leads to activation of cyclins D1, D2, and D3 which, in turn, phosphorylate pRB [30, 31] Phosphorylation of pRB releases the transcription factor E2F and, thus, allows transcriptions of numerous genes required for cellular proliferation [30, 31]. Thus, by inhibiting CDK4 activity, p16INK4a slows cell-cycle progression (Fig. 6.1a), and loss of p16INK4a would lead to overly rapid growth for the cells and neoplastic transformation (Fig. 6.1b) [32].


Fig. 6.1
Schematic of p16INK4a function. a No deletion of 16INK4a: P16INK4a (p16) binds to cyclin-dependent kinase 4 or 6 (CDK4/6) and CyclinD and prevents phosphorylation of the retinoblastoma protein (RB). RB remains bound to E2F and transcriptional activation does not occur, b Homozygous deletion of 16INK4a: Cyclin-dependent kinase 4 or 6 (CDK4/6) binds CyclinD and phosphorylates the retinoblastoma protein (RB). Phosphorylated-RB releases E2F. E2F-mediated transcriptional activation proceeds and subsequent cell replication can occur
P14ARF likely has multiple functions in the cell, but one major function is regulation of the p53 pathway. Mutations in p53 are uncommon in mesothelioma [20, 33−35]. P14ARF has been shown to interact with and block the activity of mouse double minute 2 (MDM2) and ARF-binding protein 1/Mcl1-ubiquitin ligase E3 (ARF-BP1/Mule; Fig. 6.2a) [36−38]. Both MDM2 and ARF-BP1/MULE are ubiquitin ligases that can ubiquitinate multiple proteins, including p53, and lead to proteasome-mediated degradation of these ubiquitinated proteins [36−38]. P53 is thought to stimulate the expression of another cyclin-dependent kinase inhibitor, CDKN1A/p21 [36−38]. Thus, loss of p14ARF is thought to also allow overly rapid growth of cells and neoplastic transformation (Fig. 6.2b) [36−38].


Fig. 6.2
Schematic of p14ARF function. a No deletion of p14 ARF : P14 ARF (p14) binds to mouse double minute 2 (MDM2) and prevents MDM2 from ubiquitinating p53. P53 remains active and can prevent cell-cycle progression or initiate apoptosis, b Homozygous deletion of p14 ARF : MDM2 from ubiquitinates p53. Then, ubiquitinated p53 is degraded by the proteasome. Subsequently, the cell cycle can progress even in the presence of DNA damage and increase the risk of development additional mutations
Subsequently, an adjacent related gene CDKN2B which encodes p15INK4B/MTS2 was also identified in this region. About 72 % of mesotheliomas show co-deletion of p15INK4B/MTS2 and p16INK4a [39]. P15INK4B/MTS2 is thought to have a similar function as p16INK4a and is an inhibitor of CDK4 and CDK6; thus, p15INK4B is considered a tumor suppressor, which can supplement the activity of p16INK4a in mouse models [40].
MTAP is a gene 100 kilobases telomeric to the CDKN2A gene and encodes MTAP. By fluorescence in situ hybridization, MTAP deletions were identified in 67 % of total pleural mesothelioma cases, 63 % of epithelioid mesotheliomas, 79 % of biphasic mesotheliomas, and 80 % of sarcomatoid mesotheliomas [18]. All of the cases with MTAP deletion also showed CDKN2A deletion, but about 10 % of cases with CDKN2A deletion did not have MTAP deletion [18]. The MTAP protein converts methylthioadenosine to adenine, which is required for AMP synthesis, and methylthioribose 1-phosphate, which is required for methionine synthesis [18].
Chromosome 22
By cytogenetics and comparative genomic hybridization, the most frequent chromosome lost in mesothelioma, occurring in up to 57 % of cases, is chromosome 22. In addition, or alternatively, sequences within the long arm of chromosome 22 (22q) may be deleted (Table 6.1) [2, 4−7]. One of the genes present on chromosome 22q12 is NF2 which is the gene identified in patients with neurofibromatosis type 2. Other genes present on chromosome 22 which also have been reported to be deleted include SMARCB1, CHEK2, and DMC1 [20].
By reverse transcription polymerase chain reaction (RT-PCR) and sequencing, or by fluorescence in situ hybridization, 38–72 % of cases of mesothelioma have NF2 homozygous deletion (both copies of the gene deleted), heterozygous deletion (one copy of the gene deleted), truncations, alternative splice variants leading to deletions of one or more exons, and point mutations or small insertions or deletion leading to missense or nonsense mutations (Table 6.3) [20, 21, 35, 41, 42]. Some reports have indicated that mutations in NF2 are more common in epithelioid mesotheliomas, whereas other reports have shown increased frequency of NF2 mutations in biphasic or sarcomatoid mesotheliomas (Table 6.3) [20, 21, 35, 41, 42]. In addition, there is evidence that even in cases with full-length protein expression, the protein is phosphorylated and inactivated [42].
Table 6.3
Frequency of NF2 deletion or mutation in mesothelioma by reverse transcriptase polymerase chain reaction (RT-PCR), DNA sequencing, or fluorescence in situ hybridization (FISH) observed in studies from various authors who are listed in the first column
Method | Epithelioid | Biphasic | Sarcomatoid | Biphasic or sarcomatoid | Total | |
---|---|---|---|---|---|---|
Bianchi 1995 | RT-PCR and sequencing | Mutations 6/15 (40 %) | ||||
Thurneysen 2009 | RT-PCR and sequencing | Mutations and splicing variants leading to truncation 13/26 (50 %) | Mutations and splicing variants leading to truncation 4/18 (22 %) | Mutations and splicing variants leading to truncation 17/44 (39 %) | ||
Bott 2011 | CGH, FISH and sequencing | Heterozygous deletion 27/53 (51 %)Mutation 4/53 (7.5 %)Heterozygous deletion and mutation 7/53 (13 %) | ||||
Takeda 2012 | FISH | Homozygous deletion 10/30 (33 %) | Homozygous deletion 6/12 (50 %) | Homozygous deletion 16/42 (38 %) | ||
Andujar 2013 | Sequencing | Mutations including exon deletions and/ or point mutations 13/34 (38.2 %) |
NF2 encodes a tumor suppressor protein Merlin (moesin–ezrin–radixin-like protein) which is also known as Schwannomin or Neurofibromin 2. It was initially identified as the gene involved in patients with neurofibromatosis type 2, which is characterized by development of bilateral vestibular schwannomas, gliomas, meningiomas, schwannomas of other central sites, and juvenile cataract formation [43, 44]. The NF2 gene encodes at least two main isoforms of Merlin that are found in normal human cells from alternative splicing of the transcripts. Only isoform I is thought to act as a tumor suppressor gene. The function of merlin is complex and the subject of much ongoing research. Merlin is thought to play a role in upregulation and downregulation of multiple signal transduction pathways for cell proliferation. Merlin had been found to interact with at least 34 different proteins [45, 46].
Merlin activity is regulated by phosphorylation. Phosphorylation of serine at amino acid 518 (Ser518) in the C-terminal domain can occur through multiple pathways, including the RAC1 and p21-activated kinase (PAK) pathway as well as by cyclic adenosine monophosphate-dependent protein kinase A (PKA) [46−48]. Phosphorylation of Ser518 leads to unfolding of the N-terminal and C-terminal domains or an “open” conformation. The “open” conformation of the protein cannot interact with many of the proteins to which the “closed” confirmation can bind. Phosphorylation of Ser518 also causes change in localization of the protein from the cell membrane to the cytoplasm [46−48]. The “closed” form of merlin also may function as an inhibitor of the RAC–PAK signaling cascade and prevent PAK-induced cyclin D1 expression [46−48].
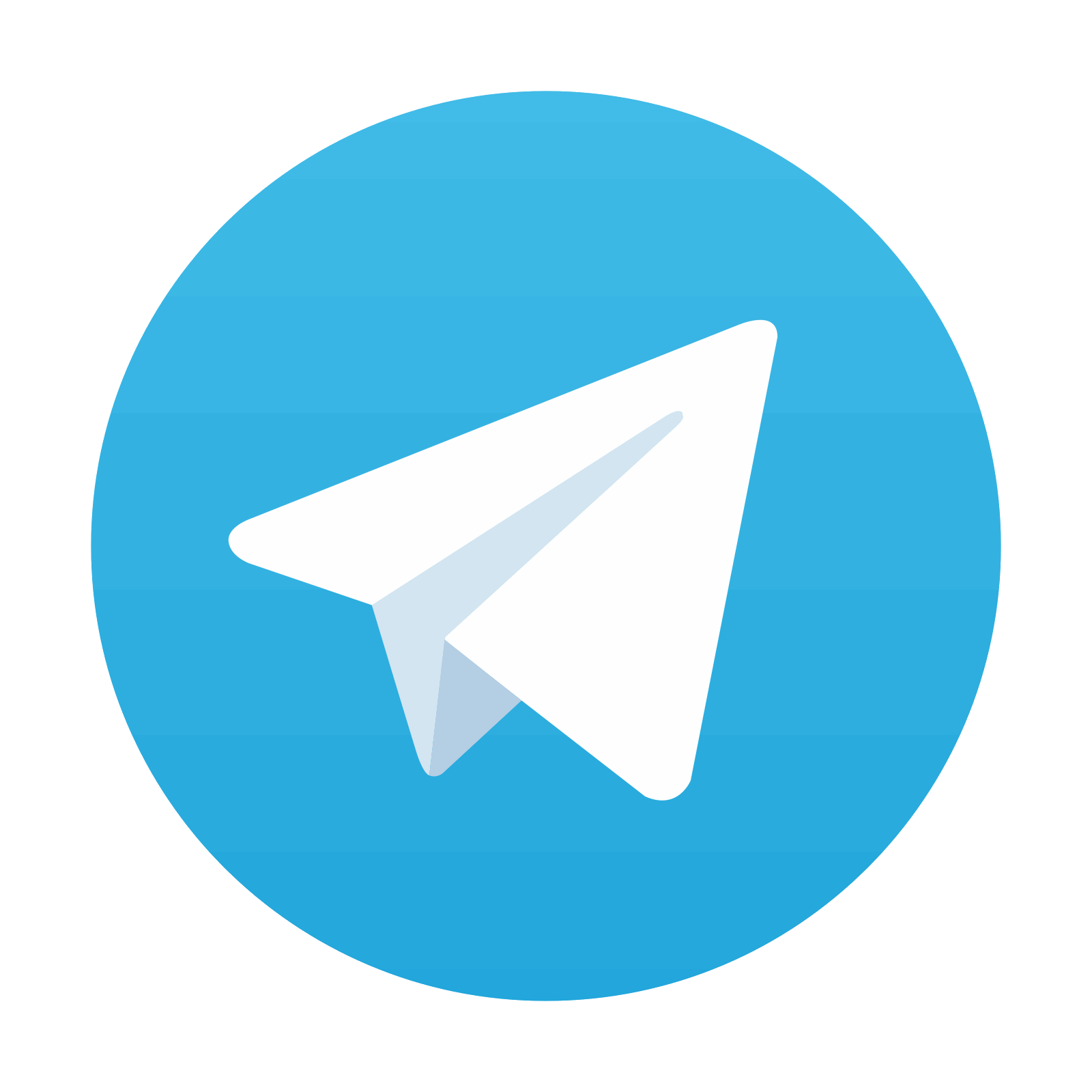
Stay updated, free articles. Join our Telegram channel

Full access? Get Clinical Tree
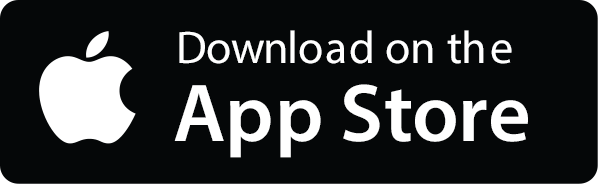
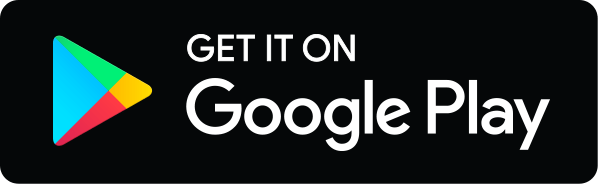